Abstract
Summary
In this study, we determined that operator positioning precision contributes significant measurement error in high-resolution peripheral quantitative computed tomography (HR-pQCT). Moreover, we developed software to quantify intra- and inter-operator variability and demonstrated that standard positioning training (now available as a web-based application) can significantly reduce inter-operator variability.
Introduction
HR-pQCT is increasingly used to assess bone quality, fracture risk, and anti-fracture interventions. The contribution of the operator has not been adequately accounted in measurement precision. Operators acquire a 2D projection (“scout view image”) and define the region to be scanned by positioning a “reference line” on a standard anatomical landmark. In this study, we (i) evaluated the contribution of positioning variability to in vivo measurement precision, (ii) measured intra- and inter-operator positioning variability, and (iii) tested if custom training software led to superior reproducibility in new operators compared to experienced operators.
Methods
To evaluate the operator in vivo measurement precision, we compared precision errors calculated in 64 co-registered and non-co-registered scan-rescan images. To quantify operator variability, we developed software that simulates the positioning process of the scanner’s software. Eight experienced operators positioned reference lines on scout view images designed to test intra- and inter-operator reproducibility. Finally, we developed modules for training and evaluation of reference line positioning. We enrolled six new operators to participate in a common training, followed by the same reproducibility experiments performed by the experienced group.
Results
In vivo precision errors were up to threefold greater (Tt.BMD and Ct.Th) when variability in scan positioning was included. The inter-operator precision errors were significantly greater than the short-term intra-operator precision (p < 0.001). New trained operators achieved comparable intra-operator reproducibility to experienced operators and lower inter-operator reproducibility (p < 0.001). Precision errors were significantly greater for the radius than for the tibia.
Conclusion
Operator reference line positioning contributes significantly to in vivo measurement precision and is significantly greater for multi-operator datasets. Inter-operator variability can be significantly reduced using a systematic training platform, now available online (http://webapps.radiology.ucsf.edu/refline/).





Similar content being viewed by others
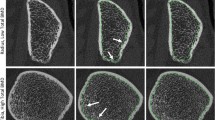
References
Cheung AM, Adachi JD, Hanley DA, Kendler DL, Davison KS, Josse R et al (2013) High-resolution peripheral quantitative computed tomography for the assessment of bone strength and structure: a review by the Canadian Bone Strength Working Group. Curr Osteoporos Rep 11:136–146
Boutroy S, Bouxsein ML, Munoz F, Delmas PD (2005) In vivo assessment of trabecular bone microarchitecture by high-resolution peripheral quantitative computed tomography. J Clin Endocrinol Metab 90:6508–6515
Burghardt AJ, Buie HR, Laib A, Majumdar S, Boyd SK (2010) Reproducibility of direct quantitative measures of cortical bone microarchitecture of the distal radius and tibia by HR-pQCT. Bone 47:519–528
Khosla S, Riggs BL, Atkinson EJ, Oberg AL, McDaniel LJ, Holets M et al (2006) Effects of sex and age on bone microstructure at the ultradistal radius: a population-based noninvasive in vivo assessment. J Bone Miner Res 21:124–131
Macdonald HM, Nishiyama KK, Kang J, Hanley DA, Boyd SK (2011) Age-related patterns of trabecular and cortical bone loss differ between sexes and skeletal sites: a population-based HR-pQCT study. J Bone Miner Res 26:50–62
Kazakia GJ, Tjong W, Nirody JA, Burghardt AJ, Carballido-Gamio J, Patsch JM et al (2014) The influence of disuse on bone microstructure and mechanics assessed by HR-pQCT. Bone 63:132–140
Zhu TY, Griffith JF, Qin L, Hung VW, Fong T-N, Au S-K et al (2014) Alterations of bone density, microstructure and strength of the distal radius in male patients with rheumatoid arthritis: a case-control study with HR-pQCT. J Bone Miner Res:1–42
Rizzoli R, Laroche M, Krieg M-A, Frieling I, Thomas T, Delmas P et al (2010) Strontium ranelate and alendronate have differing effects on distal tibia bone microstructure in women with osteoporosis. Rheumatol Int 30:1341–1348
Seeman E, Delmas PD, Hanley DA, Sellmeyer D, Cheung AM, Shane E et al (2010) Microarchitectural deterioration of cortical and trabecular bone: differing effects of denosumab and alendronate. J Bone Miner Res 25:1886–1894
Melton LJ, Christen D, Riggs BL, Achenbach SJ, Müller R, van Lenthe GH et al (2010) Assessing forearm fracture risk in postmenopausal women. Osteoporos Int 21:1161–1169
Christen D, Melton LJ, Zwahlen A, Amin S, Khosla S, Müller R (2013) Improved fracture risk assessment based on nonlinear micro-finite element simulations from HRpQCT images at the distal radius. J Bone Miner Res 28:2601–2608
Glüer C, Blake G, Lu Y, Blunt B (1995) Accurate assessment of precision errors: how to measure the reproducibility of bone densitometry techniques. Osteoporos Int 5:262–270
MacNeil JA, Boyd SK (2008) Improved reproducibility of high-resolution peripheral quantitative computed tomography for measurement of bone quality. Med Eng Phys 30:792–799
Burghardt AJ, Pialat J-B, Kazakia GJ, Boutroy S, Engelke K, Patsch JM et al (2013) Multicenter precision of cortical and trabecular bone quality measures assessed by high-resolution peripheral quantitative computed tomography. J Bone Miner Res 28:524–536
Engelke K, Stampa B, Timm W, Dardzinski B, de Papp AE, Genant HK et al (2012) Short-term in vivo precision of BMD and parameters of trabecular architecture at the distal forearm and tibia. Osteoporos Int 23:2151–2158
Ellouz R, Chapurlat R, van Rietbergen B, Christen P, Pialat J-B, Boutroy S (2014) Challenges in longitudinal measurements with HR-pQCT: evaluation of a 3D registration method to improve bone microarchitecture and strength measurement reproducibility. Bone 63:147–157
Boyd SK (2008) Site-specific variation of bone micro-architecture in the distal radius and tibia. J Clin Densitom 11:424–430
Mueller TL, van Lenthe GH, Stauber M, Gratzke C, Eckstein F, Müller R (2009) Regional, age and gender differences in architectural measures of bone quality and their correlation to bone mechanical competence in the human radius of an elderly population. Bone 45:882–891
Pialat JB, Burghardt AJ, Sode M, Link TM, Majumdar S (2012) Visual grading of motion induced image degradation in high resolution peripheral computed tomography: impact of image quality on measures of bone density and micro-architecture. Bone 50:111–118
Laib A, Häuselmann HJ, Rüegsegger P (1998) In vivo high resolution 3D-QCT of the human forearm. Technol Health Care 6:329–337
Davis KA, Burghardt AJ, Link TM, Majumdar S (2007) The effects of geometric and threshold definitions on cortical bone metrics assessed by in vivo high-resolution peripheral quantitative computed tomography. Calcif Tissue Int 81:364–371
Burghardt AJ, Kazakia GJ, Ramachandran S, Link TM, Majumdar S (2010) Age- and gender-related differences in the geometric properties and biomechanical significance of intracortical porosity in the distal radius and tibia. J Bone Miner Res 25:983–993
Buie HR, Campbell GM, Klinck RJ, MacNeil JA, Boyd SK (2007) Automatic segmentation of cortical and trabecular compartments based on a dual threshold technique for in vivo micro-CT bone analysis. Bone 41:505–515
Hildebrand T, Ru P (1997) A new method for the model-independent assessment of thickness in three-dimensional images. J Microsc 185:67–75
Müller R, Rüegsegger P (1995) Three-dimensional finite element modelling of non-invasively assessed trabecular bone structures. Med Eng Phys 17:126–133
MacNeil J, Boyd SK (2008) Bone strength at the distal radius can be estimated from high-resolution peripheral quantitative computed tomography and the finite element method. Bone 42:1203–1213
Van Rietbergen B, Odgaard A, Kabel J, Huiskes R (1996) Direct mechanics assessment of elastic symmetries and properties of trabecular bone architecture. J Biomech 29:1653–1657
Mueller TL, Christen D, Sandercott S, Boyd SK, van Rietbergen B, Eckstein F et al (2011) Computational finite element bone mechanics accurately predicts mechanical competence in the human radius of an elderly population. Bone 48:1232–1238
Laib A, Hildebrand T, Häuselmann H, Rüegsegger P (1997) Ridge number density: a new parameter for in vivo bone structure analysis. Bone 21:541–546
Kirmani S, Christen D, van Lenthe GH, Fischer PR, Bouxsein ML, McCready LK et al (2009) Bone structure at the distal radius during adolescent growth. J Bone Miner Res 24:1033–1042
Burrows M, Liu D, McKay H (2010) High-resolution peripheral QCT imaging of bone micro-structure in adolescents. Osteoporos Int 21:515–20
Barnabe C, Feehan L (2012) High-resolution peripheral quantitative computed tomography imaging protocol for metacarpophalangeal joints in inflammatory arthritis: the SPECTRA collaboration. J Rheumatol 39:7–9
Carballido-Gamio J, Bonaretti S, Holets M, Saeed I, McCready L, Majumdar S et al (2013) Automated scan prescription for HR-pQCT: a multi-atlas prospective registration approach. ASBMR
Acknowledgments
The authors thank Isra Saeed and Louise McCready for subject recruitment, Margaret Holets for data acquisition, James Peterson for data management, and Nicholas P. Derrico and the MrOS operators that took part in this study: Deborah Cusick, Shannon Hanson, Kristi Jacobson, Kyla Kent, Patricia Miller, and Nita Webb.
This study was funded by the NIH/NIAMS R01 AR060700 and by The Osteoporotic Fractures in Men (MrOS) study, which is supported by the National Institutes of Health funding. The following institutes provide support: the National Institute on Aging (NIA), the National Institute of Arthritis and Musculoskeletal and Skin Diseases (NIAMS), the National Center for Advancing Translational Sciences (NCATS), and NIH Roadmap for Medical Research under the following grant numbers: U01 AG027810, U01 AG042124, U01 AG042139, U01 AG042140, U01 AG042143, U01 AG042145, U01 AG042168, U01 AR066160, and UL1 TR000128.
Author information
Authors and Affiliations
Corresponding author
Ethics declarations
All subjects signed informed consent to participate in the study, and the Committees on Human Research at UCSF and Mayo Clinic approved study procedures.
Conflict of interest
Nicolas Vilayphiou is an employee of Scanco Medical AG. Serena Bonaretti, Caroline Mai Chan, Andrew Yu, Kyle Nishiyama, Danmei Liu, Stephanie Boutroy, Ali Ghasem-Zadeh, Steven K. Boyd, Roland Chapurlat, Heather McKay, Elizabeth Shane, Mary Bouxsein, Dennis M. Black, Sharmila Majumdar, Eric S. Orwoll, Thomas Lang, Sundeep Khosla, and Andrew J. Burghardt declare that they have no conflict of interest.
Appendix: HR-pQCT operator training
Appendix: HR-pQCT operator training
To train new operators, we developed custom software and a detailed scan positioning protocol. The software comprised two modules, one to train reference line positioning and one to evaluate positioning performance for the purposes of operator certification. The graphical user interface reproduces the original acquisition software (Fig. 6(c)). Graphical control elements on the left side of the interface allowed the user to select training or evaluation modules, and from a series of scout view collections (eight scout images each) according to anatomic site, laterality, and difficulty. In training mode, the software provides operators both immediate and summary feedback on their positioning accuracy. The immediate feedback consists of a traffic light that is illuminated after each reference line is positioned. A green, yellow, or red light is shown, corresponding to “exact”, “good”, or “out of range” positions, respectively. The final feedback consists of a separate window displaying a summary report of operator performance after completing the selected collection (Fig. 4d). Positioning feedback was determined by comparing the user’s reference line position to a gold standard reference position. The gold standard positions were determined through independent consensus of three experienced operators at UCSF. Exact indicates that the operator and reference positions coincided; good indicates that the operator position was within 2 pixels from the reference position; and out of range indicates that the operator position was greater than 2 pixels from the reference position. Two pixels corresponded to 0.34 mm, which was the short-term standard deviation among experienced operators.
We created training and evaluation collections for the radius and tibia from the double-length scans described in “Methods”. A horizontal mirror image of each scout view image was created to have an equal number of “left” and “right” limb images, for a total of 112 scout view images per anatomic site. We divided the scout view images into two groups depending on the degree of difficulty in positioning the reference line. Difficulty was assigned based on the presence or absence of a clear anatomic landmark, as assessed by an experienced UCSF operator. From each of these two groups, 49 images were randomly selected to create the training collections, and we used the remaining seven images to create the evaluation datasets. Both for training and evaluation modules, we created 15 collections of eight images randomly selected within their visibility group. Before accessing the reproducibility experiments, the six new operators had to complete all the datasets of the training module at least once, and had to succeed on at least three sets for the radius and three sets for the tibia. A set was considered successful when the sum of exact and good positions was seven out of eight positions.
Together with the training and evaluation software, we provided specific guidelines to identify the anatomic landmark and to position the reference line, as follow (Fig. 6). For the radius, we defined the anatomic landmark as the peak of the radiocarpal articular surface of the radius, which separates the radiopaque subchondral bone (bright signal) from the radiolucent joint space (dark signal) (Fig. 6 (a, b)). When the peak is clearly visible, the reference line must intersect the peak (Fig. 6 (a)), whereas when the peak is not visible, the reference line must intersect the mid-point of the joint surface (Fig. 5b). The hiker analogy in Fig. 6 (e–h) illustrates the concept. Similarly, for the tibia, the anatomic landmark is the peak of the edge of the tibial plafond, which separates the radiopaque subchondral bone (bright signal) from the radiolucent joint space (dark signal) (Fig. 6 (c, d)). When the peak is clearly visible, the reference line must intersect the center of the peak (Fig. 6 (c)), whereas when the peak is not visible, the reference line must intersect the flat plafond (Fig. 6 (d)).
Guidelines for reference line positioning recommended for the training and evaluation software. In the radius, the reference line intersects (a) the peak when visible or (b) the mid-articular surface when the peak is not visible. Similarly, in the tibia, the reference line intersects (c) the peak when visible or (d) the flat plafond when the peak is not visible. In the radius, the hiker analogy easily explains the positioning of the reference line. The articular surface of the radius (e) can be considered as a hiking path (f). The hiker walks along the path (g) until he reaches the peak where he plants his flag, which coincides with the location where the reference line intersects the edge that represents the articular surface of the radius (h)
Rights and permissions
About this article
Cite this article
Bonaretti, S., Vilayphiou, N., Chan, C.M. et al. Operator variability in scan positioning is a major component of HR-pQCT precision error and is reduced by standardized training. Osteoporos Int 28, 245–257 (2017). https://doi.org/10.1007/s00198-016-3705-5
Received:
Accepted:
Published:
Issue Date:
DOI: https://doi.org/10.1007/s00198-016-3705-5