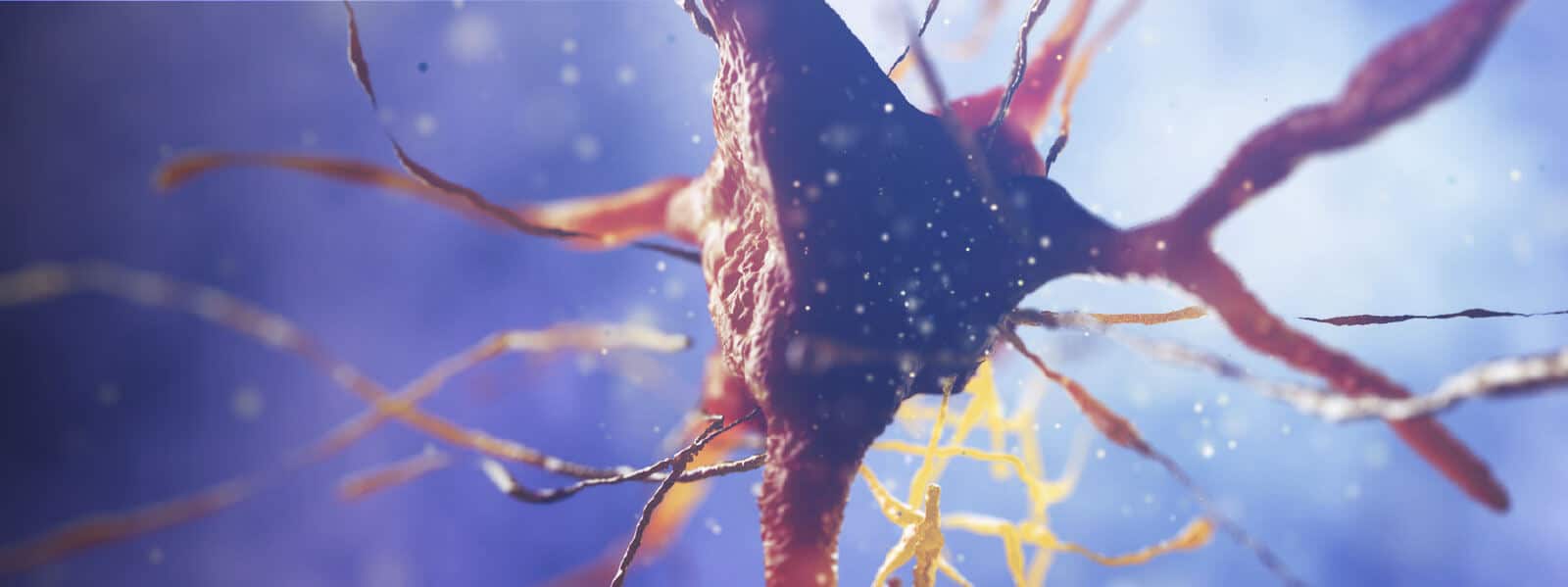
Physiology News Magazine
Metamorphosis from tadpole to frog: a tale of two networks
The transformation from tadpole to frog has long fascinated scientists and laymen alike, yet little is known about the neural basis of the switch in locomotory strategy from tail oscillations to limb kicking. Our new in vitro preparations herald unique opportunities to study neural plasticity accompanying metamorphosis
Features
Metamorphosis from tadpole to frog: a tale of two networks
The transformation from tadpole to frog has long fascinated scientists and laymen alike, yet little is known about the neural basis of the switch in locomotory strategy from tail oscillations to limb kicking. Our new in vitro preparations herald unique opportunities to study neural plasticity accompanying metamorphosis
Features
Denis Combes (1*), Simon Merrywest (2), John Simmers (1), Keith Sillar (2)
1: Laboratoire de Physiologie et Physiopathologie de la Signalisation Cellulaire, UMR CNRS 5543, Universités Bordeaux 1 & Victor Segalen Bordeaux 2, France
2: School of Biology, Bute Medical Buildings, University of St Andrews, Scotland, UK
https://doi.org/10.36866/pn.58.17
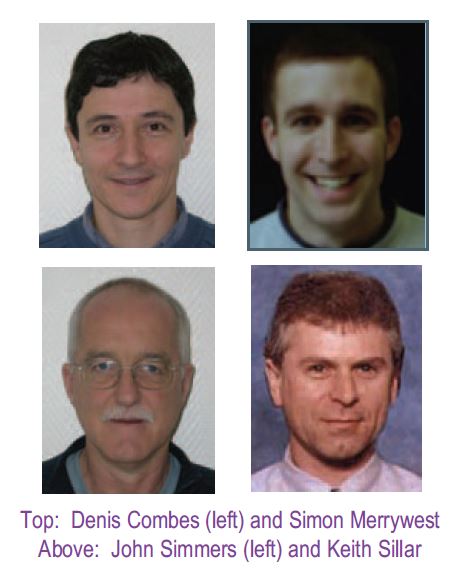
Within the vertebrates there are two principal modes of locomotion: limbbased propulsion (such as walking or flying) and axial body movements using the trunk muscles (such as swimming or side-winding). The phylogenetic position and developmental timetable of most species commits them to a body format predisposed for one or other type of movement.
For some amphibian species, however, a body format has evolved which allows for both locomotory modes to occur conjointly, at least at some stages of development. In urodeles (salamanders) this capacity is retained throughout adult life. In anurans (frogs and toads) a remarkable developmental transition, called metamorphosis occurs in which an axial swimming system in tadpoles is progressively superseded during metamorphosis by an adult limb-based locomotor strategy (Shi, 2000). In each case, the organism must possess neural circuitry within the spinal cord underpinning both behaviours simultaneously. The transition during metamorphosis must result from a set of developmental events including neurogenesis, apoptosis and synaptogenesis. Amazingly, all of these coordinated processes must be engaged whilst the organism continues to behave within its environment (in contrast to insect metamorphosis). Moreover, the entire palette of plastic changes occurring in the nervous system during metamorphosis is critically dependent on the presence of thyroid hormone and its subsequent impact on the genome. A detailed knowledge of the underlying neuronal mechanisms is thus of general interest and potential importance in understanding how hormones configure neural networks during development. However, a cellular and systems based neurobiological approach to understanding metamorphosis in a spinal motor system has thus far proven elusive.
To begin to address this issue of developmental neural plasticity, we have recently developed isolated preparations of the spinal cord of the frog Xenopus laevis at different metamorphic stages. These remain viable in vitro, expressing stagespecific motor patterns that would normally drive locomotion in vivo (Combes et al. 2004). Thus in isolated pre-metamorphic preparations, spinal ventral root motor output corresponds to typical fish-like undulatory swimming movements involving alternate bilateral contractions of axial muscles with a characteristic head-totail delay along the body (Roberts et al. 1998). Following tail resorption in postmetamorphic froglets, spinal motor output is now appropriate for rhythmic leg-kicks via slower and bilaterallysynchronous cycles of hindlimb extension and flexion. Thus, in a period of 2-3 weeks the organism’s central locomotor circuitry, which is distributed along the larval spinal cord, is replaced by an adult hindlimb-kick network confined to the lumbar region of the cord.
At intermediate metamorphic stages the earliest movements of the emerging hindlimbs consist initially of bilateral extension movements that maintain the legs in a rearward position during propulsive undulatory swimming (Fig. 1).
However, the bursting pattern of lumbar motorneurons is tightly coordinated with the axial rhythm (Fig. 1 insets) suggesting that alternating lateral displacement of the hindlimbs may actively assist tail-based propulsion.
As the hindlimbs and their muscles continue to develop, this essentially auxiliary locomotor role is superseded by synchronous rhythmic leg movements now with their own independent frequency to provide supplementary propulsive force (Fig. 2). As in the freely-behaving animal, the motor patterns for both axial- and limb-based locomotion can be expressed independently or conjointly (albeit at very different frequencies), thereby confirming the co-existence of separate spinal rhythm generators. However, the temporal coincidence of hindlimb and axial motor rhythms in these transitional metamorphic preparations (Fig. 2 insets) suggests the existence of separate but still functionally-overlapping neural circuits.
Clearly, unravelling such developmental issues in Xenopus spinal locomotor networks now awaits detailed examination at the cellular and network levels. Moreover, our newly developed preparations offer opportunities to pursue this study through multiple approaches from molecular development and endocrinology via physiology, to the ontogeny of motor behaviour.
Acknowledgement
This collaboration is supported by a Research Interchange Grant from the Leverhulme Trust (UK), to whom we are grateful.
References
Combes D, Merrywest SD, Simmers J & Sillar KT (2004) Developmental segregation of spinal networks driving axial and hindlimb based locomotion in metamorphosing Xenopus laevis. J Physiol 559, 17-24.
Roberts A, Soffe SR, Wolf ES, Yoshida M & Zhao FY (1998). Central circuits controlling locomotion in young frog tadpoles. Ann NY Acad Sci 860, 19-34.
Shi YB (2000). Amphibian metamorphosis: from morphology to molecular biology. Wiley Liss, New York.