- 1Independent Consultant, Tegucigalpa, Honduras
- 2Instituto de Hidráulica e Hidrología, Universidad Mayor de San Andrés, La Paz, Bolivia
- 3Institut des géosciences de l'environnement (IGE) Univ. Grenoble Alpes, CNRS, IRD, Grenoble, France
- 4Laboratoire de Météorologie Dynamique, LMD-IPSL, Sorbonne Université, CNRS, Paris, France
- 5Departamento de Geografía, Facultad de Humanidades, Universidad del Valle, Cali, Colombia
- 6LEGOS, Université de Toulouse, IRD, CNES, CNRS, UPS, Toulouse, France
- 7Subdirección de Ciencias de la Atmósfera e Hidrósfera, Instituto Geofísico del Perú, Lima, Peru
- 8Departamento de Ordenamiento Territorial y Construcción, Universidad Nacional Agraria La Molina, Lima, Peru
- 9Unidad de Recursos Hídricos, Instituto Hondureño de Ciencias de la Tierra, Universidad Nacional Autónoma de Honduras, Ciudad Universitaria, Tegucigalpa, Honduras
- 10Departamento de Geociências, Universidade Federal do Amazonas, Manaus, Brazil
The Madeira River rises in the Andes, draining the southwestern Amazon basin and contributing up to 50% of the Amazon River sediment load. The Porto Velho station monitors the Upper Madeira basin and is located just downstream of the Jirau and Santo Antonio hydropower dams. At this station, decreasing trend (p < 0.10) of the surface suspended sediment concentration (SSSC) has been documented during the sediment peak season (December to February) for the 2003–2017 period. This study aims to evaluate the role of the rainfall variability on this documented decreasing trend. For this purpose, we applied correlation and trend analysis in water discharge, SSSC and rainfall time series over the main tributaries of the Upper Madeira basin. The decline of SSSC in December is attributed to the reduction of rainfall in the Madre de Dios sub-basin from the start of the rainy season in October. However, the SSSC negative trend (p < 0.10) in January and February is associated with a shift in the magnitude of rainfall during these months in the Andean region after 2008, and the dilution associated with base flow. These results reveal that the decline of SSSC in the Madeira River should not be evaluated just on the basis of the data downstream from the dams, but also of the processes upstream in the Andean part of the basin. In a context of drastic anthropogenic climate and environmental changes, understanding the combined influence of regional hydroclimate variability and human actions on erosion and sediment transport remains a critical issue for the conservation of the Amazon-Andes system.
Introduction
The Andes leave an indelible imprint on the geochemistry and ecology of the Amazon River system, the largest in the world. The Madeira River, the second largest tributary of the Amazon, drains the southwestern Amazon basin, which has a great geographical, biological, and climatic diversity (Molina-Carpio et al., 2017). At its confluence with the Amazon, the Madeira River delivers 26,580 m3·s−1 and provides 426 Mt·year−1 of suspended sediment load (SSL; nearly 50% of the Amazon's SSL at Óbidos for the 2002–2011 period; Vauchel et al., 2017). At the Porto Velho station (Figure 1) a mean annual discharge of 18,550 m3·s−1 and SSL of 441 Mt·year−1 were recorded for the 2002–2011 period (Vauchel et al., 2017).
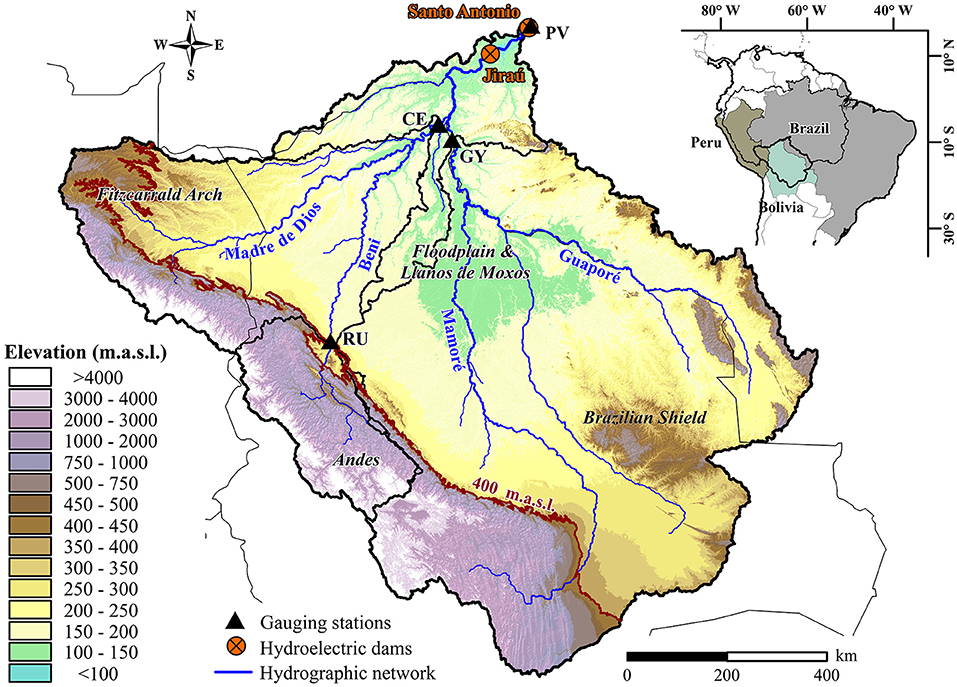
Figure 1. Upper Madeira basin (1 × 106 km2) at Porto Velho (PV) outlet gauge station. Cachuela Esperanza (CE) on the Beni River, Rurrenabaque (RU) on the Andean Beni River, and Guayaramerin (GY) on the Mamoré River. Sources: Elevation data from STRM (Farr et al., 2007).
The complexity of the Madeira River system is evident from the presence of four geomorphological units (Figure 1): The Andes, the Fitzcarrald Arch, the Llanos de Moxos floodplain, and the Brazilian shield. Steep terrain and young lithologies make the Andes the main source of sediments and solutes to the lower reaches of the Madeira River (McClain and Naiman, 2008). The average sediment production of the Andean stretch of the Upper Madeira was estimated at 640 Mt·year−1 (2002–2011; Vauchel et al., 2017), implying that over 30% of the Andean sediment is deposited in the Andean piedmont and the Llanos de Moxos floodplain. Depending on which geomorphological unit the tributaries are born, contrasting characteristics are observed: white-water tributaries (the Beni, Mamoré and Madre de Dios rivers that rise in the Andes), the clear-water Guaporé River that comes from the Brazilian shield and several black-water lowland tributaries. In fact, the large differences in SSL between the Mamoré and Beni rivers (Figures 2B,D) are mainly explained by the different geomorphology of their watersheds. Although both rivers rise in the Andes, no sedimentation process is observed in the Beni River floodplain, resulting in the Andean Beni River at Rurrenabaque (Figure 1) to provide 45% of the Madeira SSL with a watershed area of only 7% of the Upper Madeira basin (Vauchel et al., 2017). Hence, the Beni River provides nearly 80% of the SSL at Porto Velho: 47% from the Beni River itself and 32% from its main tributary, the Madre de Dios River (Vauchel et al., 2017). On the other hand, significant sedimentation occurs in the Andean piedmont and the entry to the floodplain in the Mamoré River basin. This is explained by Vauchel et al. (2017) by the Mamoré River slope break at the piedmont, that reduces the energy available in the river channel to transport sediment. Furthermore, the Guaporé River, the main tributary of the Mamoré, has most of its basin on the geomorphic stable Brazilian shield, resulting in very low sediment yield and denudation rate (0.02 mm·year−1; Guyot et al., 2007; Withmann, 2008). As a consequence, the Mamoré and Guaporé rivers provide only 15% of the Madeira SSL at Porto Velho (Vauchel et al., 2017).
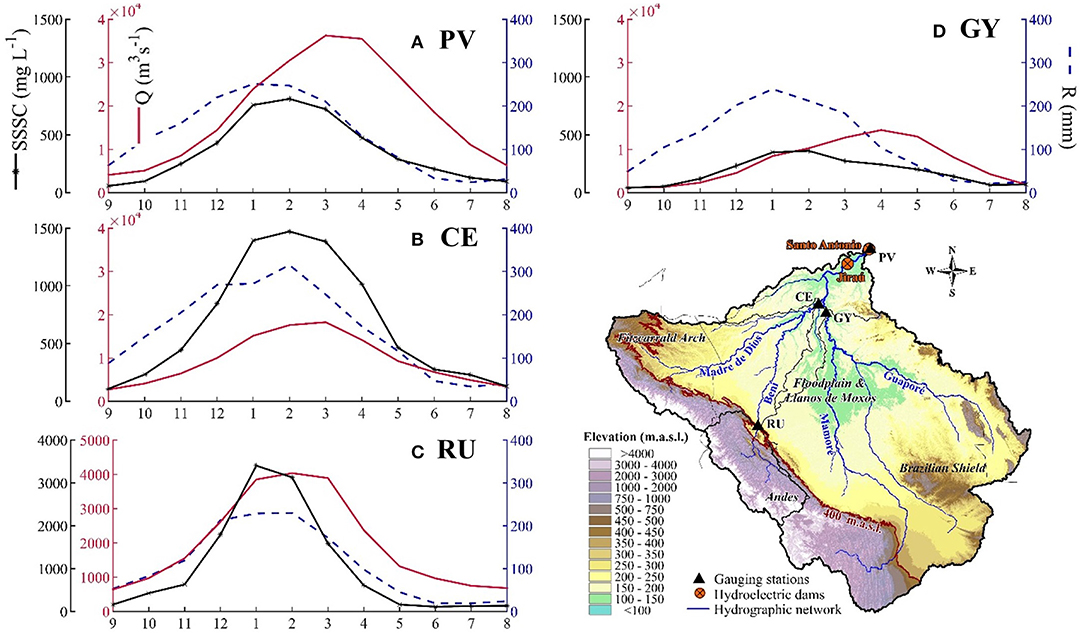
Figure 2. Annual cycle (2003–2017) of rainfall (R, blue dash line), water discharge (Q, red line), and surface suspended sediment concentrations (SSSC, black asterisk line): (A) at Porto Velho (PV) on the Madeira River; (B) at Cachuela Esperanza (CE) on the Beni River; (C) at Rurrenabaque (RU) on the Andean Beni River; and (D) at Guayaramerin (GY) on the Mamoré River. Sources: for the rainfall: CHIRPS.v2 (Funk et al., 2015), Q and SSSC adapted from Ayes et al. (2019a) and Ayes et al. (2019b) with data from SO-HYBAM, ANA, and SENAMHI. Please note that the two left y-axis differ in (C) because of the magnitude, and the x-axis is the hydrological year, from September (9) to August (8).
The tropical climate of the Madeira basin, and especially the intensity, magnitude, and seasonality of the rainfall in sediment-source regions as the Andes greatly influences the sediment dynamics at different time scales (e.g., Pepin et al., 2013). A significant rainfall spatial variability has been identified in the Upper Madeira basin. From north to south mean annual rainfall is 2600 mm for the Madre de Dios sub-basin, 1800 mm for the Beni sub-basin and 1600 mm for the Mamoré-Guaporé (Molina-Carpio et al., 2017). High rainfall in the Madre de Dios sub-basin is explained by its northernmost position and the presence of an important “rainfall hotspot” in the Sub-Andes and piedmont, where up to 6600 mm·year−1 is recorded (Espinoza et al., 2015). On a seasonal timescale, the entire Upper Madeira basin is characterized by a south tropical rainfall regime (Molina-Carpio et al., 2017), with a marked wet season during the austral summer, related to the mature phase of the South American Monsoon System (Berbery and Collini, 2000). On average, over half of the annual rainfall is recorded between December and March, with January and February being the wettest months (Espinoza et al., 2019a).
The Madeira River intra-annual discharge seasonality at Porto Velho, which peaks between March and April (Figure 2A), is the result of the rainfall and hydrological regimes of the main tributaries. Figure 2B shows that the mean annual discharge peak of the Beni River at Cachuela Esperanza comes at about the end of February and the beginning of March, while the peak at the station of Guayaramerin on the Mamoré River usually occurs later in mid-April (Figure 2D). Surface suspended sediment concentration (SSSC) at Porto Velho starts to increase in October with the beginning of the rainy season. It peaks between January and March (Figure 2A), in response to the Beni River SSSC annual cycle (Figures 2B,C).
Information on the Madeira River sediment dynamics is even more essential as the consequences of human activities (e.g., deforestation, sedimentation induced by dams, climate change) on the wellbeing of the riverine and indigenous population and ecosystems become more evident. The Andean-Amazon connectivity has been compromised for years. Nobre et al. (2016) suggest that deforestation over the southwestern Amazon could result in savannization in the upcoming decades. Deforestation in the Upper Madeira might increase soil erosion and sediment production as observed in the Andes of Colombia (Restrepo et al., 2015) and in Ecuador (Molina et al., 2008). Additionally, land use changes can modify the local hydroclimatic conditions as observed in the Upper Madeira Basin (Gutierrez-Cori et al., 2021). There is no doubt that the effects of land use changes need to be evaluated over a large timeframe. Changes in the spatio-temporal rainfall variability over the Andes-Amazon transition region as reported by Espinoza et al. (2019a) and Segura et al. (2020) has been related to large scale atmospheric conditions and could modify the sediment dynamics as well over different temporal scales. Indeed, Armijos et al. (2020) found that the temporal rainfall variability is well-related (R2 = 0.89) to the fine suspended sediment concentration (FSC; >0.45 μm and <63 μm) at Porto Velho, while the coarse sediment (>63 μm) is better related to the water discharge.
Hydropower facilities already in operation or been planned in the Madeira basin (Anderson et al., 2018) can significantly reduce the SSL reaching the Amazon River. Santo Antonio and Jirau, two hydropower dams in the river stretch upstream from Porto Velho (Figure 1) and completed in 2011 and 2012, respectively, have already caused serious social and environmental impacts (Almeida et al., 2019, 2020; Van Damme et al., 2019; Lima et al., 2020). These impacts had a profound effect on downstream and upstream riverine areas in Brazil and Bolivia and the communities who depend on the river resources. The dams were also credited by Latrubesse et al. (2017) to have caused an ~20% decrease in the mean surface suspended sediment concentration (SSSC) of the Madeira River at Porto Velho, located just 7 km downstream of Santo Antonio dam. Li et al. (2020) also detected a negative trend of SSSC for the 1995–2017 period at Fazenda Vista Alegre, the station furthest downstream on the Madeira River before its confluence with the Amazon River. In addition, Ayes et al. (2019a) found an ever-greater fall (30%) in FSC at Porto Velho in the post-dam period. However, a concomitant 36% decline in the FSC observed at Cachuela Esperanza, located upstream from the dams on the Beni River, which provides about 80% of the Madeira SSL, suggests that most of the fall in FSC could not be attributed to the dams. Indeed, the study of Vauchel (2014) demonstrated that the dams do not generate a backwater effect at Cachuela Esperanza. On the other side the already observed sedimentation of coarser particles (mostly fine and medium sands) in the Santo Antonio and Jirau reservoirs has been directly associated with the river impounding by the dams (International Hydropower Association (IHA), 2017).
While negative trends from 2003 to 2017 and a downward break in 2010 were detected at Porto Velho for the annual and December-February (DJF) series of FSC, trends were not detected for the discharge series during this period (Ayes et al., 2019a). For the DJF sediment peak season at Cachuela Esperanza, the study identified a FSC negative trend and a significant break in 2010. No FSC trend or break were found for the Mamoré River at Guayaramerin for the same period in the previous study. The causes of the FSC downward break just before the dams were completed and the negative trend during DJF for the 2003–2017 period in the Beni River, merit being identified and explained.
Given the importance the Madeira River sediment dynamics has on the geochemistry and ecology of the Amazon River system and with a view to improve the knowledge of the different processes (natural and anthropogenic) that are occurring within the Upper Madeira River basin, this study aims to investigate the role of the rainfall variability on the SSSC downward break in 2010 and declining trend identified for the 2003–2017 period at the Porto Velho station.
Materials and Methods
Water Discharge and Sediment Data
The water discharge (Q) and surface suspended sediment concentration (SSSC) data are generated by the National Water Agency of Brazil (ANA in Portuguese) at Porto Velho and the National Meteorological Service of Bolivia (SENAMHI in Spanish) at Rurrenabaque and Cachuela Esperanza, with the collaboration of the SO-HYBAM Observatory. This information is available online at a daily time-step for Porto Velho from 1967 to 2019 for Q and from 1995 to 2017 for SSSC, and for Rurrenabaque from 1967 to 2016 for Q and 2003–2017 for SSSC. Additionally, November to February SSSC data from Cachuela Esperanza at the Beni River outlet for the 2003–2017 period was used. This information was taken from Ayes et al. (2019a) and was initially provided by SENAMHI-Bolivia and SO-HYBAM. In order to compare the results of this study with those of previous ones (i.e., Ayes et al., 2019a,b), we evaluated a period from 2003 to 2017 at Porto Velho and Rurrenabaque. The hydrological year from September (yeart−1) to August (yeart0) was used for the analysis. Water discharge is regularly measured using an Acoustic Doppler Current Profiler to establish water level-discharge rating curves.
Suspended sediment samples are collected three times per month at the surface in the lowlands and up to six times per month at the piedmont and Andean stations, following the protocol of the SO-HYBAM Observatory, and then interpolated at a daily time-step by the SO-HYBAM. More information on the sediment concentration measurement procedure and data quality can be found in Armijos et al. (2017), Vauchel et al. (2017) and the observatory webpage (https://hybam.obs-mip.fr/). It should be noticed that the surface sample is not representative of the sediment concentration on the entire cross-section for the sediment time-series. A rating curve can be established between these concentrations, but the analysis was carried out solely on the concentrations at the surface to reduce uncertainties. SSSC was used to evaluate the possible causes for trends and the 2010 break at Porto Velho, and not the fine suspended sediment concentration (FSC) in the water column as in Ayes et al. (2019a). Nevertheless, as Ayes et al. (2019a) estimated FSC by multiplying SSSC by a constant value following Armijos et al. (2020), clearly both series are fully comparable regarding time variability. Moreover, the relationship of SSSC and FSC has not been evaluated at the Rurrenabaque station, which was also used in this study.
Missing SSSC data at Porto Velho (February−2016 to March−2016) were estimated based on the method of Espinoza-Villar et al. (2013) which used the reflectance information from the Moderate Resolution Imaging Spectroradiometer (MODIS) sensors on board the Aqua and Terra satellites, previously validated for the 2000–2011 period with in-situ measurements in the Madeira River. Likewise, MODIS reflectance has been calibrated with observed SSSC for several Amazon Rivers (Martinez et al., 2009), the Solimões River (Espinoza-Villar et al., 2012), the Purus River (Dos Santos et al., 2018), and the confluence of the Solimões and Negro rivers (Marinho et al., 2018) among others. Estimated SSSC time series derived from MODIS and measured SSSC are available at the SO-HYBAM webpage.
Rainfall Data
Rainfall was estimated from the satellite-based precipitation product CHIRPS.v2 (Funk et al., 2015). This satellite dataset is freely available at the Climate Hazards Center website (https://www.chc.ucsb.edu/data) with two spatial resolutions of 0.05° and 0.25° and a 1-day of temporal resolution. The product has been used previously for the Amazon Basin (e.g., Espinoza et al., 2019b and Cavalcante et al., 2020) and compared with observed datasets for the Bolivian Altiplano (Satgé et al., 2019) and the entire Amazon Basin (Espinoza et al., 2019b; Paca et al., 2020). For this study the mean sub-basins rainfall at 0.05° resolution was calculated and accumulated to the monthly time-step for the October-February season from 1981 to 2017.
CHIRPS data exhibit non-negligible bias, particularly in the humid-Andes regions like the upper Madre de Dios and upper Beni River at Rurrenabaque sub-basins, where CHIRPS data were found to underestimate precipitation (Noriega, 2018). Thus, an observed homogenized Bolivian dataset by Hunziker et al. (2017) for the 2003-2013 period was used to evaluate and support the precipitation changes identified with CHIRPS in the Andes. The 20 ground stations are listed in Table 1 and mapped in Figure 5 of the results section. A most extensive use of ground-based observations in this study was prevented by the sparsity of the rain gauge network, particularly in the Madre de Dios sub-basin and lowlands.
Correlation and Time Series Analysis
Possible relationships between the data were analyzed through ranked Kendall (τ) correlation. While to investigate the SSSC downward breaks at Cachuela Esperanza and Porto Velho for the 2003–2017 period (Ayes et al., 2019a), the following nonparametric statistical tests were applied to the rainfall, discharge, and SSSC monthly time-series: (1) the Pettitt (Pettitt, 1979) and Lanzante tests (Lanzante, 1996) to seek breakpoints in the time-series, and (2) the Mann-Whitney test (w; Mann and Whitney, 1947) to investigate changes in the distribution if a break is found. A break in a time-series was accepted when the two tests detected it in the same year. Whereas, the Mann-Kendall test (MKτ; Kendall, 1938; Mann, 1945) and Sen slope (Sen 1968) were used to evaluate temporal trends. Outcomes with 90 and 95% confidence intervals are shown in the results section.
Onset of the Rainy Season
To determine the onset of the rainy season, CHIRPS.v2 daily data is first spatially averaged over the Upper Madeira basin and the four main tributaries sub-basins. To reduce the noise and define the onset of the wet season a pentad consecutive approach is used. A pentad is defined as the mean daily rainfall in five consecutive days. The onset is defined by a threshold (historic average daily rainfall over the Upper Madeira basin) persistent in time (six consecutive pentads out of seven) above the threshold. This methodology has been previously used in several studies in order to identify the length of the rainy season in the Southern Amazon basin (e.g., Fu et al., 2013; Arias et al., 2015; Gutierrez-Cori et al., 2021).
Results
Figure 3 shows that the mean SSSC (590 mg·L−1 for the DJF season) at Porto Velho from 2010 to 2017 was lower than during the 2003–2009 period (SSSC = 750 mg·L−1). Following the SSSC changes detected in the Beni River at Cachuela Esperanza (Ayes et al., 2019a) and as nearly all of the fine suspended sediment transported by the Beni River comes from the Andean part of its basin (see Introduction), the temporal relationship between Rurrenabaque and Cachuela Esperanza SSSC series was evaluated at a monthly time-step from November to February. From 2003 to 2017 a significant positive correlation between Rurrenabaque and Cachuela Esperanza SSSC series is found (τ = 0.43, p < 0.001), which even improves when the extreme 2014–flood event is removed (τ = 0.48, p < 0.001). Both the Rurrenabaque sediment contribution and the significant correlation between Rurrenabaque and Cachuela Esperanza SSSC indicate that the temporal variability of the Andean Beni River at Rurrenabaque is key to understand the fine sediment dynamics at Cachuela Esperanza.
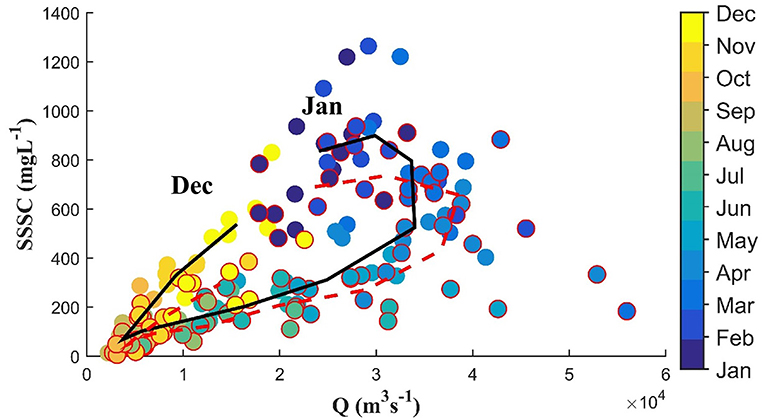
Figure 3. Water discharge (Q)–Surface suspended sediment concentrations (SSSC) relationship at Porto Velho in the Upper Madeira basin. Black (red dash) lines represent the 2003–2009 (2010–2017) period. Dots bordered in red indicate SSSC data of the 2010–2017 period.
The SSSC, Q and rainfall time evolution were evaluated at Porto Velho and Rurrenabaque gauge stations and the watersheds they monitor, for each month separately (Figure 4). In December, a negative SSSC trend (MKτ = −0.48, Sen = −24.7, p < 0.05; Figure 4A), was identified at Porto Velho for 2003–2017, accompanied by a downward break in 2010 (p < 0.10) and a change of distribution after the Mann-Whitney test (w = 50; p < 0.05). There is no significant change in the December SSSC at Rurrenabaque or in discharge for both stations (Figures 4A,D). Rainfall in December for the whole Upper Madeira basin monitored at Porto Velho does not exhibit a significant trend either (Figure 4A).
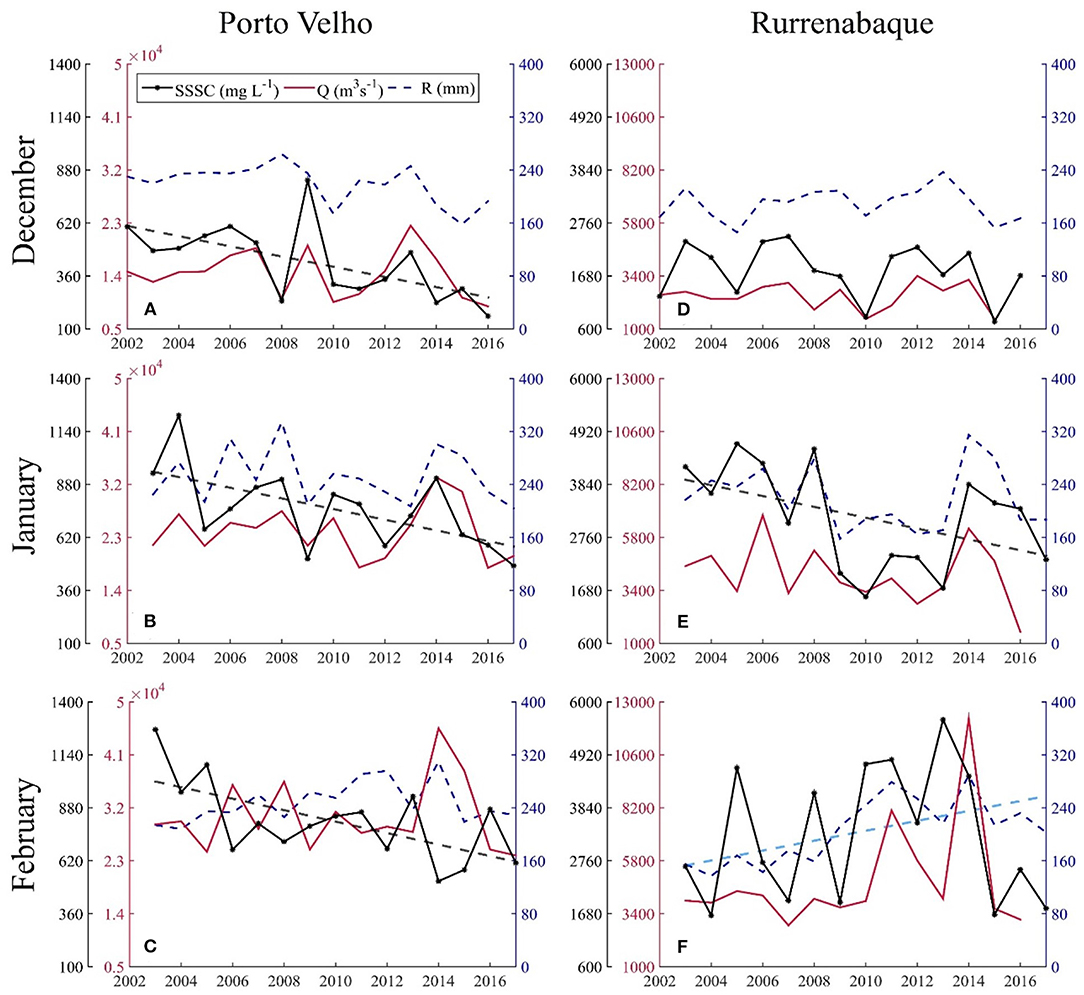
Figure 4. Inter-annual rainfall (R, blue dash line), water discharge (Q, red line), and surface suspended sediment concentration (SSSC, black asterisk line) from December till February at Porto Velho on the Madeira River (A–C) and at Rurrenabaque on the Andean Beni River (D–F). The left y-axis for SSSC (black) and Q (red), right y-axis for R: note that the left y-axis differs according to the magnitude of the variable monitored by the stations. Only significant trends (p < 0.10) are plotted in dash lines.
In January, a negative trend for the SSSC time-series (MKτ = −0.43, Sen = −29.0, p < 0.05; Figure 4B) in 2003–2017 was detected at Porto Velho. At Rurrenabaque a significant negative January SSSC trend (MKτ = −0.33, Sen = −120.6, p < 0.10) for the 2003–2017 period and a downward break (p < 0.10) in 2008 were detected (Figure 4E). A noticeable change in SSSC occurred in 2008, from a mean concentration of 4070 mg·L−1 (2003–2008) to 2060 mg·L−1 (2009–2013), which was followed by an upward change from 2014 to 2016 (3760 mg·L−1; Figure 4E). In spite of the good correlation between SSSC and rainfall at Rurrenabaque (τ = 0.67, p < 0.001), neither a significant break nor a trend was detected for January rainfall and discharge at the 90% confidence level for this sub-basin.
A negative SSSC trend (MKτ = −0.35, Sen = −26.8, p < 0.10; Figure 4C) was identified again in February at Porto Velho but no discharge and rainfall trends were detected. In contrast to January, a positive rainfall trend (MKτ = 0.63, Sen = 10.0, p < 0.01) was identified at Rurrenabaque for February (Figure 4F). This suggests a shift in the monthly rainfall peak from January to February from 2003 to 2017 for the Rurrenabaque sub-basin. Indeed, CHIRPS.v2 data shows that between 2003 and 2008 the mean rainfall was 240 mm in January and 160 mm in February. This changed to 175 mm (−27%) and 240 mm (+50%), respectively, for the 2009–2013 period, while a mean rainfall of 240 mm has been recorded for both months since 2014 (Figures 4E,F). The shift in the rainfall between January and February was also detected for the observed rainfall in most of the Andean stations of the Beni and Madre de Dios River basins, when comparing the 2003–2008 and 2009–2013 periods (Figure 5). Supplementary Table 1 summarize trends and breaks tests during these 3 months.
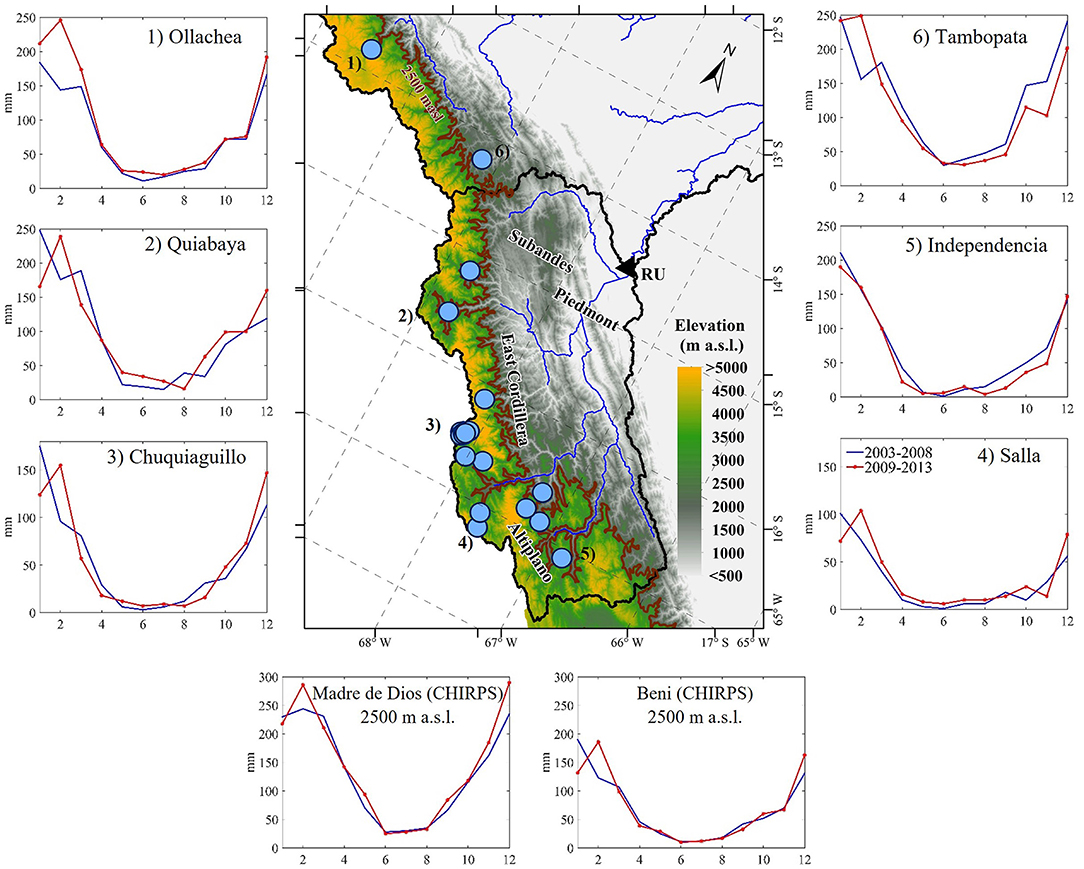
Figure 5. Annual cycle at six of 20 observed stations listed in Table 1 in the Andean region for the two periods, 2003–2008 and 2009–2013 and mean annual cycle for CHIRPS.v2 after the 2500 m a.s.l. Note that the y-axis differs according to the magnitude of the rainfall monitored by the stations.
As spatio-temporal rainfall variability seems to differ along the Upper Madeira basin and no trend was detected for the basin mean rainfall, we evaluated the temporal evolution for each month from December to February during the 2003–2017 period (Figure 6). In December, significant negative rainfall trends (p < 0.10) were observed in the nearby areas of Porto Velho and to the southern part of the Mamoré-Guaporé sub-basins (Figure 6A). A negative but not significant trend was also observed in the upper Madre de Dios River and most of the northern part of the Guaporé sub-basins and a positive not significant elsewhere. Negative but mostly not significant rainfall trends were observed in January in the Andean part of the Beni and Madre de Dios watersheds and in the Guaporé sub-basin. Only a few regions in the Mamoré-Guaporé sub-basin exhibited a significant negative trend (p < 0.10). Positive trends were detected in the lowlands to the north of the Upper Madeira basin (Figure 6B). A significant positive trend (p < 0.10) was detected for the rainfall in February for the Andean parts of the Beni and Madre de Dios sub-basins and adjacent lowlands (Figure 6C). Because of these spatial differences, the basin-averaged rainfall at Porto Velho did not present a significant change in the December to February period (Figures 4A–C).
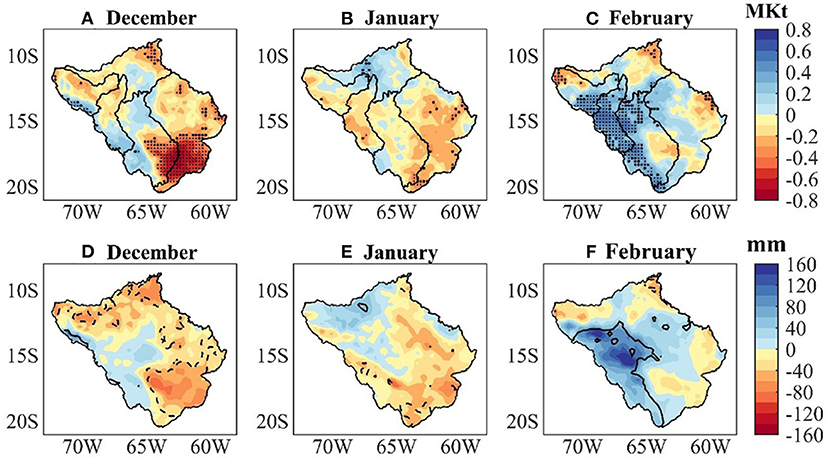
Figure 6. Spatial distribution of Mann-Kendall coefficient (MKτ) values (p < 0.05 are displayed as dots and p < 0.10 as larger dots), indicating the trend for: (A) December, (B) January, and (C) February for the 2003–2017 period. Trends were computed in each CHIRPS.v2 rainfall grid at 0.25° of spatial resolution. The four tributaries' sub-basins are plotted. Differences between the mean monthly rainfall for the 2010–2017 period minus the 2003–2009 period for: (D) December, (E) January, and (F) February in mm. After the Mann-Whitney test, significant at p < 0.10 are contoured, continuous lines for positive differences, and dash lines for negative.
Figures 6D–F confirms that for December the 2010–2017 time period was significantly drier than the 2003–2009 near Porto Velho, most of the Madre de Dios and in the southern part of the Mamoré-Guaporé sub-basins. For January, the 2010–2017 period was drier than the 2003–2009 one in the Andean Beni River sub-basin and the Guaporé watershed. In February the 2010–2017 period was significantly wetter than the 2003–2009 period in the Andes, particularly in the Andean Beni River watershed at Rurrenabaque.
Being December a transition month to the SSSC peak at Porto Velho and since dry conditions were observed in the north region after 2010 (Figures 6A,D), the onset of the rainy season for the four main tributaries sub-basins was evaluated. Figure 7B shows that the rainfall season starts earlier (mid of September) in the Madre de Dios sub-basin than in the other three sub-basins. This is accompanied by a much higher (almost twice) daily rainfall than in the remaining Upper Madeira basin. Because of this and considering the importance the rainfall has on soil erosion at the start of the rainfall season, the precipitation trend for October to December (OND) was evaluated (Figure 7A) for 2003–2017. A significant negative trend (p < 0.05) over the Madre de Dios sub-basin was found. In parallel, the OND intensity as measured by the basin mean daily rainfall (mm.day−1) in this sub-basin exhibits a significant negative trend (p < 0.05) for the same period. In fact, the highest OND daily rainfall (>50 mm.day−1) occurred at the beginning (2003–2005) of the study period (Figure 7C), compared to <40 mm.day−1 for eight of the remaining years.
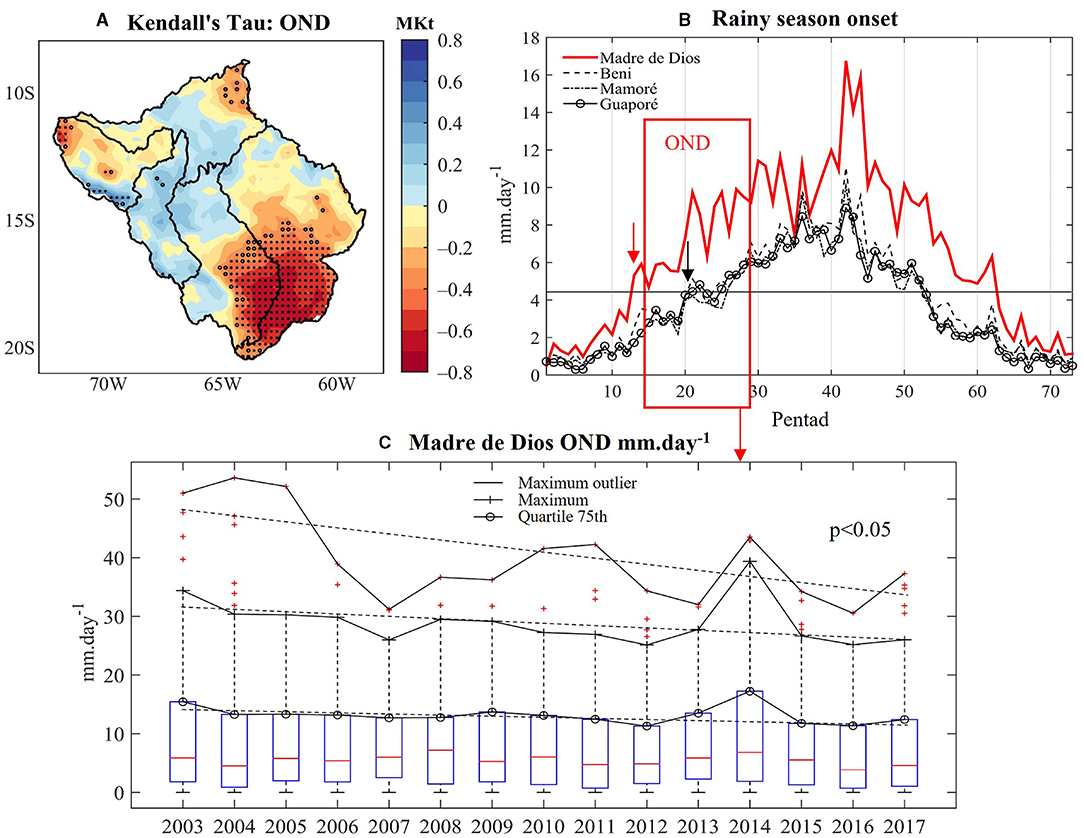
Figure 7. (A) As Figure 6A for October to December (OND); (B) mean annual rainfall cycle (2003–2017) for each sub-basin in pentad. Black horizontal line stands for the mean pentad for the Upper Madeira Basin (4.26 mm.day−1); the onset of rainy season is indicated by red arrow for the Madre de Dios sub-basin (around mid of September) and in black for the Beni, Mamoré, and Guaporé (around mid of October) and; (C) time evolution of the basin mean daily OND: outliers, maximum, and quartile 75th rainfall in the Madre de Dios sub-basin. The three with significative negative trends (p < 0.05).
Discussion
Causes of the Decreasing Trend of SSSC at Porto Velho Station
A significant influence from the Madre de Dios' precipitation on the SSSC recorded at Porto Velho is observed during the first months of the annual cycle, which is associated with the earlier onset of the rainy season in this sub-basin compared to the other main sub-basins of the Upper Madeira. Thus, the interannual negative trend (p < 0.05) for both seasonal and daily OND rainfall for 2003–2017, could have translated in less erosion in the Madre de Dios sub-basin and less fine suspended arriving at Porto Velho at the beginning of the rainy season. It is probably the main cause for the December SSSC decreasing trend at Porto Velho during the study period. The influence of precipitation over this sub-basin on the start of the annual runoff cycle was also observed for an extended period (1981–2017) by Espinoza et al. (2019a), by correlating rainfall in the Upper Madeira basin and runoff at Porto Velho.
The 2003–2017 significant negative rainfall trend in December over the south of the Mamoré-Guaporé basin does not translate into a SSSC trend at the Guayaramerin station (Ayes et al., 2019a) and thus it has no effect on the SSSC time evolution at Porto Velho. This is accounted by the fact that the sediment originated in the southern part of the Guaporé basin does not reach the main stem (Guyot, 1993), and also by the sediment deposition in the piedmont and the Llanos de Moxos (Vauchel et al., 2017).
Changes in the Andean rainfall likely explain the January negative SSSC trend detected at Porto Velho (Figure 4B). In fact, a significant negative SSSC trend and a downward break were detected at Rurrenabaque (Figure 4E) for the 2003–2017 period, giving support to that hypothesis. SSSC values at Rurrenabaque and Porto Velho in January are expected to be related as the average flow travel time from Rurrenabaque to Porto Velho, and thus of the SSSC, was estimated in 12 days by using data from Molina-Carpio et al. (2017) for the Beni River and Molina-Carpio et al. (2008) for the Madeira River. The similar SSSC trend slopes at Porto Velho and Rurrenabaque suggest that a significant negative SSSC trend could also be expected in the Madre de Dios River. Unfortunately, SSSC data is not measured at the Andean Madre de Dios sub-basin, and no data at the river's outlet was available for this research to confirm this assertion.
The negative SSSC trend in February detected at Porto Velho deserves a more comprehensive explanation. Whereas, the sediment recorded at Porto Velho in February comes from the Andes, the sediment dynamics at this time of the year is already influenced by the dilution associated with the base flow at Rurrenabaque (Ayes et al., 2019b). At Porto Velho, the already significant sub-surface and base flow coming from different parts of the floodplain (Miguez-Macho and Fan, 2012; Frappart et al., 2019) also contributes to dilution. This helps to explain the negative correlation observed between Q and SSSC (τ = −0.33, p < 0.01) in February at Porto Velho (Figure 4C). The negative SSSC trend in February in the Madeira River could also be influenced by the SSSC negative trend recorded at Rurrenabaque (Figure 4E) in January, as it takes near 2 weeks for the fine suspended sediment to pass Rurrenabaque and arrive at Porto Velho.
At Rurrenabaque, the negative trend in January rainfall (significant only for some places, Figure 6B) contrasts with the significant positive trend in February. This translates into a veritable shift of the rainfall hyetograph peak from January to February during the study period 2003–2017 (Figure 5). The shift is particularly noticeable in 2009–2013 with a reduction of rainfall in January and an increase in February, when compared to the 2003–2008 period, as described by CHIRPS.v2 and the observed dataset. Espinoza et al. (2019a) observed that the occurrence of the annual cycle peak of rainfall changed from January in 1982–1990 to February for the 2000–2017 period for the whole upper Madeira basin. Segura et al. (2020) detected changes in the atmospheric circulation mechanisms that controls DJF rainfall over the southern tropical Andes, since the beginning of the 2000's. The authors related the changing conditions to the meridional circulation between the tropical North Atlantic and western Amazon basin. These changes in the atmospheric circulation have moistened the mid-troposphere over western tropical South America. Therefore, the authors identified a decline in the inter-annual variability of rainfall during DJF for the Altiplano, including the La Paz River sub-basin as previously observed by Mamani (2019). La Paz sub-basin provides about 40% of the suspended sediment load recorded at Rurrenabaque (Guyot, 1993). The reported changes in the inter-annual variability of rainfall during the DJF wet season suggest possible changes at shorter time scales (i.e., daily or hourly), probably affecting rainfall erosivity.
Ayes et al. (2019b) found a long-term coincidental peak of rainfall and SSSC at Rurrenabaque in January. As expected from the high correlation between monthly rainfall and SSSC, the same authors identified a linear function relating the direct surface flow and SSSC at Rurrenabaque for the 2003–2016 period. The positive rainfall trend in February does not have a concomitant SSSC trend (Figures 4F, 6C). This could be explained by the relevance of the base flow that dilutes SSSC from February onwards in the Rurrenabaque watershed (Ayes et al., 2019b). Thus, the positive rainfall trend in February does not necessarily convert to a rise in SSSC. This effect is further amplified at Porto Velho because of the Llanos de Moxos floodplain in February and the time that suspended sediment takes to arrive at that station.
In a scenario of increasing deforestation in the Andean-Amazon region (Gutierrez-Cori et al., 2021) that would expect to lead to increasing erosion and sediment transport (Martinez et al., 2009), it is someway striking to detect a decrease in the SSSC in the Madeira River which could not be related to the dams built during the same time span. It also stresses that some processes should be analyzed at a relatively small spatial scale and are to be associated with changes occurring in specific regions.
Limitations of the Study and Perspectives
Carrying out continuous monitoring for more than 20 years in the Amazon Basin is a challenge and certainly it has limitations that are related to logistics, economics, and people's safety. However, throughout these years, within the HYBAM Observatory, an attempt has been made to reduce these limitations and uncertainties in the sampling protocols which is documented in Guyot (1993), Filizola et al. (2009), Espinoza-Villar et al. (2013), Vauchel et al. (2017), and Armijos et al. (2020). The present-day suspended sediment sampling strategy does not allow a refined spatio-temporal variability assessment. Ideally the sampling strategy would include measurements over the entire cross-section of the river laterally and vertically, for both water discharge and suspended sediment concentration, on a daily time step. However, the cost of such endeavor on the stations of the Madeira River and Amazon basins, would be unbearable for the HYBAM Observatory and national networks (Vauchel et al., 2017). Yet, efforts have been made through the years by the Observatory to assess the representativity of sparse measurements and their uncertainties, from Guyot (1993) to Armijos et al. (2020), among others. In Amazonian rivers located at the lowlands, the 10-day samplings have shown low variability within the month. A 7-day sampling is done at piedmont and Andean stations as Rurrenabaque, after observing that these stations exhibit a high variability (Guyot, 1993; Vauchel et al., 2017). The possible bias related to the sampling frequency may affect the long-term estimates of the magnitude of the sediment concentration, but less so its trend and shift.
It is worth to mention that sediment dynamics in the Madeira basin also differentiates according to particle size. As documented by Armijos et al. (2020) coarse sandy sediment flux is strongly related to water discharge, while the fine sediment flux is mainly controlled by the seasonal cycle of precipitation. Guyot et al. (1999) found that 97–98% of the suspended sediment transported by the Andean tributaries of the Upper Madeira River should be classified as fine. However, a proper separation of fine and sand suspended sediment has not been implemented at the piedmont station of Rurrenabaque on the Beni River. This could translate into the sand fraction being underestimated in this Andean tributary. Thus a better sampling method at this station would allow for a reduction of uncertainties, particularly for the coarse fraction of suspended sediments. At Porto Velho, Espinoza-Villar et al. (2013) found that fine particles constitute 99% of the surface suspended sediment and observed a homogeneity of SSSC for the river width, which is not the case for the Solimoes or Negro Rivers (Filizola et al., 2009 and Marinho et al., 2021). On the other hand, sand concentration increases with depth along the water column and it has been estimated that 9-12% of total suspended load and more than 90% of the bedload at Porto Velho is made up of sands (Furnas-Odebrecht and ANA, cited by Molina-Carpio et al., 2008; Armijos et al., 2020). A recent study (Marques et al., 2018) found that the sand share in the suspended sediment load at Porto Velho has fallen to 4% since 2012, coinciding with the Madeira River closure by the Santo Antonio and Jirau dams. A significant part of the sand recorded at Porto Velho could come from the rivers traversing the Brazilian shield, like the Guapore River and the Madeira River itself. Meanwhile most, if not nearly all, fine suspended sediment load originates in the Andes as wash load.
Because of the particle size characteristics of the Madeira River and its main tributaries described above, a dams-induced sedimentation of sands, both transported in suspension and as bedload, should be expected (and it was, see Molina et al., 2008). The available data, which is expected to increase in the near future, would confirm this assertion. On the other hand, sedimentation of SSSC and the fine fraction associated to dam-operation is probably negligible and would not change our main findings and conclusions.
Conclusions
The results of this study indicate that for a relatively short period (2003–2017) significant diminution in the surface suspended sediment concentration (SSSC) time-series of the Upper Madeira basin are associated with the spatiotemporal rainfall variability in the basin. The negative rainfall trend (p < 0.05) from October to December in most of the Madre de Dios River sub-basin, and in the northeastern part of the Upper Madeira basin near Porto Velho, resulted in a probable decline in the Madeira River SSSC at the beginning of the SSSC annual cycle. Whereas, a decline in the SSSC contribution of the Andean regions of the Beni and Madre de Dios rivers is the likely cause of the January SSSC negative trend (p < 0.10) at Porto Velho. This was related to a shift of the rainfall peak from January to February during the study period. During 2009–2013 the mean January rainfall in those Andean regions dropped by more than 20% from the 2003–2008 period and with it the mean SSSC fell nearly 50% at Rurrenabaque. Conversely, the mean February rainfall increased nearly 50% after 2008 in the Andean part of the Beni and Madre de Dios sub-basins. The positive rainfall trend (p < 0.05) during February rainfall did not generate a positive SSSC trend as the sediment dynamics in this month is already influenced by the dilution associated with base flow at Rurrenabaque (as shown in Ayes et al., 2019b) and the Llanos de Moxos floodplain. It is also likely that the diminution in the interannual DJF rainfall variability, including rainfall extremes from 2002 onwards (e.g., Mamani, 2019; Segura et al., 2020) in the semiarid and subhumid regions that provides most of the fine sediment at Rurrenabaque had a relevant influence on the erosion processes and SSSC trend at this station.
These changes in the rainfall and SSSC coincided with the construction of the Jirau and Santo Antonio dams and the time when they started to operate. Although the hydroelectric facilities could have an influence, the sediment trapping effect of the dams is probably of minor importance for the SSSC dynamics. However, it probably was very relevant for coarser particles (fine and medium sands) transported by the Madeira River. This study shows that the decline of SSSC in the Madeira River should not be evaluated just on the basis of the SSSC data downstream from the hydroelectric facilities, but also on the basis of the natural processes upstream from the dams.
These results illustrate the complexity of the Madeira sediment dynamics and its relationship with changes in the regional hydrological cycle. Changes in the rainfall peak over the Andean Beni and Madre de Dios region, which covers <1.8% of the Amazon Basin, were related to a decline of fine suspended sediments in the middle stretch of the Madeira River. The changes in the rainfall patterns occur at a time when the Upper Madeira Basin is undergoing a major biophysical transition (e.g., deforestation and construction of major infrastructure projects) that usually is expected to act in the opposite way, increasing soil erosion and sediment transport. These results highlight the need to identify the complex relationships acting on the several components of the hydrological cycle in the Amazon-Andes transition zone, where erosion and sediment transport are key processes for the preservation of the Amazon biodiversity. Further insights and knowledge could emerge as more information becomes available.
Data Availability Statement
Publicly available datasets were analyzed in this study. This data can be found at: https://hybam.obs-mip.fr/, https://www.geography.unibe.ch/research/climatology_group/research_projects/decade/index_eng.html, and https://data.chc.ucsb.edu/products/CHIRPS-2.0/.
Author Contributions
IA: conceptualization, methodology, formal analysis, investigation, writing-original draft, and visualization. JM-C: conceptualization, methodology, formal analysis, investigation, validation, and writing-original draft. JE: conceptualization, methodology, formal analysis, validation, and writing-original draft. OG-C: methodology, formal analysis, validation, visualization, and writing-review, and editing. WC: writing-review, editing, and visualization. FF: validation, writing-review, and editing. EA, RE-V, and NF: writing-review and editing. JA: methodology, writing-review, and editing. All authors contributed to the article and approved the submitted version.
Funding
JM-C was supported by the Universidad Mayor de San Andres (UMSA) within the framework provided by the HYdrogéochimie du Bassin AMazonien (HYBAM) program and PHYBAAM (Processus Hydrologiques des Bassins Andins Amazoniens) project. JE was supported by the French AMANECER-MOPGA project funded by ANR and IRD (ref. ANR-18-MPGA-0008). OG-C was supported by French MOPGA program funded by Sorbonne Université. WC was supported by the Universidad del Valle (Cali-Colombia).
Conflict of Interest
The authors declare that the research was conducted in the absence of any commercial or financial relationships that could be construed as a potential conflict of interest.
Publisher's Note
All claims expressed in this article are solely those of the authors and do not necessarily represent those of their affiliated organizations, or those of the publisher, the editors and the reviewers. Any product that may be evaluated in this article, or claim that may be made by its manufacturer, is not guaranteed or endorsed by the publisher.
Acknowledgments
We would like to thank ANA from Brazil, SENAMHI from Bolivia, SENAMHI from Peru and the HYBAM Observatory of the Institut de Recherche pour le Développement (IRD) for providing the hydrological and sediment data. Additionally, to the Climate Hazards Group for generating the satellite-rainfall based product used in this study and Hunziker et al. (2017) for the homogenized rainfall data. We thank Waldo Lavado from SENAMHI in Peru, Rogério Marinho from UFAM and Henrique Roig from UnB in Brazil, Diego Pons from Colorado State University in USA, Jean-Sébastien Moquet from IRD in France, two reviewers and the editor for their help in this study.
Supplementary Material
The Supplementary Material for this article can be found online at: https://www.frontiersin.org/articles/10.3389/frwa.2021.738527/full#supplementary-material
References
Almeida, R. M., Hamilton, S. K., Rosi, E. J., Arantes, J. D., Barros, N., Boemer, G., et al. (2019). Limnological effects of a large Amazonian run-off-river dam on the main river and drowned tributary valley. Nature 9:16846. doi: 10.1038/s41598-019-53060-1
Almeida, R. M., Hamilton, S. K., Rosi, E. J., Barros, N., Doria, C. R. C., Flecker, A. S., et al. (2020). Hydropeaking operations of two run-of-river mega-dams alter downstream hydrology of the largest Amazon tributary. Front. Environ. Sci. 8:120. doi: 10.3389/fenvs.2020.00120
Anderson, E. P., Jenkins, C. N., Heilpern, S., Madonado-Ocampo, J. A., Carvajal-Vallejos, F. M., Encalada, A. C., et al. (2018). Fragmentation of Andes-to-Amazon connectivity by hydropower dams. Sci. Adv. 4:eaao1642. doi: 10.1126/sciadv.aao1642
Arias, P. A., Fu, R., Vera, C, and Rojas, M. (2015). A correlated shortening of the North and South American monsoon seasons in the past few decades. Clim. Dyn. 45, 3183–3203. doi: 10.1007/s00382-015-2533-1
Armijos, E., Crave, A., Espinoza, J. C., Filizola, N., Espinoza-Villar, R., Ayes, I., et al. (2020). Rainfall control on Amazon sediment flux synthesis from 20 years of monitoring. Environ. Res. Commun. 2:051008. doi: 10.1088/2515-7620/ab9003
Armijos, E., Crave, A., Espinoza-Villar, R., Fraizy, P., Dos Santos, A. L. M. R., Sampaio, F., et al. (2017). Measuring and modeling vertical gradients in suspended sediments in the Solimões/Amazon River. Hydrol. Process. 31, 654–667. doi: 10.1002/hyp.11059
Ayes, I., Armijos, E., Espinoza-Villar, R., Espinoza, J. C., Molina-Carpio, J., Ayala, J. M., et al. (2019a). Decline of fine suspended sediments in the Madeira River Basin (2003-2019). Water 11:514. doi: 10.3390/w11030514
Ayes, I., Callau-Poduje, A. C., Molina-Carpio, J., Ayala, J. M., Armijos, E., Espinoza-Villar, R., et al. (2019b). On the relationship between suspended sediment concentration, rainfall variability and groundwater: an empirical and probabilistic analysis for the Andean Beni River, Bolivia (2003-2016). Water 11:2497. doi: 10.3390/w11122497
Berbery, E. H., and Collini, E. A. (2000). Springtime precipitation and water vapor flux over Southeastern South America. Mon. Weather Rev. 128, 1328-1346. doi: 10.1175/1520-0493(2000)128<1328:SPAWVF>2.0.CO;2
Cavalcante, R. B. L., Ferreira, D. B., da, S., Pontes, P. R. M., Tedeschi, R. G., da Costa, C. P. W., et al. (2020). Evaluation of extreme rainfal índices from CHIRPS precipitation estimates over the Brazilian Amazonia. Atmos. Res. 238:104879. doi: 10.1016/j.atmosres.2020.104879
Dos Santos, A. L. M. R., Martinez, J. M., Filizola Jr., N. P., Armijos, E., and Alves, L. G. S. (2018). Purus River suspended sediment variability and contributions to the Amazon River from satellite data (2000-2015). Geosci. Rep. 350, 13–19. doi: 10.1016/j.crte.2017.05.004
Espinoza, J. C., Chavez, S., Ronchail, J., Junquas, C., Takahashi, K., and Lavado, W. (2015). Rainfall hotspots over the southern tropical Andes: Spatial distribution, rainfall intensiry, and relations with large-scale atmospheric circulation. Water Resour. Res. 5, 3459–3475. doi: 10.1002/2014WR016273
Espinoza, J. C., Ronchail, J., Marengo, J. A., and Segura, H. (2019b). Contrasting North-South changes in Amazon wet-day and dry-day frequency and related atmospheric features (1981-2017). Clim. Dyn. 52, 5413–5430. doi: 10.1007/s00382-018-4462-2
Espinoza, J. C., Sörensson, A., Ronchail, J., Molina-Carpio, J., Segura, H., Gutierrez-Cori, O., et al. (2019a). Regional hydro-climatic changes in the Southern Amazon Basin (Upper Madeira Basin) during the 1982-2017 period. J. Hydrol. Reg. Stud. 26:100637. doi: 10.1016/j.ejrh.2019.100637
Espinoza-Villar, R., Martinez, J.-L., Guyot, J.-L., Fraizy, P., Armijos, E., Crave, A., et al. (2012). The integration of field measurements and satellite observations to determine river solid loads in poorly monitored basins. J. Hydrol. 444–445, 221–228. doi: 10.1016/j.jhydrol.2012.04.024
Espinoza-Villar, R., Martinez, J.-L., Le Texier, M., Guyot, J.-L., Fraizy, P., Meneses, P. R., et al. (2013). A study of sediment transport in the Madeira River, Brazil, using MODIS remote-sensing images. J. S. Am. Earth Sci. 44, 45–54. doi: 10.1016/j.jsames.2012.11.006
Farr, T. G., Rosen, P. A., Caro, E., Crippen, R., Duren, R., Hensley, S., et al. (2007). The Shuttle Radar Topography Mission. Rev. Geophys. 45. doi: 10.1029/2005RG000183
Filizola, N., Seyler, F., Mourão, M. H., Arruda, W., Spínola, N., and Guyot, J. L. (2009). Study of the variability in suspended sediment discharge at Manacapuru, Amazon River, Brazil. Latin Am. J. Sedimentol. Basin Anal. 16, 93–99.
Frappart, F., Papa, F., Günter, A., Tomasella, J., Pfeffer, J., Ramillien, G., et al. (2019). The spatio-temporal variability of groundwater storage in the Amazon River Basin. Adv. Water Resour. 124, 41–52. doi: 10.1016/j.advwatres.2018.12.005
Fu, R., Yin, L., Li, W., Arias, P. A., Dickinson, R. E., Huang, L., et al. (2013). Increased dry-season length over southern Amazonia in recent decades and its implication for future climate projection. Proc. Natl. Acad. Sci. U. S. A. 110, 18110–5. doi: 10.1073/pnas.1302584110
Funk, C., Verdin, A., Michaelsen, J., Peterson, P., Pedreros, D., and Husak, G. (2015). A global satellite assisted precipitation climatology. Earth Syst. Sci. Data Discuss. 8, 401–425. doi: 10.5194/essdd-8-401-2015
Gutierrez-Cori, O., Espinoza, J. C., Li, Z. X. L., Wongchuig, S., Arias, P. A., Ronchail, J., et al. (2021). On the Hydroclimate-vegetation relationship on the southwestern Amazon during the 2000-2019 period. Front. Water 3:648499. doi: 10.3389/frwa.2021.648499
Guyot, J.-L. (1993). Hydrogéochimie des fleuves de l'Amazonie bolivienne (Collection Etudes et Thèses). ORSTOM, France.
Guyot, J.-L., Jouanneau, J.-M., and Wasson, J.-G. (1999). Characterization of river bed and suspended sediments in the Rio Madeira drainage basin (Bolivian Amazonia). J. S. Am. Earth Sci. 12, 401–410. doi: 10.1016/S0895-9811(99)00030-9
Guyot, J.-L., Jouanneau, J. M., Soares, L., Boaventura, G. R., Maillet, N., and Lagane, C. (2007). Clay mineral composition of river sediments in the Amazon basin. Catena 71, 340–356. doi: 10.1016/j.catena.2007.02.002
Hunziker, S., Gubler, S., Calle, J., Moreno, I., Andrade, M., Velarde, F., et al. (2017). Identifying, attributin, and overcoming common data quality issues of manned station observations. Int. J. Climatol. 37, 4131–4145. doi: 10.1002/joc.5037
International Hydropower Association (IHA) (2017). Case study: Jirau, Brazil. Available online at: https://www.hydropower.org/case-studies/brazil-jirau (accessed September 1, 2020).
Kendall, M. G. (1938). A new measure of rank correlation. Biometrika 30, 81–93. doi: 10.1093/biomet/30.1-2.81
Lanzante, J. R. (1996). Resistant, robust and non-parametric techniques for the analysis of climate data: theory and examples, including applications to historical radiosonde station data. R. Meteorol. Soc. 16, 1197–1226.
Latrubesse, E. M., Arima, E. Y., Dunne, T., Park, E., Baker, V. R., d'Horta, F., et al. (2017). Damming the rivers of the Amazon basin. Nature 546, 363–369. doi: 10.1038/nature22333
Li, T., Wang, S., Liu, Y., Fu, B., and Gao, D. (2020). Reversal of the sediment load increase in the Amazon basin influenced by divergent trends of sediment transport from the Solimões and Madeira Rivers. CATENA 195:104804. doi: 10.1016/j.catena.2020.104804
Lima, M. A. L., Carvalho, A. R., Nunes, M. A., Angelini, R., and Doria, C. R. C. (2020). Declining fisheries and increasing prices: the economic cost of tropical rivers impoundment. Fish. Res. 221:105399. doi: 10.1016/j.fishres.2019.105399
Mamani, L. (2019). Variabilidad hidroclimática espacio-temporal en la cuenca andina del Río Beni. La Paz: Tesis de Ingeniería Civil, Universidad Mayor de San Andrés, 113.
Mann, H. B. (1945). Nonparametric tests agains trend. Econometrica 13, 245–259. doi: 10.2307/1907187
Mann, H. B., and Whitney, D. R. (1947). On a test of whether one of two random variables is stochastically larger than the other. Ann. Math. Stat. 18, 50–60. doi: 10.1214/aoms/1177730491
Marinho, R. R., Harmel, T., Marinez, J. M., and Filizola Jr., N. P. (2021). Spatiotemporal dynamics of suspended sediments in the Negro River, amazon basin, from in situ and sentinel-2 remote sensing Data. ISPRS Int. J. Geo Inf. 10:86. doi: 10.3390/ijgi10020086
Marinho, T., Filizola, N., Martinez, J.-M., Armijos, E., and Nascimento, A. (2018). Suspended Sediment Variability at the Solimões and Negro confluence between May 2013 and February 2014. Geosciences 8:265. doi: 10.3390/geosciences8070265
Marques, F., Strasser, M., and Carvalho, N. (2018). “Avaliação da sazonalidade da descarga sólida e da granulometria no rio Madeira,” in XIII Encontro Nacional de Engenharia de Sedimentos I Particulas das Americas (Vitoria).
Martinez, J. M., Guyout, J. L., Filizola, N., and Sondag, F. (2009). Increase in suspended sediment discharge of the Amazon River assessed by monitoring network and satellite data. Catena 79, 257–264. doi: 10.1016/j.catena.2009.05.011
McClain, M. E., and Naiman, R. J. (2008). Andean influences on the biogeochemistry and ecology of the Amazon River. BioScience 58, 325–336. doi: 10.1641/B580408
Miguez-Macho, G., and Fan, Y. (2012). The role of groundwater in the Amazon water cycle: 1. Influence on seasonal streamflow, flooding and wetlands. Geophys. Res. 117. doi: 10.1029/2012JD017539
Molina, A., Govers, G., Poesen, J., Van Hemelrick, H., De Bievre, B., and Vanacker, V. (2008). Environmental factors controlling spatial variation in sediment yield in a central Andean mountain area. Geomorphology 98, 176–186. doi: 10.1016/j.geomorph.2006.12.025
Molina-Carpio, J., Espinoza, J. C., Vauchel, P., Ronchail, J., Caloir, B. G., Guyot, J.-L., et al. (2017). Hydroclimatology of the Upper Madeira River basin: spatio-temporal variability and trends. Hydrol. Sci. J. 62, 911–927. doi: 10.1080/02626667.2016.1267861
Molina-Carpio, J., Ledezma, F., and Vauchel, P. (2008). “Estudio del río Madera: Remanso hidraúlico y sedimentación,” in Bajo el Caudal: Estudio del río Madera: Remanso hidraúlico y sedimentación, eds P. Molina and A, Carrasco (La Paz: FOBOMADE), 95–152.
Nobre, C. A., Sampaio, G., Borma, I. S., Castilla-Rubio, J. C., Silva, J. S., and Cardoso, M. (2016). Land-use and climate change risks in the amazon and the need of a novel sustainable development paradigm. Proc. Natl. Acad. Sci U.S.A. 113, 10759–10768. doi: 10.1073/pnas.1605516113
Noriega, E. (2018). Evaluación y tratamiento estadístico de datos de precipitación de los productos TRMM y CHIRPS y su aplicación práctica en la cuenca Andina del Rio Beni (Tesis). de Ingeniería Civil, Universidad Mayor de San Andrés, La Paz, Bolivia.
Paca, V. H., da Motta Espinoza-Dávalos, G. E., Moreira, D. M., and Comair, G. (2020). Variability of trens in precipitation across the Amazon River Basin determined from the CHIRPS precipitation product and from station records. Water 12:1244. doi: 10.3390/w12051244
Pepin, E., Guyot, J.-L., Armijos, E., Bazan, H., Fraisy, P., Moquet, J. S., et al. (2013). Climatic control on eastern Andean denudation rates (Central cordillera from Ecuador to Bolivia). J. S. Am. Earth Sci. 44, 85–93. doi: 10.1016/j.jsames.2012.12.010
Pettitt, A. N. (1979). A non-parametric approach to the change-point problem. Appl. Statist. 28, 126–155. doi: 10.2307/2346729
Restrepo, J. D., Kettner, A. J., and Syvitski, J. P. M. (2015). Recent deforestation causes rapid increase in river sediment load in the Colombian Andes. Anthropocene 10, 13–28. doi: 10.1016/j.ancene.2015.09.001
Satgé, F., Roelland, D., Bonnet, M., Molina-Carpio, J., and Pillco, R. (2019). Consistency of satellite-based precipitation products in space and over time compared with gauge observations and snow-hydrological modelling in the Lake Titicaca region. Hydrol. Earth Syst. Sci. 23, 595–619. doi: 10.5194/hess-23-595-2019
Segura, H., Espinoza, J. C., Junquas, C., Lebel, T., Vuille, M., and Garreaud, R. (2020). Recent changes in the precipitation-driving processes over the southern tropical Andes/western Amazon. Clim. Dyn. 54, 2613–2631. doi: 10.1007/s00382-020-05132-6
Van Damme, P. A., Córdoba-Clavijo, L., Baigún, C., Hause, M., Doria, C. R. C., and Duponchelle, F. (2019). Upstream dam impacts on gilded carfish Brachyplatystoma rousseauxii (Siluriformes: Pimelodidae) in the Bolivian Amazon. Neotrop. Ichthyol. 17:e190118. doi: 10.1590/1982-0224-20190118
Vauchel, P. (2014). Estudio de la crecida 2014 en la cuenca del río Madera. Available online at: www.ore-hybam.org/index.php/eng/content/download/17209/89238/ (accessed February 20, 2019).
Vauchel, P., Santini, W., Guyot, J.-L., Moquet, J. S., Martinez, J.-M., Espinoza, J. C., et al. (2017). A reassessment of the suspended sediment load in the Madeira River basin from the Andes of Peru and Bolivia to the Amazon River in Brazil, based on 10 years of data from the HYBAM monitoring programme. J. Hydrol. 553, 35–48. doi: 10.1016/j.jhydrol.2017.07.018
Keywords: surface suspended sediment concentration, spatio-temporal rainfall variability, trends, Madeira River, Andes
Citation: Ayes Rivera I, Molina-Carpio J, Espinoza JC, Gutierrez-Cori O, Cerón WL, Frappart F, Armijos Cardenas E, Espinoza-Villar R, Ayala JM and Filizola N (2021) The Role of the Rainfall Variability in the Decline of the Surface Suspended Sediment in the Upper Madeira Basin (2003–2017). Front. Water 3:738527. doi: 10.3389/frwa.2021.738527
Received: 09 July 2021; Accepted: 25 August 2021;
Published: 20 September 2021.
Edited by:
Soumendra Nath Bhanja, Oak Ridge National Laboratory (DOE), United StatesReviewed by:
Narayan Shrestha, Environment and Climate Change, CanadaBadronnisa Yusuf, Putra Malaysia University, Malaysia
Copyright © 2021 Ayes Rivera, Molina-Carpio, Espinoza, Gutierrez-Cori, Cerón, Frappart, Armijos Cardenas, Espinoza-Villar, Ayala and Filizola. This is an open-access article distributed under the terms of the Creative Commons Attribution License (CC BY). The use, distribution or reproduction in other forums is permitted, provided the original author(s) and the copyright owner(s) are credited and that the original publication in this journal is cited, in accordance with accepted academic practice. No use, distribution or reproduction is permitted which does not comply with these terms.
*Correspondence: Irma Ayes Rivera, ayesrivera@hotmail.com
†These authors have contributed equally to this work and share first authorship