Abstract
A missense mutation in HERC1 provokes loss of cerebellar Purkinje cells, tremor, and unstable gait in tambaleante (tbl) mice. Recently, we have shown that before cerebellar degeneration takes place, the tbl mouse suffers from a reduction in the number of vesicles available for release at the neuromuscular junction (NMJ). The aim of the present work was to study to which extent the alteration in HERC1 may affect other cells in the nervous system and how this may influence the motor dysfunction observed in these mice. The functional analysis showed a consistent delay in the propagation of the action potential in mutant mice in comparison with control littermates. Morphological analyses of glial cells in motor axons revealed signs of compact myelin damage as tomacula and local hypermyelination foci. Moreover, we observed an alteration in non-myelinated terminal Schwann cells at the level of the NMJ. Additionally, we found a significant increment of phosphorylated Akt-2 in the sciatic nerve. Based on these findings, we propose a molecular model that could explain how mutated HERC1 in tbl mice affects the myelination process in the peripheral nervous system. Finally, since the myelin abnormalities found in tbl mice are histological hallmarks of neuropathic periphery diseases, tbl mutant mice could be considered as a new mouse model for this type of diseases.








Similar content being viewed by others
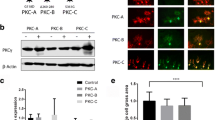
References
Mashimo T, Hadjebi O, Amair-Pinedo F, Tsurumi T, Langa F, Serikawa T, Sotelo C, Guenet JL et al (2009) Progressive Purkinje cell degeneration in tambaleante mutant mice is a consequence of a missense mutation in HERC1 E3 ubiquitin ligase. PLoS Genet 5(12):e1000784
Rossi F, Jankovski A, Sotelo C (1995) Target neuron controls the integrity of afferent axon phenotype: a study on the Purkinje cell-climbing fiber system in cerebellar mutant mice. J Neurosci 15(3 Pt 1):2040–2056
Wassef M, Sotelo C, Cholley B, Brehier A, Thomasset M (1987) Cerebellar mutations affecting the postnatal survival of Purkinje cells in the mouse disclose a longitudinal pattern of differentially sensitive cells. Dev Biol 124(2):379–389
Porras-Garcia ME, Ruiz R, Perez-Villegas EM, Armengol JA (2013) Motor learning of mice lacking cerebellar Purkinje cells. Front Neuroanat 7:4
Bachiller S, Rybkina T, Porras-Garcia E, Perez-Villegas E, Tabares L, Armengol JA, Carrion AM, Ruiz R (2015) The HERC1 E3 Ubiquitin Ligase is essential for normal development and for neurotransmission at the mouse neuromuscular junction. Cell Mol Life Sci 72(15):2961–2971
Hegde AN, Upadhya SC (2007) The ubiquitin-proteasome pathway in health and disease of the nervous system. Trends Neurosci 30(11):587–595
van Tijn P, Hol EM, van Leeuwen FW, Fischer DF (2008) The neuronal ubiquitin-proteasome system: murine models and their neurological phenotype. Prog Neurobiol 85(2):176–193
Sanchez-Tena S, Cubillos-Rojas M, Schneider T, Rosa JL (2016) Functional and pathological relevance of HERC family proteins: a decade later. Cell Mol Life Sci 73(10):1955–1968
Deng HX, Chen W, Hong ST, Boycott KM, Gorrie GH, Siddique N, Yang Y, Fecto F et al (2011) Mutations in UBQLN2 cause dominant X-linked juvenile and adult-onset ALS and ALS/dementia. Nature 477(7363):211–215
Dlamini N, Josifova DJ, Paine SM, Wraige E, Pitt M, Murphy AJ, King A, Buk S et al (2013) Clinical and neuropathological features of X-linked spinal muscular atrophy (SMAX2) associated with a novel mutation in the UBA1 gene. Neuromuscul Disord 23(5):391–398
Ramser J, Ahearn ME, Lenski C, Yariz KO, Hellebrand H, von Rhein M, Clark RD, Schmutzler RK et al (2008) Rare missense and synonymous variants in UBE1 are associated with X-linked infantile spinal muscular atrophy. Am J Hum Genet 82(1):188–193
Rusmini P, Sau D, Crippa V, Palazzolo I, Simonini F, Onesto E, Martini L, Poletti A (2007) Aggregation and proteasome: the case of elongated polyglutamine aggregation in spinal and bulbar muscular atrophy. Neurobiol Aging 28(7):1099–1111
Aghamaleky Sarvestany A, Hunter G, Tavendale A, Lamont DJ, Llavero Hurtado M, Graham LC, Wishart TM, Gillingwater TH (2014) Label-free quantitative proteomic profiling identifies disruption of ubiquitin homeostasis as a key driver of Schwann cell defects in spinal muscular atrophy. J Proteome Res 13(11):4546–4557
Bogdanik LP, Sleigh JN, Tian C, Samuels ME, Bedard K, Seburn KL, Burgess RW (2013) Loss of the E3 ubiquitin ligase LRSAM1 sensitizes peripheral axons to degeneration in a mouse model of Charcot-Marie-Tooth disease. Dis Model Mech 6(3):780–792
Saifi GM, Szigeti K, Wiszniewski W, Shy ME, Krajewski K, Hausmanowa-Petrusewicz I, Kochanski A, Reeser S et al (2005) SIMPLE mutations in Charcot-Marie-Tooth disease and the potential role of its protein product in protein degradation. Hum Mutat 25(4):372–383
Ylikallio E, Poyhonen R, Zimon M, De Vriendt E, Hilander T, Paetau A, Jordanova A, Lonnqvist T et al (2013) Deficiency of the E3 ubiquitin ligase TRIM2 in early-onset axonal neuropathy. Hum Mol Genet 22(15):2975–2983
Lobsiger CS, Cleveland DW (2007) Glial cells as intrinsic components of non-cell-autonomous neurodegenerative disease. Nat Neurosci 10(11):1355–1360
Colomar A, Robitaille R (2004) Glial modulation of synaptic transmission at the neuromuscular junction. Glia 47(3):284–289
Rousse I, Robitaille R (2006) Calcium signaling in Schwann cells at synaptic and extra-synaptic sites: active glial modulation of neuronal activity. Glia 54(7):691–699
Hunter G, Aghamaleky Sarvestany A, Roche SL, Symes RC, Gillingwater TH (2014) SMN-dependent intrinsic defects in Schwann cells in mouse models of spinal muscular atrophy. Hum Mol Genet 23(9):2235–2250
Ilieva H, Polymenidou M, Cleveland DW (2009) Non-cell autonomous toxicity in neurodegenerative disorders: ALS and beyond. J Cell Biol 187(6):761–772
Hunter G, Powis RA, Jones RA, Groen EJ, Shorrock HK, Lane FM, Zheng Y, Sherman DL, Brophy PJ, Gillingwater TH (2016) Restoration of SMN in Schwann cells reverses myelination defects and improves neuromuscular function in spinal muscular atrophy. Hum Mol Genet
Erzen I, Cvetko E, Obreza S, Angaut-Petit D (2000) Fiber types in the mouse levator auris longus muscle: a convenient preparation to study muscle and nerve plasticity. J Neurosci Res 59(5):692–697
Ruiz R, Casanas JJ, Torres-Benito L, Cano R, Tabares L (2010) Altered intracellular Ca2+ homeostasis in nerve terminals of severe spinal muscular atrophy mice. J Neurosci 30(3):849–857
Ruiz R, Biea IA, Tabares L (2014) alpha-Synuclein A30P decreases neurodegeneration and increases synaptic vesicle release probability in CSPalpha-null mice. Neuropharmacology 76 Pt A:106–117
Ruiz R, Casanas JJ, Sudhof TC, Tabares L (2008) Cysteine string protein-alpha is essential for the high calcium sensitivity of exocytosis in a vertebrate synapse. Eur J Neurosci 27(12):3118–3131
Ruiz R, Tabares L (2014) Neurotransmitter release in motor nerve terminals of a mouse model of mild spinal muscular atrophy. J Anat 224(1):74–84
Ruiz R, Cano R, Casanas JJ, Gaffield MA, Betz WJ, Tabares L (2011) Active zones and the readily releasable pool of synaptic vesicles at the neuromuscular junction of the mouse. J Neurosci 31(6):2000–2008
Bradford MM (1976) A rapid and sensitive method for the quantitation of microgram quantities of protein utilizing the principle of protein-dye binding. Anal Biochem 72:248–254
Burguillos MA, Svensson M, Schulte T, Boza-Serrano A, Garcia-Quintanilla A, Kavanagh E, Santiago M, Viceconte N, Oliva-Martin MJ, Osman AM, Salomonsson E, Amar L, Persson A, Blomgren K, Achour A, Englund E, Leffler H, Venero JL, Joseph B, Deierborg T (2015) Microglia-secreted Galectin-3 acts as a toll-like receptor 4 ligand and contributes to microglial activation. Cell Rep
Ruiz R, Perez-Villegas EM, Bachiller S, Rosa JL, Armengol JA (2016) HERC 1 ubiquitin ligase mutation affects neocortical, CA3 hippocampal and spinal cord projection neurons: an ultrastructural study. Front Neuroanat 10:42
Schindelin J, Arganda-Carreras I, Frise E, Kaynig V, Longair M, Pietzsch T, Preibisch S, Rueden C et al (2012) Fiji: an open-source platform for biological-image analysis. Nat Methods 9(7):676–682
Murinson BB, Griffin JW (2004) C-fiber structure varies with location in peripheral nerve. J Neuropathol Exp Neurol 63(3):246–254
Orita S, Henry K, Mantuano E, Yamauchi K, De Corato A, Ishikawa T, Feltri ML, Wrabetz L et al (2013) Schwann cell LRP1 regulates remak bundle ultrastructure and axonal interactions to prevent neuropathic pain. J Neurosci 33(13):5590–5602
Arnold AS, Gill J, Christe M, Ruiz R, McGuirk S, St-Pierre J, Tabares L, Handschin C (2014) Morphological and functional remodelling of the neuromuscular junction by skeletal muscle PGC-1alpha. Nat Commun 5:3569
Datyner NB, Gage PW (1980) Phasic secretion of acetylcholine at a mammalian neuromuscular junction. J Physiol 303:299–314
Sabatini BL, Regehr WG (1996) Timing of neurotransmission at fast synapses in the mammalian brain. Nature 384(6605):170–172
Ludwin SK, Kosek JC, Eng LF (1976) The topographical distribution of S-100 and GFA proteins in the adult rat brain: an immunohistochemical study using horseradish peroxidase-labelled antibodies. J Comp Neurol 165(2):197–207
Griffin JW, Thompson WJ (2008) Biology and pathology of nonmyelinating Schwann cells. Glia 56(14):1518–1531
Scherer SS, Wrabetz L (2008) Molecular mechanisms of inherited demyelinating neuropathies. Glia 56(14):1578–1589
Trapp BD, Kidd GJ (2004) Structure of the myelinated axon, vol 1. Myelin biology and disorders. Elsevier, Amsterdam
Nagai J, Uchida H, Matsushita Y, Yano R, Ueda M, Niwa M, Aoki J, Chun J et al (2010) Autotaxin and lysophosphatidic acid1 receptor-mediated demyelination of dorsal root fibers by sciatic nerve injury and intrathecal lysophosphatidylcholine. Mol Pain 6:78
Chong-Kopera H, Inoki K, Li Y, Zhu T, Garcia-Gonzalo FR, Rosa JL, Guan KL (2006) TSC1 stabilizes TSC2 by inhibiting the interaction between TSC2 and the HERC1 ubiquitin ligase. J Biol Chem 281(13):8313–8316
Domenech-Estevez E, Baloui H, Meng X, Zhang Y, Deinhardt K, Dupree JL, Einheber S, Chrast R et al (2016) Akt regulates axon wrapping and myelin sheath thickness in the PNS. J Neurosci 36(16):4506–4521
Angaut-Petit D, McArdle JJ, Mallart A, Bournaud R, Pincon-Raymond M, Rieger F (1982) Electrophysiological and morphological studies of a motor nerve in 'motor endplate disease' of the mouse. Proc R Soc Lond B Biol Sci 215(1198):117–125
Edwards JP, Lee CC, Duchen LW (1994) The evolution of an experimental distal motor axonopathy. Physiological studies of changes in neuromuscular transmission caused by cycloleucine, an inhibitor of methionine adenosyltransferase. Brain 117(Pt 5):959–974
Feng Z, Koirala S, Ko CP (2005) Synapse-glia interactions at the vertebrate neuromuscular junction. Neuroscientist 11(5):503–513
Ko CP, Robitaille R (2015) Perisynaptic Schwann cells at the neuromuscular synapse: adaptable, multitasking glial cells. Cold Spring Harb Perspect Biol 7(10):a020503
Reddy LV, Koirala S, Sugiura Y, Herrera AA, Ko CP (2003) Glial cells maintain synaptic structure and function and promote development of the neuromuscular junction in vivo. Neuron 40(3):563–580
Fernandez-Chacon R, Wolfel M, Nishimune H, Tabares L, Schmitz F, Castellano-Munoz M, Rosenmund C, Montesinos ML et al (2004) The synaptic vesicle protein CSP alpha prevents presynaptic degeneration. Neuron 42(2):237–251
Patton BL, Chiu AY, Sanes JR (1998) Synaptic laminin prevents glial entry into the synaptic cleft. Nature 393(6686):698–701
Voigt T, Meyer K, Baum O, Schumperli D (2010) Ultrastructural changes in diaphragm neuromuscular junctions in a severe mouse model for spinal muscular atrophy and their prevention by bifunctional U7 snRNA correcting SMN2 splicing. Neuromuscul Disord 20(11):744–752
Mirsky R, Woodhoo A, Parkinson DB, Arthur-Farraj P, Bhaskaran A, Jessen KR (2008) Novel signals controlling embryonic Schwann cell development, myelination and dedifferentiation. J Peripher Nerv Syst 13(2):122–135
Herbert AL, Monk KR (2017) Advances in myelinating glial cell development. Curr Opin Neurobiol 42:53–60
Herskovitz S, Scelsa S, Schaumburg H. (2014) Electrodiagnostic, imaging, nerve, and skin biopsy investigation in peripheral nerve disease. Peripheral neuropathies in clinical practice. Oxford University Press, Oxford
Perez-Villegas EM, Negrete-Diaz JV, Porras-Garcia ME, Ruiz R, Carrion AM, Rodriguez-Moreno A, Armengol JA (2017) Mutation of the HERC 1 ubiquitin ligase impairs associative learning in the lateral amygdala. Mol Neurobiol
Adlkofer K, Frei R, Neuberg DH, Zielasek J, Toyka KV, Suter U (1997) Heterozygous peripheral myelin protein 22-deficient mice are affected by a progressive demyelinating tomaculous neuropathy. J Neurosci 17(12):4662–4671
Goebbels S, Oltrogge JH, Kemper R, Heilmann I, Bormuth I, Wolfer S, Wichert SP, Mobius W et al (2010) Elevated phosphatidylinositol 3,4,5-trisphosphate in glia triggers cell-autonomous membrane wrapping and myelination. J Neurosci 30(26):8953–8964
Goebbels S, Oltrogge JH, Wolfer S, Wieser GL, Nientiedt T, Pieper A, Ruhwedel T, Groszer M et al (2012) Genetic disruption of Pten in a novel mouse model of tomaculous neuropathy. EMBO Mol Med 4(6):486–499
Beirowski B, Wong KM, Babetto E, Milbrandt J (2017) mTORC1 promotes proliferation of immature Schwann cells and myelin growth of differentiated Schwann cells. Proc Natl Acad Sci U S A 114(21):E4261–E4270
Figlia G, Norrmen C, Pereira JA, Gerber D, Suter U (2017) Dual function of the PI3K-Akt-mTORC1 axis in myelination of the peripheral nervous system. elife 6
Maurel P, Salzer JL (2000) Axonal regulation of Schwann cell proliferation and survival and the initial events of myelination requires PI 3-kinase activity. J Neurosci 20(12):4635–4645
Ogata T, Iijima S, Hoshikawa S, Miura T, Yamamoto S, Oda H, Nakamura K, Tanaka S (2004) Opposing extracellular signal-regulated kinase and Akt pathways control Schwann cell myelination. J Neurosci 24(30):6724–6732
Bolis A, Coviello S, Bussini S, Dina G, Pardini C, Previtali SC, Malaguti M, Morana P et al (2005) Loss of Mtmr2 phosphatase in Schwann cells but not in motor neurons causes Charcot-Marie-Tooth type 4B1 neuropathy with myelin outfoldings. J Neurosci 25(37):8567–8577
Tersar K, Boentert M, Berger P, Bonneick S, Wessig C, Toyka KV, Young P, Suter U (2007) Mtmr13/Sbf2-deficient mice: an animal model for CMT4B2. Hum Mol Genet 16(24):2991–3001
Golan N, Kartvelishvily E, Spiegel I, Salomon D, Sabanay H, Rechav K, Vainshtein A, Frechter S et al (2013) Genetic deletion of Cadm4 results in myelin abnormalities resembling Charcot-Marie-Tooth neuropathy. J Neurosci 33(27):10950–10961
Lee SM, Sha D, Mohammed AA, Asress S, Glass JD, Chin LS, Li L (2013) Motor and sensory neuropathy due to myelin infolding and paranodal damage in a transgenic mouse model of Charcot-Marie-Tooth disease type 1C. Hum Mol Genet 22(9):1755–1770
Adlkofer K, Martini R, Aguzzi A, Zielasek J, Toyka KV, Suter U (1995) Hypermyelination and demyelinating peripheral neuropathy in Pmp22-deficient mice. Nat Genet 11(3):274–280
Murakami T, Garcia CA, Reiter LT, Lupski JR (1996) Charcot-Marie-Tooth disease and related inherited neuropathies. Medicine (Baltimore) 75(5):233–250
Pareyson D (1999) Charcot-marie-tooth disease and related neuropathies: molecular basis for distinction and diagnosis. Muscle Nerve 22(11):1498–1509
Aggarwal S, Bhowmik AD, Ramprasad VL, Murugan S, Dalal A (2016) A splice site mutation in HERC1 leads to syndromic intellectual disability with macrocephaly and facial dysmorphism: Further delineation of the phenotypic spectrum. Am J Med Genet A 170(7):1868–1873
Nguyen LS, Schneider T, Rio M, Moutton S, Siquier-Pernet K, Verny F, Boddaert N, Desguerre I et al (2016) A nonsense variant in HERC1 is associated with intellectual disability, megalencephaly, thick corpus callosum and cerebellar atrophy. Eur J Hum Genet 24(3):455–458
Ortega-Recalde O, Beltran OI, Galvez JM, Palma-Montero A, Restrepo CM, Mateus HE, Laissue P (2015) Biallelic HERC1 mutations in a syndromic form of overgrowth and intellectual disability. Clin Genet 88(4):e1–e3
Utine GE, Taskiran EZ, Kosukcu C, Karaosmanoglu B, Guleray N, Dogan OA, Kiper PO, Boduroglu K, Alikasifoglu M (2017) HERC1 mutations in idiopathic intellectual disability. Eur J Med Genet
Acknowledgements
This work was supported by grants to AMC and RR from the Fundación Ramón Areces—Spain—and DGICYT—Spain (Departamento Gubernamental de Investigaciones Científicas y Tecnológicas: BFU2011-27207); RR [Juan de la Cierva contract JCI-2011-08888 from the Ministerio de Economía y Competitividad (MINECO) and VPPI-US from the University of Seville]; JLV and RRL (MINECO: SAF2015-64171-R); JAA (Spanish Junta de Andalucía BIO-122 and DGICYT BFU2015-64536-R), and SB was supported by the Fundación Ramón Areces fellowships; LMR is the recipient of a grant from the Servicio Andaluz de Salud de la Junta de Andalucía (C-0009-2015). We are grateful to Juan Luis Ribas, Cristina Vaquero, and Asunción Fernández for their helpful technical supports (CITIUS, University of Seville) and to Drs. Manuel Sarmiento and Karin Neukam for their helpful comments and for editorial assistance.
Author information
Authors and Affiliations
Corresponding author
Ethics declarations
Conflict of Interest
The authors declare that they have no conflict of interests.
Electronic Supplementary Material
Supplementary Figure 1
In transverse sections of the Ranvier node, the aspect of Schwann cells’ microvilli surrounding the nodal membrane (A) (see [41]) was substituted in tbl axons by irregular concentric myelin figs. (B, arrow). Electron microphotographs of transverse sections of tbl sciatic nerve showed: Increased periaxonal space (C, arrow) and anomalous inner mesaxon (D, arrows) together to tomacula of different sizes (E, asterisks). Less affected tbl small and medium size myelinated axons do not show great signs of hypermyelination (H-I) as compared with control axons (F-G) of the same diameter. The number of axons (J) and the percentage (K) per Remak fiber are higher in tbl mice. Scale bars: 5 μm (B), 2 μm (A, E), 1 μm (C, D), 0.5 μm (F, H), and 0.2 μm (G, I). **P < 0.005; ***P < 0.0005. (GIF 700 kb)
Rights and permissions
About this article
Cite this article
Bachiller, S., Roca-Ceballos, M.A., García-Domínguez, I. et al. HERC1 Ubiquitin Ligase Is Required for Normal Axonal Myelination in the Peripheral Nervous System. Mol Neurobiol 55, 8856–8868 (2018). https://doi.org/10.1007/s12035-018-1021-0
Received:
Accepted:
Published:
Issue Date:
DOI: https://doi.org/10.1007/s12035-018-1021-0