Abstract
Several types of alloplastic (artificial) grafts, known as scaffolds, have been developed for the treatment of bone defects caused by trauma and/or infection. Among the materials used to manufacture scaffolds, 45S5 Bioglass is a bioceramic that arouses significant interest due to ease preparation and excellent bioactive response. Among the various processing methods cited in the literature for the production of bioactive glass scaffolds, gelcasting is a method that produces macroporous structures, with interconnected and spherical pores and high mechanical strength. However, in the literature there are few reports about bioactive glass scaffolds produced by gelcasting method. In this work, 45S5-BG scaffolds were produced by gelcasting of foams varying the amount of foaming agent in order to optimize the desirable characteristics of the scaffold. The scaffolds show porosity between 70 and 86% and compressive strength of 1.22 ± 0.7 and 0.78 ± 0.4 MPa. In the biological studies, all 45S5-BG scaffolds showed cytocompatibility towards human osteoblastic cells and bioactive properties using SBF assay.











Similar content being viewed by others
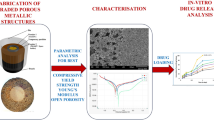
References
Monteiro F, Román JS (2004) Introducción y Desarollo Histórico, 1st edn. Faenza Editrice Ibérica, España, pp 17–26
Ratner B (2004) Biomaterials science: an introduction to materials in medicine, 2nd edn. Academic Press, Cambridge, pp 1–19
Soares G (2005) Biomateriais. In: Fórum de Biotecnologia Biomateriais. UFRJ, Rio de Janeiro, pp 24–28
Holzapfel BM, Reichert JC, Schantz J-T, Gbureck U, Rackwitz L, Nöth U, Jakob F, Rudert M, Groll J, Hutmacher DW (2012) How smart do biomaterials need to be? A translational science and clinical point of view. Adv Drug Deliv Rev 65:581–603. https://doi.org/10.1016/j.addr.2012.07.009
Da Rocha DN, Gobbo LA, Da Silva MHP (2013) Production and characterization of niobate apatite. J Mater Res Technol 2:24–29. https://doi.org/10.1016/j.jmrt.2013.04.007
Hench LL, Wilson J (1993) An introduction to bioceramics, vol 1. Advanced series in ceramic. World Scientific Singapore, Singapore, pp 41–62
Hench LL, Splinter RJ, Allen WC, Greenlee TK (1971) Bonding mechanisms at the interface of ceramic prosthetic materials. J Biomed Mater Res 5:117–141. https://doi.org/10.1002/jbm.820050611
Jones JR, Brauer DS, Hupa L, Greenspan DC (2016) Bioglass and bioactive glasses and their impact on healthcare. Int J Appl Glass Sci 7:423–434. https://doi.org/10.1111/ijag.12252
Chen Q-Z, Thouas G (2011) Fabrication and characterization of sol–gel derived 45S5 Bioglass®–ceramic scaffolds. Acta Biomater 7:3616–3626. https://doi.org/10.1016/j.act.bio.2011.06.005
Chen QZ, Thompson ID, Boccaccini AR (2006) 45S5 Bioglass®—derived glass–ceramic scaffolds for bone tissue engineering. Biomaterials 27:2414–2425. https://doi.org/10.1016/j.biomaterials.2005.11.025
Rahaman MN, Day DE, Bal BS, Fu Q, Jung SB, Bonewald LF, Tomsia AP (2011) Bioactive glass in tissue engineering. Acta Biomater 7:2355–2373. https://doi.org/10.1016/j.actbio.2011.03.016
Gerhardt L-C, Boccaccini AR (2010) Bioactive glass and glass–ceramic scaffolds for bone tissue engineering. Materials 3:3867–3910. https://doi.org/10.3390/ma3073867
Boccaccini AR, Ma PX (2014) Tissue engineering using ceramics and polymers, 2nd edn. Woodhead Publishing, Cambridge, pp 67–114
Ghomi H, Fathi MH, Edris H (2011) Fabrication and characterization of bioactive glass/hydroxyapatite nanocomposite foam by gelcasting method. Ceram Int 37:1819–1824. https://doi.org/10.1016/j.ceramint.2011.03.002
De Sousa E, Ortega FS, Pandolfelli VC (2009) Produção e caracterização de espumas de alumina pelo processo gelcasting sem controle atmosférico. Cerâmica 55:151–156
De Sousa E, Rambo CR, Ortega FS, De Oliveira APN, Pandolfelli VC (2009) Espumas vítreas do sistema Li2O–ZrO2–SiO2–Al2O3 produzidas pelo processo gelcasting. Cerâmica 55:157–162
Sepulveda P, Binner JG (1999) Processing of cellular ceramics by foaming and in situ polymerisation of organic monomers. J Eur Ceram Soc 19:2059–2066. https://doi.org/10.1016/s0955-2219(99)00024-2
Ortega FS, Valenzuela FAO, Scuracchio CH, Pandolfelli VC (2003) Alternative gelling agents for the gelcasting of ceramic foams. J Eur Ceram Soc 23:75–80. https://doi.org/10.1016/s0955-2219(02)00075-4
Jones JR, Hench L (2003) Regeneration of trabecular bone using porous ceramics. Curr Opin Solid State Mater Sci 7:301–307. https://doi.org/10.1016/j.cossms.2003.09.012
Sepulveda R, Ortega FS, Innocentini MDM, Pandolfelli VC (2000) Properties of highly porous hydroxyapatite obtained by the gelcasting of foams. J Am Ceram Soc 83:3021–3024. https://doi.org/10.1111/j.1151-2916.2000.tb01677.x
Sepulveda P, Pandolfelli VC, Rogero SO, Higa OZ, Bressiani JC (1999) Hidroxiapatita porosa produzida através do ‘gelcasting’ de espumas visando aplicações biomédicas. Cerâmica 45:198–202. https://doi.org/10.1590/s0366-6913199000600007
Meng G, Wang H, Zheng W, Liu X (2000) Preparation of porous ceramics by gelcasting approach. Mater. Lett. 45:224–227. https://doi.org/10.1016/s0167-577x(00)00109-9
Wu ZY, Hill RG, Yue S, Nightingale S, Lee PD, Jones JR (2011) Melt-derived bioactive glass scaffolds produced by a gel-cast foaming technique. Acta Biomater 7:1807–1816. https://doi.org/10.1016/j.actbio.2010.11.041
Aguilar-Reyes EA, León-Patiño CA, Jacinto-Diaz B, Lefebvre LP (2012) Structural characterization and mechanical evaluation of bioactive glass 45S5 foams obtained by a powder technology approach. J Am Ceram Soc 95:3776–3780. https://doi.org/10.1111/j.1551-2916.2012.05465.x
Nommeots-Nomm A, Labbaf S, Devlin A, Todd N, Geng H, Solanki AK, Tang HM, Perdika P, Pinna A, Ejeian F, Tsigkou O, Lee PD, Esfahani MHN, Mitchell CA, Jones JR (2017) Highly degradable porous melt-derived bioactive glass foam scaffolds for bone regeneration. Acta Biomater 57:449–461. https://doi.org/10.1016/j.actbio.2017.04.030
Elsayed H, Romero AR, Ferroni L, Gardin C, Zavan B, Bernardo E (2017) Bioactive glass–ceramic scaffolds from novel ‘inorganic gel casting’ and sinter crystallization. Materials 10:1–15. https://doi.org/10.3390/ma10020171
Lefebvre L, Chevalier J, Gremillard L, Zenati R, Thollet G, Bernache-Assolant D, Govin A (2007) Structural transformations of bioactive glass 45S5 with thermal treatments. Acta Mater 55:3305–3313. https://doi.org/10.1016/j.actamat.2007.01.029
Kokubo T, Hata K, Nakamura T, Yamamura T (1991) Apatite formation on ceramics, metals, and polymers induced by a CaO–SiO2-based glass in simulated body fluid. In: Bonfield W, Hastings GW, Tanner KE (eds) Bioceramics, vol 4. Butterworth-Heinemann, London, pp 113–120. ISBN 978-0-7506-0269-3
Bellucci D, Cannillo V, Sola A, Chiellini F, Gazzarri M, Migone C (2011) Macroporous Bioglass®—derived scaffolds for bone tissue regeneration. Ceram Int 37:1575–1585. https://doi.org/10.1016/j.ceramint.2011.01.023
Lopes JH, Magalhaes JA, Gouveia RF, Bertran CA, Motisuke M, Camargo SEA, Triches ES (2016) Hierarchical structures of β-TCP/45S5 bioglass hybrid scaffolds prepared by gelcasting. J Mech Behav Biomed Mater 62:10–23. https://doi.org/10.1016/j.jmbbm.2016.04.028
Gibson LJ, Ashby MF (1999) Cellular solids: structure and properties, 2nd edn. Cambridge University Press, Cambridge, pp 429–452
Bretcanu O, Chatzistavrou X, Paraskevopoulos K, Conradt R, Thompson I, Boccaccini AR (2009) Sintering and crystallisation of 45S5 bioglass® powder. J Eur Ceram Soc 16:3299–3306. https://doi.org/10.1016/j.jeurceramsoc.2009.06.035
Bellucci D, Sola A, Cannillo V (2011) A revised replication method for bioceramic scaffolds. Bioceram Dev Appl 1:1–8. https://doi.org/10.4303/bda/d110401
Sola A, Bellucci D, Raucci MG, Zeppetelli S, Ambrosio L, Cannillo V (2012) Heat treatment of Na2O–CaO–P2O5–SiO2 bioactive glasses: densification processes and postsintering bioactivity. J Biomed Mater Res Part A 100:305–322. https://doi.org/10.1002/jbm.a.33276
Farag MM, Rüssel C (2012) Glass–ceramic scaffolds derived from bioglass and glass with low crystallization affinity for bone regeneration. Mater Lett 73:161–165. https://doi.org/10.1016/j.matlet.2011.12.110
Yao Q, Li W, Yu S, Ma L, Jin D, Boccaccini AR (2015) Multifunctional chitosan/polyvinyl pyrrolidone/45S5 Bioglass® scaffolds for MC3T3-E1 cell stimulation and drug release. Mater Sci Eng C 56:473–480. https://doi.org/10.1016/j.msec.2015.06.046
Motealleh A, Eqtesadi S, Civantos A, Pajares A, Miranda P (2017) Robocast 45S5 bioglass scaffolds: in vitro behavior. J Mater Sci 52:9179–9191. https://doi.org/10.1007/s10853-017-0775-5
Fernandes MH, Gomes PS (2016) Bone cells dynamics during peri-implantitis: a theoretical analysis. JOMR 7:1–19. https://doi.org/10.5037/jomr.2016.7306
Rahaman MN, Xiao W, Fu Q, Huang W (2017) Review—bioactive glass implants for potential application in structural bone repair. Biomed Glasses 3:56–66. https://doi.org/10.1515/bglass-2017-0005
Acknowledgements
The authors would like to thank the São Paulo Research Foundation—FAPESP (Grant: 2015/24659-7), the National Council for Scientific and Technological Development (Grant: 456461/2014-0) and the Erasmus Mundus Program (Be Mundus Project) for the financial support. The authors also acknowledge the use of the analytical instrumentation facility at I3S—Instituto de Investigação e Inovação em Saúde (Portugal) and the Brazilian Nanotechnology National Laboratory (LNNano) for X-ray microtomography images.
Author information
Authors and Affiliations
Corresponding author
Ethics declarations
Conflict of interest
The authors declare no conflict of interest.
Rights and permissions
About this article
Cite this article
de Siqueira, L., Gouveia, R.F., Grenho, L. et al. Highly porous 45S5 bioglass-derived glass–ceramic scaffolds by gelcasting of foams. J Mater Sci 53, 10718–10731 (2018). https://doi.org/10.1007/s10853-018-2337-x
Received:
Accepted:
Published:
Issue Date:
DOI: https://doi.org/10.1007/s10853-018-2337-x