Abstract
This paper offers a broad and critical overview of current discussions on the potential uses and the characterization of pigments in prehistory, with a special focus on prehistoric rock art. Today, analytical approaches to pigments and paints allow us to go beyond the identification of the elemental and molecular composition of these archaeological remains, to explore also raw material procurement, transformation and use strategies of interest to investigate the technological and socio-cultural practices of prehistoric artists and their change over space and time. The paper also summarizes the palette of prehistoric artists, as well as the techniques and analytical strategies used to date to characterize prehistoric pigments and paints (colours, raw materials, binders and recipes) used in prehistoric rock art.
Similar content being viewed by others
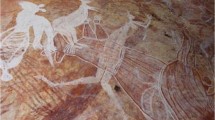
Avoid common mistakes on your manuscript.
Premise
This Topical Collection (TC) covers several topics in the field of study, in which ancient architecture, art history, archaeology and material analyses intersect. The chosen perspective is that of a multidisciplinary scenario, capable of combining, integrating and solving the research issues raised by the study of mortars, plasters and pigments (Gliozzo et al. 2021). The first group of contributions explains how mortars have been made and used through the ages (Arizzi and Cultrone 2021; Ergenç et al. 2021; Lancaster 2021; Vitti 2021). An insight into their production, transport and on-site organization is further provided by DeLaine (2021). Furthermore, several issues concerning the degradation and conservation of mortars and plasters are addressed from practical and technical standpoints (La Russa and Ruffolo 2021; Caroselli et al. 2021).The second group of contributions is focused on pigments, starting from a philological essay on terminology (Becker 2021). Three archaeological reviews on prehistoric (this paper), Roman (Salvadori and Sbrolli 2021) and Mediaeval (Murat 2021) wall paintings clarify the archaeological and historical/cultural framework. A series of archaeometric reviews illustrate the state of the art of the studies carried out on Fe-based red, yellow and brown ochres (Mastrotheodoros et al. forthcoming); Cu-based greens and blues (Švarcová et al. 2021); As-based yellows and reds (Gliozzo and Burgio 2021); Pb-based whites, reds, yellows and oranges (Gliozzo and Ionescu 2021); Hg-based red and white (Gliozzo 2021) and organic pigments (Aceto 2021). An overview of the use of inks, pigments and dyes in manuscripts; their scientific examination and analysis protocol (Burgio 2021) and an overview of glass-based pigments (Cavallo and Riccardi forthcoming) are also presented. Furthermore, two papers on cosmetic (Pérez Arantegui 2021) and bioactive (antibacterial) pigments (Knapp et al. 2021) provide insights into the variety and different uses of these materials.
Introduction
Archaeological research has provided evidence of human use of natural minerals and earths with colouring properties (iron and manganese oxides and hydroxides including haematite, goethite, maghemite, siderite and other minerals such as kaolinite, huntite, white chalk and others), with some examples dating back thousands of years (Watts et al. 2016; Wolf et al. 2018). The presence of pieces of pigment of various sizes, pigment powders and residues and even drops of paint in archaeological deposits, contexts or materials (shells, stone and bone tools, grinding stones, different sorts of containers and so forth) is well attested, even before the appearance of rock art or the global dispersal of anatomical modern humans.
The identification of ochre processing workshops (including raw materials, processing tools and/or storage containers) in different chronologies and places—e.g. Blombos cave in South Africa 100,000 years ago (Henshilwood et al. 2011), Qafzeh Cave in Israel around 92,000 years ago (Hovers et al. 2003), Porc-Epic Cave in Ethiopia ca. 40 ka cal BP (Rosso et al. 2014), Santa Maira in Spain between 15 and 6 ka cal BP (Tortosa et al. 2020), Cova de l’Or in Spain in early Neolithic deposits (Domingo et al. 2012), the Early-Middle Holocene site of Takarkori, in Lybia (di Lernia et al. 2016), among many others—reveals that the use of colouring raw materials was not accidental but a well-planned activity since prehistoric times, demanding an important investment of time and effort to source and process these materials.
In the last few decades, the combination of multi-technical (different non-invasive and invasive analytical techniques, use-wear analysis, experimental archaeology, etc.) and multidisciplinary approaches (chemistry, archaeology, geology, traceology, ethnography and so forth) is providing a more comprehensive view of these raw materials beyond the identification of their nature and composition, as we will discuss along with this paper. With the new approaches, we are now able to explore also potential sources, processing practices and uses informing on ancient pigment sourcing, procurement (whether autonomous or obtained through exchange networks) and transformation strategies and technologies, and their changes over time. We can also explore if the raw materials were used directly on soft or hard surfaces (e.g. as crayons) or transformed into powder through grinding and scraping and if any additives or binders were added to produce a paste or a paint.
Our aim with this paper is to offer a broad overview of the techniques and analytical strategies used so far to characterize prehistoric pigments and the raw materials, binders and recipes identified to date in rock art. A full review of the global literature dealing with pigment analysis of prehistoric rock art is beyond the scope of this paper since in the last couple of decades the number of papers addressing this topic has considerably increased across the world. Thus, while we reference global literature when offering examples, we specially selected those from Western and Mediterranean European prehistory and rock art to illustrate our discussions, as it is our main study area.
The many uses of pigments in prehistoric times
We know today that the presence of pigments in archaeological deposits is not only related to the artistic production since many of these raw materials have other preservative, antiseptic, abrasive, repellent, protective or even medical properties (Velo, 1984; Hovers et al. 2003; García-Borja et al. 2004; Soressi and d’Errico, 2007; Roebroeks et al. 2012; Rifkin et al. 2015, among many others), which were very probably known in prehistoric times.
As early as the 1980s, Couraud (1988) proposed already three different uses of colouring raw materials during the European Palaeolithic, which could be also extended to other regions and periods:
-
The production of different forms of artistic expression
-
Domestic and functional uses
-
Funerary practices
The archaeological literature has often related the use of these materials to different sorts of artistic, aesthetic or symbolic practices. These include different sorts of deliberate marks, drawings and paintings produced on rock walls both within caves and rock shelters as well as in the open air and on different sorts of portable objects. For a long time, this kind of artistic production seemed to be mainly related to anatomical modern humans, with the earliest examples including several fragmented ochreous pebbles and crayons with intentional incisions located at different South African sites (Blombos cave, Klasies River, Pinnacle Point 13B, Klein Kliphuis) and dating back between 100,000 and 85,000 years ago (Henshilwood et al. 2009; D’Errico et al. 2012); 73,000-year-old red crosshatch marks identified on a rock fragment at Blombos Cave (Henshilwood et al. 2018); 77,000-year-old shell beads decorated with ochre at Blombos Cave, Sibudu and Border Cave (Henshilwood et al. 2004) and other African sites or the earliest examples of figurative and non-figurative painted rock art produced by anatomical modern humans (AMH) in Spain (Pike et al. 2012; Hoffman et al. 2018a) and Indonesia (Aubert et al. 2014, 2018; Brum et al. 2021) dating back at least 40 and 45,000 years respectively. From then on, the tradition of using colouring raw materials to produce different sorts of art on different media (stone, bone, antler, pottery and so forth) spread around the world at different points in time.
Today, a central debate in research on archaeological pigments and human evolution is if their use to produce different sorts of visual arts and marks on different media was exclusive to us (AMH) or there are any precedents in the human lineage. In the last decade, several red rock art marks and paintings located at different Spanish sites (some mouth or airbrush blown red paint located over a stalagmitic dome at Ardales site, Málaga, a hand stencil at Maltravieso, Cáceres, and a scalariform sign at La Pasiega, Cantabria) have been dated as early as, at least, 64 to 66,000 years ago (Hoffman et al. 2018a, Zilhão 2020). At that time, AMH had not yet arrived in the Iberian Peninsula, thus suggesting that these depictions were produced by Eurasian Neanderthals. Neanderthals’ use of personal ornaments with red colouring residues as well as the use of colouring materials has been also reported by several researchers. For example, at Cueva de los Aviones (Murcia, Spain) Zilhão et al. (2010) interpreted a mixture of charcoal, dolomite, haematite and pyrite as well as yellow natrojarosite associated with marine shells dating back 115,000 years (Hoffman et al. 2018b), as potentially used for cosmetic purposes, since according to them, the only known archaeological use of natrojarosite is in cosmetics. Along the same lines, Soressi and d’Errico (2007) suggest that the manganese dioxide from the French Mousterian sites of Pech de l’Azé I and Pech de l’Azé IV may have been used for body painting (Soressi and d’Errico 2007). Drops of a haematite-rich liquid of unknown use were also identified on the sediment at Maastricht-Belvédère deposits in the Netherlands pushing back in time the use of this mineral by early Neanderthals to at least 200–250 ka (Roebroeks et al. 2012). Thus, long before the arrival of AMH to Eurasia, Neanderthals were already using mineral colouring materials for different purposes. Whether this use of different pigments was symbolic or practical is difficult to know since (as already discussed) many of these colouring raw materials had many properties and as such, they were well suited for other daily activities like hide tanning, tool hafting, tool polishing and so forth (Hovers et al. 2003).
The use of different colouring raw materials for practical purposes in prehistoric times has been also deduced based on a mixture of archaeological, ethnographic and experimental observations. Some practical applications proposed so far include their use as additives mixed with natural glues to be used as a fixative for hafting tools (Beyries and Inizan 1982; Allain and Rigaud 1989; Wadley 2005; Lombard 2007; Helwig et al. 2014), as natural abrasives for fine polishing of bone tools and ornaments (Goñi et al. 1999; García Borja et al. 2004), as a natural antiseptic for tanning hides to reverse the decay process (Audion and Plisson 1982; Adams 1988; Rifkin 2011), to dry tendons for different purposes (such us bow strings or for attaching stone tools to the shafts), etc. (Domingo et al. 2012).
Colouring materials have been also reported in funerary contexts from the Middle Palaeolithic to the Neolithic and beyond (for example, Formicota 1986; Molleson et al. 1992; Cacho et al. 1996; Stringer and Gamble 1996; Bar-Yosef 1997; Goren et al. 2001; d’Errico and Backwell 2016). In these contexts, they appeared as lumps of different sizes or powder remains identified in the deposits or within different sorts of containers and tools (offerings?), as well as in the form of body or clothing decorations (e.g. Schotsmans et al. 2020), or even covering human skeletal remains. Couraud suggested that some of these funerary practices could aim at facilitating the preservation of the human remains since ochre helps slow down decomposition (Couraud 1988). A similar interpretation is provided by other researchers (Delibes 2000; Blas 2003; Martín-Gil et al. 2004) when the mineral used in these funerary contexts was cinnabar. Other authors, though, see a more symbolic explanation when ochre is recorded in relation to human skeletal remains (Hovers et al. 2003). Interestingly, ethnographic accounts suggest that the boundaries between symbolic and practical domains are often blurred and both can coexist in the same object, tradition or practice (Rosso 2017; Domingo et al. 2020). Nevertheless, symbolic uses are usually culturally constructed and archaeologically invisible since they leave behind no empirical evidence, and thus, they are hard to demonstrate. Based on the different uses identified in funerary contexts of Çatalhöyük, Schotsmans et al. (2020) suggest that pigments were probably used to build identities as well as for social differentiation. For example, in funerary contexts, most of the inhabitants of this site did not receive pigment treatment, and the very few who did point to different treatment depending on their sex (cinnabar for male and blue and green pigments for female).
In the last few decades, interdisciplinary approaches to the archaeology of colour have been also applied to the characterization of pigments used for rock art production in prehistoric times, which are the focus of our research (Domingo et al. 2021). This particular topic faces more challenges than the study of other archaeological pigments since rock art sampling entails compromising the integrity of ancient works of art often protected and listed in the different National Heritage acts (e.g. in Spain rock art is protected with the highest level of legal protection—Asset of Cultural Interest—according to the 1985 Spanish Heritage Act, and as such, it cannot be destroyed or removed). Some are even listed in the UNESCO World Heritage list (Fig. 1). So even if today we are talking about microsamples, rock art is considered an irreplaceable asset and it entails (as it should be) more restrictions when it comes to invasive sampling.
Map with rock art sites and concentrations of sites included in the UNESCO World Heritage list. 1. Writing-on-Stone Áisinai’pi (Canada). 2. Mesa Verde National Park (United States). 3. Sierra de San Francisco (Mexico). 4. Chiribiquete National Park (Colombia). 5. Nasca and Palpa (Perú). 6. Serra da Capivara (Brasil). 7. Ischigualasto/Talampaya Natural Park (Argentina). 8. Cueva de las Manos (Argentina). 9. Risco Caído and the Sacred Mountains of Gran Canaria Cultural Ladscape (Spain). 10. Valle do Côa (Portugal) and Siega Verde (Spain). 11. Bend of Boyne (Ireland). 12. Altamira and Palaeolithic cave art of Northern Spain. 13. Rock art of the Mediterranean Basin on the Iberian Peninsula. 14. Vézère Valley (France). 15. Grotte Chauvet-Pont d’Arc (France). 16. Valcamonica (Italy). 17. Tanum (Sweden). 18. Rock Art of Alta (Norway). 19. Tassili n’Ajjer (Algeria). 20. Tadrart Acacus (Libya). 21. Ennedi Massif natural and cultural landscape (Chad). 22. Ecosystem and Relict Cultural Landscape of Lopé-Okanda (Gabon). 23. Kondoa (Tanzania). 24. Twyfelfontein o/Ui-//aes (Namibia). 25. Tsodilo (Botswana). 26. Chongoni (Malawi). 27. Matobo Hill (Zimbabwe). 28. Maloti Drakensberg (South Africa). 29. Wadi rum Protected area (Jordan). 30. Hail Region (Saudi Arabia). 31. Gobustan (Azerbaijan). 32. Tamgaly (Kazakhstan). 33. Sulaiman-Too Sacred Mountains (Kyrgyzstan). 34. Bhimbetka (India). 35. Mongolian Altai (Mongolia). 36. Zuojiang Huashan rock art (China). 37. Rock Islands Southern Lagoon (Republic of Palau). 38. Kakadu National Park (Australia). 39. Uluru-Kata Tjuta (Australia)
In this part of the world, prehistoric art appears from Norway to Spain and covers more than 60,000 years (Hoffman et al. 2018a), spanning from the Middle Palaeolithic to the Bronze Age. It includes several forms of non-figurative and figurative art produced on different media (stone, bone, antler, ivory, animal teeth and maybe some perishable materials such as wood) and using a variety of techniques (paintings, engravings, carvings). In such a vast territory, different traditions developed over time, including among others Palaeolithic, Levantine and Schematic art. Palaeolithic art is an art of naturalistic animals and signs with singular sites in Iberia and France like Altamira, Chauvet, Lascaux, Foz Côa, to name a few) dating between 40,000 and 11,700 cal. BP. Levantine rock art is an art of naturalistic humans and animals illustrating narratives of hunting, war and violence, maternity and death. This art is specific to Mediterranean Iberia, with outstanding sites like Cova dels Cavalls, Remigía, la Sarga or Cogul. It dates sometime around 7500 years ago (no reliable numerical dates are known yet) (Ochoa et al. 2021). Schematic art is an art of schematic humans, animals and geometric shapes, with different traditions across Europe dating between the Early Neolithic and the Bronze Age (for a global overview of European prehistoric art, see Sauvet et al. 2014).
Characterizing prehistoric pigments
Over the last three decades, archaeometric approaches have highly increased our knowledge of prehistoric pigments used both in caves and in open-air rock shelters and boulders worldwide to create the first masterpieces of the history of art (Salomon et al. 2008; Menu 2009; de Balbín Behrmann and Alcolea González 2009; Aubert et al. 2014; Chalmin and Huntley 2017; Stuart and Thomas 2017; Domingo et al. 2021; Gallinaro and Zerboni 2021; Sepúlveda 2021). The identification of the archaeological pigments and their sources (e.g. Pitarch et al. 2014 and 2019; Defrasne et al. 2019), the examination of the manufacturing techniques and pictorial recipes (e.g. Brunet et al., 1982; Menu and Walter 1992, 1996; Baffier et al. 1999; Salomon et al. 2008; Menu 2009) or understanding the mechanisms causing paint and bedrock degradation (Hernanz et al. 2007; Aramendia et al. 2020) are some of the common archaeological questions that can be effectively unravelled through physicochemical analyses. Beyond the more traditional dating purposes (Steelman and Rowe 2012; Ochoa et al. 2021), analytically studying rock paintings, we can gain information about the technological and socio-cultural practices and backgrounds of prehistoric artists and, at the same time, acquire knowledge about the conservation of these particular artistic expressions often located in changing environments. Indeed, accurate diagnostic analysis of rock art productions can provide insight into pigments’ raw materials and their provenance, offering precious information on the geographic practices and the social networks of prehistoric artists and their counterparts (Chalmin et al. 2006; Iriarte et al. 2009; Jezequel et al. 2011; Mas et al. 2013; Pitarch et al. 2014), as well as into painters’ manufacturing methods for pigment processing, such as grinding, heating, sieving and settling, and pigment application techniques (Menu and Walter 1992; Salomon et al. 2008; Bonneau et al. 2012; Salomon et al. 2015, Hernanz et al. 2008; López et al. 2017; Cuenca-Solana et al. 2016; d’Errico et a. 2016; Gay et al. 2020). Through these sorts of approaches, we obtain information to reconstruct the different steps of the chaîne operatoire (operative sequence) required to produce an artwork, the behaviours, acts and skills of ancient artists and their changes over space and time, of interest to identify cultural differences, similarities and interactions (Jezequiel et al. 2011; Bonneau et al. 2012; Pitarch et al. 2014; Velliky et al. 2020; Tortosa et al. 2020). Moreover, the physicochemical analyses of the rock paintings are also important to characterize any alteration mechanisms threatening the preservation of the paintings, due to natural aging and weathering or to anthropic direct or indirect interventions. Such an approach is necessary to develop the best conservation strategies and practices adapted to the particular features of the artworks and the environmental specificities of their contexts. Distinguishing between pictorial layers, the rock supports and any interstratified/external patinas and crusts is decisive for understanding weathering processes and the preservation of the rock art paints (Doehnne and Price 2010; Dorn et al. 2008).
Overall, multidisciplinary research based on analytical approaches applied to the study of prehistoric rock art has changed the way we understand the materials, tools and procedures involved in rock paintings production. In the past few decades, we have moved from the more purely stylistic and descriptive studies of the art to the objective examination of the chemical and physical properties of the materials constituting the pictograms. In this way, the universal language of science, integrated with aesthetical evaluations, is now a better integral means to distinguish, analyze, understand, compare and describe prehistoric rock paintings. It is worth mentioning that scientific examinations alone are not enough to offer complete answers to archaeological research questions, but they need to be opportunely included in a larger archaeological research context using a multidisciplinary and collaborative approach among the different disciplines. A crucial aspect related to the study of rock art pigments and paintings, and more in general to the examination of a wide range of cultural heritage objects, is the increasing awareness that there is not known technique that—by itself—could answer all of the research questions raised by any scientific research (Pollard et al. 2007; Madariaga 2015; Hernanz and Gavira-Vallejo 2021). As such, the analysis of rock art pigments requires a combination of several analytical methods to provide a complete characterization of the materials within their environment in terms of physical, chemical, optical, structural, geological and morphological properties. The integration of data from different analytical techniques represents the best strategy to overcome the intrinsic limitation of every single method when applied to the study of heterogeneous matrices, such as those usually found in rock art, often multilayered and complex due to overlapping of pictorial layers, patinas, crusts and bedrock media characterized by different materials. Moreover, considering the enormous value of these heritage assets, usually appearing in remote locations, portable non-invasive analytical methods and non-destructive techniques when analyzing micro-samples are usually required for the examination of these valuable artworks. According to the literature and our own experience, pigment analyses of rock art are generally performed in progressive smaller scales, from macro-, micro- to nano-size (Fig. 2). Their chemical characterization is usually made by elemental and molecular spectroscopic techniques such as X-ray fluorescence spectroscopy (XRF) and Raman spectroscopy. In addition, to determine their morphology and crystalline structure, other techniques like optical microscopy (OM), transmission electron microscopy (TEM), scanning electron microscopy (SEM), typically combined with energy-dispersive X-ray spectroscopy to perform elemental micro-analyses (SEM–EDX) and X-ray diffraction (XRD) have been also used. A similar analytical approach can be also applied to the study of prehistoric portable art. A complete description of the commonest laboratory and portable analytical methods used in archaeological and cultural heritage research can be found in Pollard et al. (2008), Nigra et al. (2015), Madariagara (2015), Vandenabeele and Donais (2016), Bersani and Lottici (2016), Brunetti et al. (2016), while their specific application to the characterization of prehistoric rock art pigments worldwide can be obtained in Vignaud et al. (2006), Menu (2009), Aubert et al. (2014), Chalmin and Huntley (2017), Stuart and Thomas (2017), Sepúlveda (2021), Gallinaro and Zerboni (2021), Domingo et al. (2021), Hernanz and Gavira-Vallejo (2021), to name a few. It is worth mentioning that the analytical approaches and the scientific methods used in the study of rock art pigments have changed over the last 30 years, following and adapting to the contemporary technological advances that occurred in the field of portable spectroscopic analytical instruments. In fact, the first extensive and systematic physicochemical studies of rock art pigments started at the beginning of the 1990s and they were performed exclusively on micro-samples, as occurred in the substantial research regarding European Palaeolithic cave art. Several minute samples coming from important French and northern Spanish painted caves such as Altamira, Niaux, Lascaux, Ekain and La Garma were analytically studied at the laboratories of Centre de Recherche et de Restauration des Musées de France, C2RMF, in Paris (Clottes et al. 1990; Menu 1990; Menu and Walter, 1992; Clottes 1997; Chalmin et al. 2003; Vignaud et al. 2006; Chalmin et al. 2017). These precious research works shed light on the nature of the pigments employed by Palaeolithic artists and they brought to light elaborated pictorial recipes and manufacturing methods, displaying remarkable technical skills of the prehistoric painters. Afterwards, in the last few years, several technological efforts have been spent in the miniaturization of optical and electronic components of portable spectroscopic instruments trying to balance their performance with that of their respective bench top laboratory setups. These advances have led to the creation of more and more compact, light and smart spectroscopic devices with high performance for in situ non-invasive spectroscopic investigations, especially suited for the analyses of cultural heritage objects (Vandenabeele and Donais 2016; Brunetti et al. 2016). Such progresses in the improvement of portable and handheld systems for in-field non-invasive analysis has facilitated the access to valuable and immovable artworks non-suitable for in-lab analysis, like prehistoric rock art, thus enabling a growing number of studies to be conducted in situ, contributing to preserving the integrity of the artworks (e.g. Roldán et al. 2010; Lahlil et al. 2012; Beck et al. 2014; Olivares et al. 2013; Gay et al. 2015, 2016, 2020; Pitarch et al. 2014).
A Portable energy-dispersive X-ray fluorescence (EDXRF) spectrometer designed by ICMUV employed for non-invasive on-site analyses of Schematic and Levantine paintings at el Carche site (Jalance, Spain). B Schematic red animal from el Carche site (Jalance, Spain) indicating the sampled area. To minimize visual impact, the sample was taken next to an area with painting loss. C Optical microscope image of a micro-fragment sampled from a red Schematic painted figure showed in B. Three different layers can be recognized, from bottom to top: the bedrock, the red painting layer, and the orangey external patinas
A preliminary non-invasive diagnostic analytical campaign represents the roots on which to base further analyses in terms of (i) identification of the constituting materials, (ii) selection of points or areas to be sampled at a later stage, (iii) evaluation of the state of conservation of the paintings within their environment, and their monitoring over time, and (iv) planning of any conservation and/or restoration intervention (Fig. 2A). Sampling is still an essential step to advance knowledge on the study of rock art, as it is required to conduct microstratigraphic studies, dating, etc. (Fig. 2B-C). However, if prior to sampling, we conduct in situ non-invasive analyses, we will minimize impacts and obtain information to guide future sampling. Unlike sampling, non-invasive approaches allow the potential examination of the whole painting, offering a more global view of the art under study. Thus, non-invasive analyses are essential to design ethically targeted sampling strategies aimed at analyzing those areas with the potential to provide the most interesting results (Beck et al. 2013). On the contrary, a micro-sample can be representative only of the site or the sampled figure. Considering the fragility and the values of the rock paintings, the number of micro-samples should be reduced to a minimum, to answer questions that can only be answered by sampling. It is now generally accepted that a proper research protocol in the study of an artwork, rock art included, involves as a first step, when feasible, the use of non-invasive analytical approaches and the synergistic use of multi-technical and multi-scale analyses. When talking about rock art, it is important to note that the logistical organization of a non-invasive research campaign is not a trivial matter: the geographical location of the rock shelters could represent a real challenge in terms of physical access, energy source and portability of the instruments. These logistical challenges could force us to prioritize direct sampling, assisted only by small portable digital microscopy and/or digital cameras when choosing the area to be sampled, rather than being guided by preliminary scientific analyses that could have helped us formulate new research questions. This may unnecessarily increase the number of samples needed, resulting in more invasive interventions and hence causing a more negative impact on this fragile heritage. Thus, a non-invasive spectroscopic analytical campaign should be always prioritized, when feasibly. Among the portable non-invasive spectroscopic methods available, portable energy-dispersive X-ray fluorescence (Fig. 2A) and Raman spectroscopies have been extensively used in rock art research for elemental and molecular in situ analyses, respectively (e.g. Roldan et al. 2010; Hernanz 2015; Gay et al. 2016). However, more complex portable devices have been brought to the field, as the portable system combining X-ray fluorescence and diffraction techniques used for the first time to analyze the pigments at Rouffignac Cave (Dordogne, France). This was the first XRF-XRD experiment ever undertaken in an underground and archaeological environment to study prehistoric rock art (Beck et al. 2014). These types of portable instruments are usually employed to analyze artworks located in indoor environments, like a museum, since they are too large and bulky to be brought into the field (Vandenabeele and Donais 2016). Vis–NIR hyperspectral imaging technology, a novel method in the field of rock art studies so far, has been employed for the first time in the analyses of rock art materials and pigments from the Palaeolithic Cave of El Castillo (Puente Viesgo, Cantabria, Spain) (Bayarri et al. 2019). This technique is a powerful 2D spectroscopic diagnostic technology for the non-invasive analyses of polychromatic surfaces of artworks highly employed in the study of easel paintings (Cucci et al. 2016). This imaging method provides a non-invasive way to identify colour materials and maps them on the surface, representing a promising research tool also to study, preserve and manage this valuable cultural heritage.
The palette of prehistoric artists
Despite different chronological periods, artistic traditions, cultures and geographic locations, inorganic pigments from mineral origins usually characterize the palette of prehistoric artists. Very broadly speaking, the palette of prehistoric artists is generally composed of two main hues, namely red and black. Other colours such as yellow and white were less frequently used (Fig. 3). In addition, the use of charcoal from the organic origin (wood charcoal and burnt bone) has been also broadly detected. In the following lines, a general description of the most common pigments used in the production of prehistoric rock art will be outlined, describing their chemical nature and characteristics, and drawing attention to some selected examples.
Red pigments
Iron-based oxides and hydroxides compose red pigments, among which haematite (α-Fe2O3) is the most frequently identified in the Palaeolithic and post-Palaeolithic rock art record all over the world (Chalmin et al. 2003; Vignaud et al. 2006; Salomon et al. 2008; Menu 2009; de Balbín Behrmann and Alcolea González 2009; Chalmin and Huntley 2017; Aubert et al. 2014; Stuart and Thomas 2017; Gallinaro and Zerboni 2021; Domingo et al. 2021; Sepúlveda 2021, among others). Haematite is a Fe (III) oxide highly widespread in rocks and soils (Fig. 4), which colour depends on its crystal size and shape: the tones range from yellowish/red for nanoparticles to purple for micrometer sized ones or even black for larger crystals, like specularite (Cornell and Schwertmann, 2003). The mineral haematite is also the principal colouring matter constituting red ochres. Focusing a bit on some definitions, ochres are naturally occurring deposits characterized by a variable amount of clayey minerals (such as kaolinite, illite), iron oxides/hydroxides and other minerals/impurities (like quartz, calcium carbonates, etc.), showing different colours from brown to red through yellows and violets, depending on the specific nature of Fe oxides/hydroxides present (Siddal 2018) (Fig. 5). Thus, ochres comprise a wide range of raw materials from geological formations bearing iron oxides/hydroxides including shales, mudstones, siltstones, earthy sandstones and other types of ferruginous deposits (e.g. ferricrete and limonite ores). Their specific red or yellow hues, and their consequent definition as red/yellow ochres, are due to the presence of haematite (α-Fe2O3) and goethite (α-FeOOH), respectively, as main chromophores among the other ochres’ constitutive components. Ochres belong to the wider group of earth pigments, defined as natural deposits rich in clay minerals, iron oxides, manganese oxides and other minerals that produce a coloured fine powder with high tinting strength (Eastaugh et al. 2008). As a pictorial pigment, thanks to its natural abundance and chemical stability, haematite-based materials have been widely used in art from Pleistocene times—with world-renowned sites like the western European Palaeolithic sites of Lascaux (Dordogne, France) or Altamira (Spain)—to the present by all cultures of the world (Eastaugh et al. 2008). Focusing on Western and Mediterranean European studies, haematite and red ochres have been the main components of all prehistoric red pictograms, from Palaeolithic rock art, known for the richness of pictorial recipes (Clottes et al. 1990; Chalmin et al. 2003, 2004a; de Balbín Behrmann and Alcolea González 2009; Iriarte et al. 2009; Menu 2009; Hernanz et. al 2011; Jezequiel et al. 2011; Olivares et al. 2013) to the subsequent post-Palaeolithic traditions such as Levantine rock art (specific to Mediterranean Spain) and the more recent Schematic rock art (Rosina et al. 2018; Domingo et al. 2021).
Optical microscope image of an ochre powder sampled from the soil of Coves Llongues site (Castellón, Spain). The image shows the heterogeneous composition and texture of the powder characterized by crystals of different colours and sizes. From micro-Raman analyses (data not shown), this ochre is mainly composed of calcite, gypsum, haematite and magnetite
Although haematite and haematite-rich earths occur naturally, heat treatment or mechanical processes inducing the dehydration of goethite can synthesize it (Cornell and Schwertmann, 2003; González et al. 2000). Specifically to the heating procedures, yellow goethite, or high goethite content materials like yellow ochre, turns its yellowish colour to red shades upon heating at temperatures above 250–300 °C, forming disordered haematite whose complete crystallization happens at higher temperatures (> 850 °C), due to a topotactic transformation that involves the loss of water by the iron (III) hydroxide. For archaeologists, understanding the pigment production methods is an indirect way to assess the level of technological and cognitive development of prehistoric communities. In this sense, and in the field of archaeology, several research efforts initiated by Marie Pierre Pomies and coworkers have been successfully performed over the years to analytically distinguish haematite synthesized by heating from natural haematite (Pomiès et al. 1998 and 1999; Gialannella et al. 2011; Salomon et al. 2012, 2015; Cavallo et al. 2018). In particular, thanks to the complementary use of X-ray diffraction (XRD) and transmission electron microscopy (TEM) microanalyses, integrated with other complementary analytical methods, and the study of non/archaeological samples, nonuniform broadening of diffraction peaks and residual dehydration pores of single crystals can be considered as main indicators of previous heating. Moreover, when the archaeological and geological contexts are well known, this research protocol can also distinguish between a deliberate, and perhaps perfectly mastered, heating and an accidental one (Salomon et al. 2012, 2015). This knowledge is extremely important for the study and understanding of prehistoric pigments, including those used to create rock art. In fact, for many years, heating has been assumed the motive for the high frequencies of red rocks used in archaeological campsites and the red pigments in rock art paintings from Western Europe (Salomon et al. 2015). Thus, this research protocol has been effectively adopted to examine prehistoric pigments from different sites, contexts and archaeological levels. As an example, and contrary to what it was previously thought, several studies showed that no heat treatment had been applied to the red pigments sampled from wall figures and cave deposits at Lascaux (Dordogne, France) cave, identifying four different types of natural haematite (hexagonal lamellar, trapezoidal lamellar, fibrous and almost amorphous haematite). This implies that the large variety of red hues used during the Solutrean and Magdalenian periods characterizing the majestic paintings from Lascaux were natural and the Palaeolithic artists knew where to find them (Chalmin et al. 2004a; Vignaud et al. 2006; de Balbín Behrmann and Alcolea González 2009; Menu 2009). Similarly, in Arcy-sur-Cure (Yonne, France), where the prehistoric living area also has rock art and where the red pigment found on the ground has been interpreted as a workshop to prepare the paints, the experimental protocol showed that such haematite was not the result of dehydration of goethite present in the neighbouring cave of Saint Moré (Chalmin et al. 2003; Salomon 2008). Overall, no direct cases of heating have been documented in pigments used to produce rock paintings during the European Palaeolithic period so far (Menu 2009; Salomon 2008; Salomon et al. 2015). If we move beyond the art and focus on pigments identified in archaeological deposits, the use of heated yellow ochre pigments has been reported at Mousterian levels of Es-Skhul (Mount Carmel, Israel) dated to 100 ky BP (Salomon et al. 2012), at Solutrean levels at Les Maîtreaux (Bossay-sur-Claise, Indre-et-Loire, France) and the Middle Magdalenian levels of Blanchard cave (Saint-Marcel, Indre, France) (Salomon et al. 2015), at Troubat (French Pyrenees), dated to 10 ky BP (Pomiès et al. 1999), at the Italians Late Epigravettian site of Riparo Dalmeri (Trento, Italy) (Gialanella et al. 2011) and the (Proto) Aurignacian levels of Fumane Cave (Verona, Italy) and in the Late Epigravettian sequence at Tagliente rock shelter (Verona, Italy) (Cavallo et al. 2018). So, while the technologies to obtain red pigments from yellow raw materials were already known in the Palaeolithic, it seems prehistoric artists did not resort to this technique to produce their art, neither in the Palaeolithic nor in later periods. Or at least, it has not yet been proven.
Cinnabar (HgS), the bright red form of mercury (II) sulphide that represents the most common ore of oxidized mercury found in nature, is another mineral with colouring properties sourced since more recent prehistoric times (Fig. 6). While it has been identified as early as in the Pre-Pottery Neolithic and the early Neolithic funerary and occupation deposits from the Middle East and Spain (Mellaart 1967; Molleson et al. 1992; Martín-Gil et al. 1995; Martínez et al. 1999; Goren et al. 2001; García-Borja 2004; Hunt et al. 2011; Domingo et al. 2012), examples of cinnabar use in prehistoric rock art are unknown. However, at the famous Neolithic site of Çatalhöyük (Turkey), this particular pigment was used both alone or mixed with red ochre to produce wall paintings. The mixed use of this mineral has been interpreted as intentional, to achieve a brighter red colour and/or related to the potential symbolic meaning of this more rare and harder to source raw material (Çamurcuoğlu 2015).
Black pigments
Black prehistoric paintings have been produced with two different classes of natural pigments. Organic blacks, characterized by carbon-based pigments, mainly in the form of charcoal or soot, and mineral blacks, based on manganese oxides/hydroxides, are the principal components identified in black pictographs worldwide (Chalmin et al. 2003; Vignaud et al. 2006; Salomon et al. 2008; de Balbín Behrmann and Alcolea González 2009; Menu 2009; Chalmin and Huntley 2017; Stuart and Thomas 2017; Gallinaro and Zerboni 2021; Domingo et al. 2021; Sepúlveda 2021). In nature, carbonaceous matter can be found as geological deposits of graphite, coal, etc., but disordered and heterogeneous amorphous carbonaceous matters like charcoal or soot can be made by firing organic substances including wood or bone (Coccato et al. 2015). Manganese oxides/hydroxides are naturally occurring minerals present in soil and sediments. They are frequently found as coatings on rocks and nodules. According to the chemical composition, valence states of the manganese metal (II, III, IV or mixed) and crystalline structure, more than 20 different mineralogical varieties can be distinguished among them. They can be divided into two main families based on the presence or absence of additional foreign cation (Chalmin et al. 2008). Manganite MnOOH, pyrolusite MnO2, bixbyite Mn2O3, hausmannite Mn3O4 and, on the other hand, romanechite Ba2Mn5O10‧xH2O, hollandite BaMn8O16‧xH2O represent some of the most recurring minerals taking part of such two categories, respectively. They can be found in a large variety of natural settings, also in the mixture, and in the form of wads, a general term to describe dark brown to black coloured earth pigments and materials in which manganese oxide/hydroxide are contained ca. 50% (Siddal 2018). In Western European Palaeolithic rock art, the use of both types of black pigments is equable in both French and Spanish sites. In fact, there is no systematic prevalence of the use of the organic black with respect to the inorganic one, even the former was used mainly for drawing outlines. In some sites, both are present (Menu and Walter 1992; Chalmin et al. 2003, 2006; Vignaud et al. 2006; Menu 2009; de Balbín Behrmann and Alcolea González 2009; Gay et al. 2020). For instance, in Ekain Cave (Basque Country, Spain), dating to the Magdalenian culture, black is the dominant colour and charcoal is the primary black pigment used to create the famous horses (Chalmin et al. 2003). Only two uncommon figures, one horse and two bears, have been painted with manganese oxide. This uncommon use, compared to the other paintings, suggested to archaeologists that this pigment was precious or symbolic. On the contrary, in Lascaux (Dordogne, France), only manganese black pigments with different mineral nature have been identified in the black figures. Their mineral composition is similar to that of several black crayons found next to the cave walls (Chalmin et al. 2003, 2004a, b). Similarly, in the Great Ceiling at Rouffignac Cave (Dordogne, France), at least three different types of barium-bearing manganese oxides (that could be assimilated to different black raw colouring materials) were used. Meanwhile, only charcoal has been detected in the black samples belonging to Grande Grotte of Arcy-sur-Cure (Yonne, France) or Altamira (Cantabria, Pain) (de Balbín Behrmann and Alcolea González 2009). In Gargas (Hautes-Pyrénées, France), famous for preserving more than 250 “negative hands” (or hand stencils) painted in red and black and only one in white, several black negative hands were made with charcoal (“Hand Sanctuary”), and the others with manganese oxides (“Entrance Gallery”), in which manganite in association with other Mn-based oxides like pirolusite and criptomelano has been identified (Chalmin et al. 2006).
If we move to post-Palaeolithic art, and more specifically to Spanish Levantine rock art, amorphous carbon from vegetal origin occurred more frequently than manganese-based pigments (Fig. 7) (Domingo et al. 2021). While the number of sites with black pigments analyzed is still low, this preliminary finding could suggest a better access to vegetal organic matters to be charred and employed as pictorial material, than to manganese sources (Domingo et al. 2021). Something similar happens with black pigments in Latin America where charcoal has been detected randomly in all prehistoric cultures (Sepúlveda 2021). This does not mean that the prehistoric Levantine painters were not ingenious in finding their raw materials. For example, at the Los Chaparros site (Albalate del Arzobispo, Spain), pigment analysis revealed the use of manganese oxides/hydroxides, whose precise mineral nature was not exactly characterized due to the low crystallinity of the analyzed compounds, as black pigment. Similarities between the analyses of the Levantine pigment and a dendrite mineralization located close to the rock art site suggest local procurement strategies (Pitarch et al. 2014).
Driven by the same motivations that led archaeologists and scientists to deeply study the goethite-haematite thermal transformation system, also manganese oxides/hydroxides have been highly examined under heat treatment to comprehend their transformation mechanisms and to gain insights into black pigment processing technologies during the Palaeolithic. In fact, some analytical studies and archaeological findings suggested to archaeologists a possible use of heated black pigments by prehistoric artists (Smith et al. 1999; Guineau et al. 2001; Chalmin et al. 2008). Thus, despite the absence of any colour change in the black pigments, it was supposed that the calcination could potentially induce modifications of the microstructural features, such as dehydration, a loss in the cohesion of the matter, facilitating grinding (Guineau et al. 2001; Chalmin et al. 2008). To verify these assumptions, Emily Chalmin and coworkers studied the thermal transformation of manganese oxides/hydroxides systems both in artificial and several archaeological samples. They found an analytical protocol to follow in order to recognize the application of heat treatment to this kind of black pigments using scanning electron microscopy combined with energy-dispersive X-ray analysis (SEM–EDX), X-ray diffraction (XRD), transmission electron microscopy (TEM) and X-ray absorption near edge structure (XANES) (Chalmin et al. 2003, 2004a, b, 2006, 2008). In particular, manganese oxides/hydroxides can undergo a series of successive phase transformations involving oxidation/dehydration/reduction reactions depending on the heating temperature and on the specific nature of the original mineral. As an example, at 360 °C, the monoclinic manganite (MnOOH) phase is transformed into tetragonal pyrolusite (MnO2) by oxidation and dehydration. At 560 °C, this pyrolusite is reduced as well into cubic bixbyite (Mn2O3); at the end, bixbyite is transformed into tetragonal hausmannite (Mn3O4) at 950 °C and so on (Chalmin et al. 2004a, b, 2008). Two different protocols have been defined depending on the presence or not of foreign cations in the manganese-based mineral, based on the evaluation of specific morphological, chemical and structural criteria to be followed during the changes induced by heating. The complete description of all heating patterns that could occur to manganese oxides/hydroxides when calcined is well summarized in Chalmin et al. (2008). From their results, they concluded that not heating treatment had been applied to any of the various black Mn-based pigments they studied from the paintings of various prehistoric sites such as Lascaux, Ekain, Gargas, Labastide, Combe Sauniere and Roucador (Chalmin et al. 2003, 2004a,b, 2006, 2008).
Finally, to rock art research, the identification of carbon-based organic pigments is of special interest since it opens the way for radiocarbon dating. Dating rock art is one of the major challenges faced by archaeologists, and it is absolutely necessary to place the art in the cultural context (Steelman and Rowe 2012; Ochoa et al. 2021). Not without technical difficulties and strict protocols to be followed in order to minimize damaging the paintings, in Western European Palaeolithic caves, both French and Spanish researchers have focused on determining the age of spectacular rock paintings by dating charcoal pigments, obtaining more than a hundred dates so far (Steelman and Rowe 2012; Ochoa et al. 2021). Standard procedures have been adopted for dating archaeological charcoal, using acid to remove carbonates and combustion to collect carbon for AMS 14C dating. Most dates obtained on pictographs worldwide have been on charcoal pigmented paintings. There are about 150 publications on dating charcoal pigment in rock art that involve at least six laboratories (Rowe 2012), providing variable and sometimes contested results (Ochoa et al. 2021). A balance between the need to date the art and the impact of such actions should be found since while sampling large paintings like the ones found in Palaeolithic art may cause only small scars to the charcoal drawings, dating much smaller Levantine paintings may result in the destruction of a large part of the motifs to obtain enough matter for dating.
Yellow pigments
The prehistoric yellow pigments are usually characterized by yellow ochres (see Red pigments for the definition of ochres and earth pigments) whose brownish-yellow typical colour is due to the presence of goethite (α-FeOOH), an iron (III) oxide-hydroxide natural occurring mineral, as the main chromophore. Similar to what happens for the use of haematite and red ochres, the use of goethite and goethite-rich materials is common to all prehistoric traditions worldwide to create yellow paintings. However, they are uncommon in works of art prior to the Late Neolithic (Siddal 2018). Regarding Western European Palaeolithic rock art, according to Menu (2009), about 1% of the paintings were completed with yellow or brown colour, some of which are displayed on the Lascaux site. In this case, they are based on yellow ochre (Chalmin et al. 2006; Vignaud et al. 2006; Menu 2009; de Balbín Behrmann and Alcolea González 2009). Moreover, in some works from the end of the Magdalenian, jarosite, a basic hydrous sulphate of potassium and iron KFe3(SO4)2(OH)6, has been also found sporadically. Jarosite is a beautiful yellow mineral, quite rare, available in Ariège near caves (Menu 2009). Jarosite has been also detected in several prehistoric yellow figures from Latin America (Sepúlveda 2021). Furthermore, while it is not specifically rock art, it is important to mention the unique collection of Palaeolithic painted portable art coming from Parpalló Cave, (Gandía, Spain), one of the most important Palaeolithic sites in the Spanish Mediterranean region. With over 5600 decorated plaquettes, this unique site has provided a reference collection for the study of the Palaeolithic art in Europe since it covers the entire archaeological sequence from the Gravettian to the Magdalenian periods (32–14,000 cal. BP). The plaquettes are characterized by zoomorphic and sign depictions and, in addition to the predictable use of red and black pigments, a large amount of those are painted with yellow materials, identified as yellow ochres (Roldan et al. 2016).
White pigments
The occurring of white colour in Western and Mediterranean European prehistoric rock art is not frequent. Prehistoric European artists did not commonly employ white pigments or at least they have not been as well preserved as red and black paintings. Both ethnography and archaeology have emphasized the problematic conservation of white pigments, which may be providing a wrong picture of the colour preferences of prehistoric artists (e.g. Chaloupka 1993; Chippindale and Taçon 1998; Domingo 2005; Domingo et al. 2018). During the Palaeolithic, white paintings have been only found once on a negative hand in the Gargas Cave, in the Pyrenees, consisting of talc (Mg3Si4O10(OH)2) and clay (Menu 2009). Later on, during the post-Palaeolithic, and more specifically within the Levantine rock art context, a few examples demonstrate a minor use of white pigment to produce both monochrome and bichrome paintings (Domingo et al. 2021). Hernanz et al. (2008) analyzed a bicolour bull composed of red and white brushstrokes in the Marmalo IV shelter of Sierra de las Cuerdas (Cuenca). Compounds like α-quartz (α-SiO2), anatase (TiO2), apatite (Ca5(PO4)3), muscovite (KAl2(Si3Al)O10(OH,F)2), gypsum (CaSO4·2H2O) and illite ((K,H3O)(Al,Mg,Fe)2(Si,Al)4O10[(OH)2,(H2O)]) were detected in the white lines of the painted figure. In particular, the white traces in the composition of the painted animal seem to enhance the perception of the muscles of the red bull. Microstratigraphic studies revealed that the white layer was superimposed to the red one. A similar use of the white pigment for highlighting and emphasizing details of painted figures had been found in other rock art sites of the Valltorta-Gasulla complex (Civil and Centelles sites), again employed to underline details and adornments in human figures (Domingo 2005; Domingo et al. 2021). Furthermore, another example of white painting found in the Iberia peninsula is located at the rock-art shelter Abrigo del Águila (Badajoz), belonging to Iberian Schematic rock art tradition (Rosina et al. 2018). The micro-Raman analyses pointed out the presence of anatase and the authors assumed that the white figures could have been made using minerals from the illite-kaolinite group. Moving from European borders, white pigments are most frequently found in prehistoric rock art coming from other parts of the world, like in Australian, African and American rock art creations. In particular, kaolinite (Al2Si2O5(OH)4), huntite (CaMg3(CO3)4), quartz (α-SiO2), gypsum (CaSO4·2H2O), calcite (CaCO3), and calcium oxalates (CaC2O2·(H2O)x) are some of the most detected compounds used for the creation of white paintings (Stuart and Thomas 2017; Chalmin and Huntley 2017; Gallinaro and Zerboni 2021; Sepúlveda 2021). Interesting is also the use of calcined bones in Argentina (Wainaright et al. 2002).
Prehistoric paints (binders, recipes and application techniques)
In prehistoric times, the production of the artworks required significant advanced planning to collect the raw materials used to prepare the sourcing, processing and application tools, the crayons and the paints necessary to create paintings. The complexities of this process and the amount of time invested by prehistoric artists demonstrate that they were not based on improvisation (Fiore 2007). The range of mineral and organic pigments described above were used in prehistoric times either for dry use (as crayons) to produce drawings (with astonishing examples in places like the sites of Chauvet or Ekain, to name a few) or as the bases to produce paint for their monochromatic and polychromatic creations (like the famous polychrome bisons of Altamira Cave). In this case, the raw pigments were finely ground, sometimes even sieved and finally mixed with a liquid medium (vehicle) and other additives and natural binding substances (animal fat or plant extracts) to facilitate adherence. Different granulometries reflect the quality of the grinding and/or sieving process and the skills of the artists (Hernanz et al. 2008).
While little archaeological information on binders is available, ethnoarchaeology demonstrates that the use of binding agents such as honey, plant resin, natural wax, animal fat, blood and others (Ravenscroft 1985) is necessary for the art to survive, especially in the open air, since paintings produced without binders disappear in a few years or decades depending on their exposure (Domingo et al. 2018). Today, the problematic identification of these substances is most likely related to the low residual amount of their constituent organic macromolecules (carbohydrates, lipids, proteins as main classes and their residuals) in the pictorial paints due to their chemical transformations caused by their aging and degradation as well as to the intrinsic limits and technical constrains of the experimental techniques used so far for identification purposes (Domingo et al. 2021). Moreover, the potential carbohydrates, lipids and proteins that could have been used as binders in prehistoric times are also some of the nutrients used by microorganism (like fungi, bacteria, lichens and so on) for growth and they could be fixed as calcium oxalates at the end of the feeding actions (Rampazzi 2019; Domingo et al. 2021). Thus, should any residual amounts be left, the detection would be challenging (Spades and Russ 2005).
The use of lipid binders was detected at various Palaeolithic sites, such as Fontanet, Enlène and Les Trois Frères, (Pepe et al. 1991). At some sites of different chronologies, these organic binders were interpreted as consistent with animal fats, including for example the Palaeolithic sites of Tito Bustillo (Navarro and Gómez 2003), some Levantine paintings of la Saltadora site (Roldan et al. 2018) or the more recent art of the dolmen of Dombate, where the use of milk fat and butter was suggested (Bello and Carrera 1997). Further examples have been also reported in other regions around the world (e.g. Boschín et al. 2002; Prinsloo et al. 2008; Moya et al. 2016; Brook et al. 2018). Nevertheless, attention should be paid to whether organic molecules identified today are really of prehistoric origin. For example, fatty acids, among the main constituent of lipids, have been identified equally both in the paint and in natural rock coating, composed mainly by calcium oxalate and to a lesser extent by gypsum and clays, in micro-samples from the Lower Pecos Region of southwestern Texas (Spades and Russ, 2005). The authors attributed such contents to microbial activity on the rock surfaces due to lichens, bacteria and others that naturally live on the rock surface and they indicated that fats or oils were either not used to prepare the paints or that if such binders were used, they had already degraded when analyzed by gas chromatography–mass spectrometry (GC–MS). Thus, the presence of this kind of accretions commonly correlated to the paints and often fossilized on them could create misleading interpretations when investigating organic molecules. Not only patinas, but also the analyses of raw ochres collected close to several rock art sites in Australia showed a high amount of organic matter in the pigments themselves, such as lichens and bacteria (Ridges et al. 2000). Thus, the identification of biological molecules such as lipids and proteins, on the rock art pictographs, has to be carefully considered since they could also belong to the microorganisms living on rock surfaces, coatings and pigments, like lichens (García et al. 2001), bacteria (Roldan et al. 2018), fungi and algae. Thus, they cannot be immediately attributed to original binders used to produce prehistoric paint (Hernanz 2015). This issue has to be also taken into account when considering radiocarbon dating rock painting samples (Bednarik 2002) since organic matter from the natural occurring environment is undistinguishable from that coming from organic materials used as part of the prehistoric paints or binders. Another example regarding proteinaceous binders is found at la Saltadora site in Spain, where the presence of casein peptides of animal origin has been identified on both pigmented and non-pigmented areas by proteomic approaches, as described by Roldán et al. (2018). The presence of casein in Levantine pigments suggests the authors the use of milk as binding media. If finally proven, these results would be highly interesting from the archaeological point of view, and more specifically for the chrono-cultural debates surrounding the authorship of Levantine paintings, since the use of milk could be interpreted as a confirmation that it was created by farming communities and not by the last hunter-gatherers. Nonetheless, another recent finding warns of the dangers of making direct assumptions such as this since the use of milk as a binder has been also detected among hunter-gatherer communities living in South Africa 49,000 years ago (Vila et 2015). Moreover, as the authors of la Saltadora study say (Roldán et al. 2018), there is still no direct evidence that the detected casein is not a ubiquitous contemporary contaminant since it has been identified both in un/pigmented areas so more scientific efforts have to be spent to resolve this question. Among other uses, milk and casein have been also documented in cultural heritage studies as a fixative or stabilizing agent in different kinds of more recent painted and stone artworks (Chamberly et al. 2009; Snethlage and Sterflinger 2011).
The use of blood as an agent has not been detected in any European site, and it is rare in other regions, with only a few references from Australian (Loy et al. 1990) and African sites (Lewis-Williams 1994: 281; Williamson 2000). Fibres and plant extracts have been also suggested as potential binders in Australia (Watchman and Cole 1993) and South America (Boschín et al. 2002).
Other inorganic additives intentionally added to prehistoric paintings include clays (e.g. Baffier et al. 1999; Hernanz et al. 2008; Hamenau et al. 1995); quartz (like in Lascaux, Clottes 1997); quartz and amber in Altamira (Groenen, 2000); K-feldspar at La Vache, Salón Noir de Niaux and de Fontane (Clottes, 1997); quartz and K-feldspar in Arenaza (Garate et al. 2004); talc in some Schematic art sites (Hameau et al. 2001) and mixed with biotite in Niaux (Clottes, 1997), burned bones in Western European Palaeolithic (de Balbín Behrmann and Alcolea González 2009), Levantine (Hernanz et al. 2008) and Schematic art (e.g. Hameau et al. 2001). This variety of additives, often used to obtain different colour hues or textures, has been used to talk about the preparation of different paint recipes in Palaeolithic times (Menu and Walter, 1996; Baffier et al. 1999; Hameau et al. 2001; Chalmin et al. 2003; Garate et al. 2004, among others), reflecting elaborated technologies and different cultural choices in paint preparation. The degree of paint homogeneity of the rock art panels or sites may provide information for discussing the completion times of the artworks. For instance, Garate et al. (2004) deduce that the paintings of Arenaza result from a single intervention based on the consistency of the paints used, while Groenen (2000) suggests that the production of the hand stencils from Gargas stretched over a period of time considering the variety of recipes detected.
Once the pictorial mixture was ready and depending on the period and region, the paint was applied using a variety of techniques. For example, European Palaeolithic artists used different types of brushes and pads, hands and fingers and the blow painting techniques (i.e. blowing the paint directly from the mouth or through hollow tubes), while Levantine artists only used a variety of brushes, as deduced from the fine strokes characteristic of this tradition (Menu 2009; D’Errico et al. 2016). The nature of the brushes is unknown, though, since they are not preserved in the archaeological record (Domingo 2020).
Conclusions
The systematic application of scientific analyses from different disciplines (physics, chemistry, mineralogy, petrography, geology and biology) to the study of prehistoric pigments and paints have improved the way prehistoric art and colours are analyzed and understood today, allowing the discovery of multiple uses of different raw materials with colouring properties in prehistoric times and expanding the frontiers of archaeological knowledge on one of the most fascinating legacies of the past: rock art.
As discussed in this paper, building a systematic knowledge about the raw materials and their potential sources, the techniques, instruments and processes involved in the production of prehistoric art and exploring their patterns of change over space and time are questions of interest to archaeological research to gain information on the palette, the technologies and the socio-cultural practices of ancient artists as well as on aspects of social dynamics and productive processes. Such an approach is showing that while the amount of minerals and colours used in prehistoric art is limited, the patterns of production of different regions or periods are different, demonstrating that the exploration of the non-visual aspects of the art offers new avenues to track past social identities (see for example Vergara and Troncoso, 2015). As Gosselain (1992) states, the visual aspects of the artefacts (in rock art, variations in form and subject matter) can be easily replicated, while non-visual aspects (or those that we will have to explore through archaeometry, such as the nature of the raw materials used and the transformation practices, pigments recipes and so forth) are more difficult to copy, thus providing more opportunities to explore the more stables aspects of social identities (Domingo and Fiore, 2014).
As debated along this paper, and broadly speaking, while prehistoric humans were constraint by a limited number of raw materials available in nature (mainly 4 colours—red, black, yellow and white—were used all around the globe), analytical approaches are demonstrating certain variability in different steps of paint production (raw materials used, grain sizes, recipes, etc.) and application between different traditions. These variations do not simply result from environmental constraints, such as the availability of certain raw materials and no others since the absence of local supplies was solved through different strategies, such as long-distance procurement or the development of exchange networks. Thus, the variability observed in the different steps of the process of production seems to be strongly influenced by cultural decisions and choices, traditions and probably even beliefs.
Physico-chemical analyses have also potential as a tool to identify whether pigment remains located on the walls of caves and rock shelters are natural formations or intentionally produced by humans, and also as an authentication tool to confirm whether any new finds are truly prehistoric (Garate et al. 2004), even though subject to certain limits (García et al. 2001).
Furthermore, physico-chemical approaches are also important to advance knowledge on pigment conservation, of interest to understand how the art has survived, predict the long-term behaviour of pigments and bedrocks and identify potential risks. They are also crucial to develop best conservation practices and protocols, giving careful consideration to the fact that any materials introduced (modern pigments used to tone areas of bedrock loss, materials for consolidation and so forth) or removed (crusts) in any conservation interventions could impact archaeological approaches to past pigments, paints, technologies and practices.
Today the range of portable non-invasive spectroscopic techniques available to archaeologists has been a push for the scientific analysis of prehistoric art and pigments, minimizing impacts on this unique and fragile heritage. They have opened up new opportunities for direct on-site analysis, enabling the application of new analytical research approaches to the study of rock art and providing guidance to avoid or at least minimize the need to resort to sampling and the use of invasive analytical methods (whether non-destructive or destructive). While sampling is still necessary, portable non-invasive methods allow us to perform more targeted sampling, which should only be performed as a last resort when no other non-invasive techniques can answer our research questions. This sampling strategy reduces potential impacts on the art.
What is clear from this review is the importance of multitechnical and multistep multidisciplinary approaches in studying prehistoric pigments and paints to gain a more comprehensive understanding of the many uses of these particular archaeological remains and the many aspects of past cultures they can shed new light on. We are confident that in the next few years, continued technological advances in analytical techniques will improve our approaches to rock art, opening new avenues of research now still unthinkable.
Data availability
Not applicable.
Code availability
Not applicable.
References
Aceto M (2021) The palette of organic colourants in wall paintings. Archaeol Anthropol Sci. https://doi.org/10.1007/s12520-021-01392-3
Adams JL (1988) Use wear on manos and hide processing Stones. J Field Archaeol 15:307–315
Allain J, Rigaud A (1989) Colles et mastics au Magdalénien. In: Olive M, Taborin Y (eds.) Nature et fonction des foyers préhistoriques: actes du colloque international de Nemours 1987, Mémoires du Musée de Préhistoire d’Ille de France, 2:221–3
Aramendia J, Fdez-Ortiz de Vallejuelo S, Maguregui M, Martinez-Arkarazo I, Giakoumaki A, Pitarch Martí A, Madariaga JM, Ruiz JF (2020) Long-term in situ non-invasive spectroscopic monitoring of weathering processes in open-air prehistoric rock art sites. Anal Bioanal Chem 412:8155–8166
Arizzi A, Cultrone G (2021) Mortars and plasters – How to characterise hydraulic mortars. Archaeol Anthropol Sci. https://doi.org/10.1007/s12520-021-01404-2
Aubert M, Brumm A, Ramli M, Sutikna T, Saptomo EW, Hakim B, Morwood MJ, van den Bergh GD, Kinsley L, Dosseto A (2014) Pleistocene cave art from Sulawesi, Indonesia. Nature 514:223–227
Aubert M, Setiawan P, Oktaviana AA et al (2018) Palaeolithic cave art in Borneo. Nature 564:254–257. https://doi.org/10.1038/s41586-018-0679-9
Audion F, Plisson H (1982) Les ocres et leurs témoins au Paléolitique en France: enquête et experiences sur leur validité archéologique. Cahiers Du Centre De Recherches Préhistoriques 8:33–80
Baffier D, Girard M, Menu M, Vignaud C (1999) La couleur à la grande grotte d’Arcy-sur-Cure (Yonne). L’anthropologie 103:1–21
Bar-Yosef O (1997) Symbolic expressions in Later Prehistory of the Levant: why are they so few? In: Conkey M, Soffer O, Stratmann D (ed.) Beyond art: Pleistocene image and symbol. Memoirs of the California Academy of Science. 23. San Francisco, pp 161–187.
Bayarri V, Sebastián M A, Ripoll S (2019) Hyperspectral imaging techniques for the study, conservation and management of rock art. Appl Sci 9:5011. https://doi.org/10.3390/app9235011
Beck L, Genty D, Lahlil S, Lebon M, Tereygeol F, Vignaud C, Reiche I, Lambert E, Valladas H, Kaltnecker E, Plassard F, Menu M, Paillet P (2013) Non-destructive portable analytical techniques for carbon in situ screening before sampling for dating prehistoric rock paintings. Radiocarbon 55(2–3):436–444
Beck L, Rousselière H, Castaing J, Duran A, Lebon M, Moignard B, Plassard F (2014) First use of portable system coupling X-ray diffraction and X-ray fluorescence for in-situ analysis of prehistoric rock art. Talanta 129:459–464
Becker H (2021) Pigment nomenclature in the ancient Near East, Greece, and Rome. Archaeol Anthropol Sci. https://doi.org/10.1007/s12520-021-01394-1
Bednarik R G (2002) The Dating of Rock Art: a Critique. J Archaeol Sci 29:1213–1233Bello J Mª, Carrera F (1997) Las pinturas del monumento megalítico de Dombate: estilo, técnica y composición. In: Rodríguez A (ed.) O neolítico atlántico e as orixes do megalitismo: Actas do Coloquio Internacional (Santiago de Compostela, 1–6 de Abril de 1996). Consello da Cultura Galega: Universidade, Santiago de Compostela, pp 819–828
Bello JM, Carrera F (1997) Las pinturas del monumento megalítico de Dombate: estilo, técnica y composición. In: Rodríguez A (ed) O neolítico atlántico e as orixes do megalitismo: Actas do Coloquio Internacional (Santiago de Compostela, 1–6 de Abril de 1996). Universidade, Santiago de Compostela, Consello da Cultura Galega, pp 819–828
Beyries S, Inizan M L (1982) Typologie, ochre, function. In Cahen D (ed.) Tailler! Pour quoi faire, Musé Royal de l’Afrique centrale, Tervuren, pp: 313–322
Bersani D, Lottici PP (2016) Raman spectroscopy of minerals and mineral pigments in archaeometry. J Raman Spectrosc 47:499–530
Blas MA (2003) Estelas con armas: arte rupestre y paleometalurgia en el norte de la Península Ibérica. In: Balbín R, Bueno P (eds.) Primer Simposium Internacional de Arte Prehistórico de Ribadesella: el arte prehistórico desde los inicios del siglo XXI, pp 391–417
Bonneau A, Pearce DG, Pollard AM (2012) A multi-technique characterization and provenance study of the pigments used in San rock art, South Africa. J Archaeol Sci 39:287–294
Boschín MT, Seldes AM, Maier M, Casamiquela RM, Ledesma RE, Abad GE (2002) Chemical analysis of the organic and inorganic fractions of rock art and pastes from Argentinian Septentrional Patagonian archaeological sites. Zephyrus 55:183–198
Brook GA, Franco N, Cherkinsky A, Acevedo A, Fiore D, Pope TR, Weimar RD, Neher G, Evans HA, Salguero TT (2018) Pigments, binders, and ages of rock art at Viuda Quenzana, Santa Cruz, Patagonia (Argentina). J Archaeol Sci Rep 21:47–63. https://doi.org/10.1016/j.jasrep.2018.01.004
Brunet J, Callède B, Orial G (1982) Tarascon sur Ariège (Ariège), grotte de Niaux: mise en évidence decharbon de bois dans les traces préhistoriques du Salon Noir. Stud Conserv 27:173–179
Brunetti B, Miliani C, Rosi F, Doherty B, Monico L, Romani A, Sgamellotti A (2016) Non-invasive investigations of paintings by portable instrumentation: the MOLAB experience. Top Curr Chem (z) 374:10. https://doi.org/10.1007/s41061-015-0008-9
Brum A, Oktaviana AA, Burhan B, Hakim B, Lebe R, Zhao J, Sulistyarto PH, Ririmasse M, Adhityatama Sh, Sumantri I, Aubert M (2021) Oldest cave art found in Sulawesi. Sci Adv 7: eabd4648
Burgio L (2021) Pigments, dyes and inks – their analysis on manuscripts, scrolls and papyri. Archaeol Anthropol Sci. https://doi.org/10.1007/s12520-021-01403-3
Cacho C, Papí C, Sánchez A, Alonso F (1996) La cestería decorada de la cueva de los Murciélagos (Albuñol. Granada). In: Querol MA, Chapa T (coords.) Homenaje al Profesor Manuel Fernández Miranda. Complutum-Extra 6(1), Madrid, pp 105–122.
Çamurcuoğlu DS (2015) The wall paintings of Çatalhöyük (Turkey): materials, technologies and artists. University College London, Unpublished Phd
Caroselli M, Ruffolo SA, Piqué F (2021) Mortars and plasters – how to manage mortars and plasters conservation. Archaeol Anthropol Sci. https://doi.org/10.1007/s12520-021-01409-x
Cavallo G, Riccardi M P (forthcoming) Glass-based pigments in painting. Archaeol Anthropol Sci (this Topical Collection)
Cavallo G, Fontana F, Gialanella S, Gonzato F, Riccardi MP, Zorzin R, Peresani M (2018) Heat treatment of mineral pigment during the upper Palaeolithic in north-east Italy. Archaeometry 60(5):1045–1061. https://doi.org/10.1111/arcm.12360
Chalmin E, Menu M, Vignaud C (2003) Analysis of rock art paintings and technology of Palaeolithic painters. Meas Sci Technol 14:1590–1597
Chalmin E, Menu M, Pomiès MP, Vignaud C, Aujoulat N, Geneste J-M (2004a) Les blasons de Lascaux. The “blazons” of the Lascaux Cave. L’anthropologie 108:571–592
Chalmin E, Vignaud C, Menu M (2004b) Palaeolithic painting matter: natural or heat-treated pigment? Appl Phys A 79:187–191
Chalmin E, Vignaud C, Salomon H, Farges F, Susini J, Menu M (2006) Minerals discovered in paleolithic black pigments by transmission electron microscopy and micro-X-ray absorption near-edge structure. Appl Phys A 83:213–218
Chalmin E, Vignaud C, Farges F, Menu M (2008) Heating effect on manganese oxihydroxides used as black Palaeolithic pigment. Phase Transit 81(2):179–203
Chalmin E, Huntley J (2017) Characterizing Rock Art Pigments. In: David B, McNiven IJ (ed.) The Oxford handbook of the archaeology and anthropology of rock art, Oxford University Press. 1093/oxfordhb/9780190607357.013.48
Chaloupka G (1993) Journey in time: the 50 000 years story of aboriginal rock art of Arnhem Land. Reed New Holland, Sydney
Chamberly A, Di Maro A, Sanges C, Severino V, Tarantino M, Lamberti A, Parente A, Arcari P (2009) Improved procedure for protein binder analysis in mural painting by LC-ESI/Q-q-TOF mass spectrometry: detection of different milk species by casein proteotypic peptides. Anal Bioanal Chem 395:2281–2291
Chippindale C, Taçon PSC (1998) The many ways of dating Arnhem Land rock-art, north Australia. In: Chippindale C, Taçon PSC (eds) The archaeology of rock-art. Cambridge University Press, Cambridge, pp 90–111
Clottes J (1997) New laboratory techniques and their impact on Paleolithic Cave Art. In: Conkey M, Soffer O (eds.) Beyond art: Pleistocene image and symbol. Memoirs of the California Academy of Sciences 23, pp 37–52.
Clottes J, Menu M, Walter Ph (1990) La préparation des peintures magdaléniennes des cavernes ariégeoises. Bull Sot Prehistorique Fr 87(6):170–192
Coccato A, Jehlick J, Moens L, Vandenabeele P (2015) Raman spectroscopy for the investigation of carbon-based black pigments. J Raman Spectrosc 46:1003–1015
Cornell R M, Schwertmann U (2003) The iron oxides: structure, properties, reactions, occurrences and uses, 2nd, completely revised and extended edition. Wiley-VCH. https://doi.org/10.1002/3527602097.ch1.
Couraud C (1988) Pigments utilices en Préhistoire. Provenance, préparation, mode d’utilisation. L’Anthropologie 92(1):17–28
Cucci C, Delaney JK, Picollo M (2016) Reflectance hyperspectral imaging for investigation of works of art: old master paintings and illuminated manuscripts. Acc Chem Res 49:2070–2079
Cuenca-Solana D, Gutiérrez-Zugasti I, Ruiz-Redondo A, González-Morales MR, Setién J, Ruiz-Martínez E, Palacio-Pérez E, de las Heras-Martín C, Prada-Freixedo A, Lasheras-Corruchaga JA (2016) Painting Altamira Cave? Shell tools for ochre-processing in the Upper Palaeolithic in northern Iberia. J Archaeol Sci 74:135 – 151
de Balbín Behrmann R, Alcolea González JJ (2009) Les colorants de l’art paléolithique dans les grottes et en plein air. Colors in the palaeolithic painting in cave and outdoors. L’Anthropologie 113(3–4):559–601
Defrasne C, Chalmin E, Bellot-Gurlet L, Thirault E, André G (2019) From archeological layers to schematic rock art? Integrated study of the Neolithic pigments and pigmented rocks at the Rocher du Château (Western Alps, Savoie, France). Archaeol Anthropol Sci 11(11):6065–6091. https://doi.org/10.1007/s12520-019-00882-9
DeLaine J (2021) Production, transport and on-site organisation of Roman mortars and plasters. Archaeol Anthropol Sci. https://doi.org/10.1007/s12520-021-01401-5
Delibes de Castro G (2000) Cinabrio, huesos pintados en rojo y tumbas de ocre: ¿Prácticas de embalsamamiento en la Prehistoria? In: Olcina M, Soler J (eds) Scripta in honorem Enrique A. Llobregat Conesa, Alacant, pp 223–36
D’Errico F, Backwell L (2016) Earliest evidence of personal ornaments associated with burial: the Conus shells from Border Cave. J Hum Evol 93:91–108
D’Errico F, García R, Rifkin R (2012) Technological, elemental and colorimetric analysis of an engraved ochre fragment from the Middle Stone Age levels of Klasies River Cave 1. South Africa J Archaeol Scie 39(4):942–952
D’Errico F, Bouillot LD, García-Diez M, Pitarch Martí A, Garrido Pimentel D, Zilhão J (2016) The technology of the earliest European cave paintings: El Castillo Cave, Spain. J Archaeol Sci 70:48–65
di Lernia S, Bruni S, Cislaghi I, Cremaschi A, Gallinaro M, Gugliemi V, Mercuri AM, Poggi G, Zerboni A (2016) Colour in context. Pigments and other coloured residues from the Early-Middle Holocene site of Takarkori (SW Libya). Archaeol Anthropol Sci 8:381–402. https://doi.org/10.1007/s12520-015-0229-4
Doehne E, Price C A (2010) Heritage in stone: rock art, quarries, and replacement stone. In: Getty Conservation Institute (ed.) Stone conservation – an overview of current research, 2nd Edition
Domingo I (2005) Técnica y ejecución de la figura en el Arte Rupestre Levantino. Hacia una definición actualizada del concepto de estilo: validez y limitaciones. Servei de Publicacions de la Universitat de València, València
Domingo I (2020) First art. Artists from Prehistory. In Domingo I Palomo, A. (eds.) First art. Artists from Prehistory. Barcelona, Museu d’Arqueologia de Catalunya, pp 10–31
Domingo I, Fiore D (2014) Style. Its role in the archaeology of art. Encyclopedia of Global Archaeology. Springer, New York, pp 7104–7111
Domingo I, García-Borja P, Roldán C (2012) Identification, processing and use of red pigments in the Valencian early Neolithic (Spain). Archaeometry 54(5):868–892
Domingo I, May SK, Smith C (2018) Etnoarqueología y arte rupestre en el siglo XXI: de la analogía directa a la definición del método arqueológico. Kobie Serie Anejo 16:163–180
Domingo I, Vendrell M, Chieli A (2021) A critical assessment of the potential and limitations of physicochemical analysis to advance knowledge on Levantine rock art. Quat Int 572:24–40. https://doi.org/10.1016/j.quaint.2020.09.020
Domingo I, Smith C, Jackson G, Roman D (2020) Hidden sites, hidden images, hidden meanings: does the location and visibility of motifs and sites correlate to restricted or open access? J Archaeol Method Theory 27:699–722
Dorn RI, Whitley DS, Cerveny NV, Gordon SJ, Allen CD, Gutbrod E (2008) The Rock Art Stability Index: a new strategy for maximizing the sustainability of rock art. Herit Mana 1(1):37–70
Eastaugh N, Walsh V, Chaplin T, Siddall R (2008) Pigment compendium: optical microscopy of historical pigments. Taylor & Francis
Ergenç D, Fort R, Varas−Muriel M J, Alvarez de Buergo M (2021) Mortars and plasters – how to characterise aerial mortars and plasters. Archaeol Anthropol Sci. https://doi.org/10.1007/s12520-021-01398-x
Fiore D (2007) The economic side of rock art: concepts on the production of visual images. Rock Art Res 24:149–160
Formicota V (1986) Una sepoltura infantile del Neolítico Medio alle Arene Candide. Prehistoria Alpina 22:169–175
Gallinaro M, Zerboni A (2021) Rock, pigments, and weathering. A preliminary assessment of the challenges and potential of physical and biochemical studies on rock art from southern Ethiopia. Quat Int 572:41–51. https://doi.org/10.1016/j.quaint.2020.05.056
Garate D, Laval E, Menu M (2004) Etude de la matière colorante de la grotte d’Arenaza (Galdames, Pays Basques, Espagne). L’anthropologie 108:251–289
García M, Ortega Ma I, Martín MA, Hortolà P, Zuluaga Ma C (2001) Arte rupestre de estilo paleolítico del Portalón de Cueva Mayor de la Sierra de Atapuerca (Ibeas de Juarros, Burgos): ¿cronología paleolítica o contemporánea? Trab Prehist 58(1):153–169
García Borja P, Domingo I, Roldán C, Verdasco C, Ferrero J, Jardón P, Bernabeu IJ (2004) Aproximación al uso de la materia colorante en la Cova de l’Or. Recerques Del Museu D’alcoi 13:35–52
Gay M, Alfeld M, Menu M, Laval E, Arias P, Ontañón R, Reiche I (2015) Palaeolithic paint palette used at La Garma cave (Cantabria, Spain) investigated by means of combined in situ and synchrotron X-ray analytical methods. J Anal at Spectrom 30:767–776
Gay M, Müller K, Plassard F, Cleyet-Merle J-J, Arias P, Ontañón R, Reiche I (2016) Efficient quantification procedures for data evaluation of portable X-ray fluorescence–Potential improvements for Palaeolithic cave art knowledge. J Archaeol Sci Rep 10:878–886
Gay M, Plassard F, Müller K, Reiche I (2020) Relative chronology of Palaeolithic drawings of the Great Ceiling, Rouffignac cave, by chemical, stylistic and superimposition studies. J Archaeol Sci Rep 29:102006
Gialanella S, Belli R, Dalmeri G, Lonardelli I, Mattarelli M, Montagna M, Toniutti L (2011) Artificial or natural origin of hematite-based red pigments in archaeological contexts: the case of Riparo Dalmeri (Trento, Italy). Archaeometry 53(5):950–962. https://doi.org/10.1111/j.1475-4754.2011.00594.x
Gliozzo E (2021) Pigments – mercury-based red (cinnabar-vermilion) and white (calomel) and their degradation products. Archaeol Anthropol Sci. https://doi.org/10.1007/s12520-021-01402-4
Gliozzo E, Burgio L (2021) Pigments – arsenic-based yellows and reds. Archaeol Anthropol Sci. https://doi.org/10.1007/s12520-021-01431-z
Gliozzo E, Ionescu C (2021) Pigments – lead-based whites, reds, yellows and oranges and their alteration phases. Archaeol Anthropol Sci. https://doi.org/10.1007/s12520-021-01407-z
Gliozzo E, Pizzo A, La Russa MF (2021) Mortars, plasters and pigments - research questions and sampling criteria. Archaeol Anthropol Sci. https://doi.org/10.1007/s12520-021-01393-2
González G, Sagarzazu A, Villalba R (2000) Study of the mechano-chemical transformation of goethite to hematite by TEM and XRD. Mater Res Bull 35:2295–2308. https://doi.org/10.1016/S0025-5408(00)00434-7
Goñi A, Rodrigues A, MD C, Martín D, Francisco MI (1999) La Tecnología de los elementos de adorno personal en materias minerales durante el Neolítico Medio. El ejemplo del poblado de Cabecicos Negros (Almería). II Congrés de Neolític a la Península Ibèrica. Saguntum-Plav, Extra 2:163–170
Goren Y, Goring-Morris AN, Segal I (2001) The technology of skull modelling in the Pre-Pottery Neolithic B (PPNB): regional variability, the relation of technology and iconography and their archaeological implications. J Archaeol Sci 28:671–690
Gosselain OP (1992) Technology and style: poterers and pottery among the Bafia of Cameroon. Man 27:559–586
Groenen M (2000) Sombra y luz en el Arte Paleolítico. Ariel, Madrid
Guineau B, Lorblanchet M, Gratuze B, Dulin L, Roger P, Akrich R, Muller F (2001) Manganese black pigments in prehistoric paintings: the case of the Black Frieze of Pech Merle (France). Archaeometry 43:211–255
Hameau P, Menu M, Pomies M-P, Walter P (1995) L’art schématique postglaciaire dans le sud-est de la France: analyses pigmentaires. Bull Soc Préhist Fr 92:108–119
Hameau Ph, Cruz V, Laval E, Menu M, Vignaud C (2001) Analyse de la peinture de quelques sites postglaciares du Sud-Est de la France. L’anthropologie 105(4):611–626
Hernanz A, Gavira-Vallejo JM, Ruiz-López JF, Martin S, Maroto-Valiente A, de Balbín-Behrmann R, Menéndez M, Alcolea-Gonzáleze JJ (2011) Spectroscopy of Palaeolithic rock paintings from the Tito Bustillo and El Buxu Caves, Asturias. Spain J Raman Spectrosc 43(11):1644–1650
Hernanz A (2015) Raman spectroscopy of prehistoric pictorial materials. Prehistoric Art as Prehistoric Culture. Archaeopress Archaeology, Oxford, pp 11–20
Hernanz A, Gavira-Vallejo JM, Ruiz JF (2007) Calcium oxalates and prehistoric paintings. The usefulness of these biomaterials. J Optoelectron Adv M 9(3):512–521
Hernanz A, Gavira-Vallejo JM, Ruiz JF, Edwards GMH (2008) A comprehensive micro-Raman spectroscopic study of prehistoric rock paintings from the Sierra de las Cuerdas, Cuenca, Spain. J Raman Spectrosc 39:972–984
Hernanz A, Gavira-Vallejo JM (2021) Rock Art. In Madariaga JM (ed.) Analytical strategies for cultural heritage materials and their degradation. The Royal Society of Chemistry, pp 201–226
Helwig K, Monahan V, Poulin J, Andrews TD (2014) Ancient projectile weapons from ice patches in northwestern Canada: identification of resin and compound resin-ochre hafting adhesives. J Archaeol Sci 41:655–665
Henshilwood C, d’Errico F, Vanhaeren M, van Niekerk K, Jacobs Z (2004) Middle stone age shell beads from South Africa. Science 304:404
Henshilwood CS, d’Errico F, Watts I (2009) Engraved ochres from Middle Stone Age levels at Blombos Cave, South Africa. J Hum Evol 57:27–47
Henshilwood CS, D’Errico F, van Niekerk KL, Coquinot Y, Jacobs Z, Lauritsen SE, Menu M, Garcia-Moreno RA (2011) 100,000-year-old ochre-processing workshop at Blombos Cave, South Africa. Science 334:219–222
Henshilwood CS, d’Errico F, van Niekerk KL, Dayet L, Queffelec A, Pollarolo L (2018) An abstract drawing from the 73,000-year-old levels at Blombos Cave, South Africa. Nature 562:115–118
Hoffman DI et al (2018a) U-Th dating of carbonate crusts reveals Neandertal origin of Iberian cave art. Science 359:912–915
Hoffman DI et al. (2018b) Symbolic use of marine shells and mineral pigments by Iberian Neandertals 115,000 years ago. Sci. Adv. 4(2): eaar5255. https://doi.org/10.1126/sciadv.aar5255
Hovers E, Ilani S, Bar-Yosef O, Van der Meersch B (2003) An early case of color symbolism - ochre use by modern humans in Gafzeh Cave. Curr Anthropol 44:491–522
Hunt MA, Consuegra S, Díaz del Río P, Hurtado VM, Montero I (2011) Neolithic and Chalcolithic—VI to III millennia bc—use of cinnabar (HgS) in the Iberian Peninsula: analytical identification and lead isotope data for an early mineral exploitation of the Almadén (Ciudad Real, Spain) mining district. In: Ortiz JE, Puche O, Rabano I, Mazadiego LF (eds) History of research in mineral resources, Cuadernos del Museo Geominero, 13. Instituto Geológico y Minero de España, Madrid, pp 3–13
Iriarte E, Foyo A, Sanchez MA, Tomillo C, Setién J (2009) The origin and geochemical characterization of red ochres from the Tito Bustillo and Monte Castillo caves (northern Spain). Archaeometry 51(2):231–251
Jezequel P, Wille G, Beny C, Delorme F, Jean-Prost V, Cottier R, Breton J, Dure F, Despriee J (2011) Characterization and origin of black and red Magdalenian pigments from Grottes de la Garenne (Vallée moyenne de la Creuse-France): a mineralogical and geochemical approach of the study of prehistorical paintings. J Archaeol Sci 38(6):1165–1172
Knapp CW, Christidis GE, Venieri D, Gounaki I, Gibney-Vamvakari J, Stillings M, Photos-Jones E (2021) The ecology and bioactivity of some Greco-Roman medicinal minerals: the case of Melos earth pigments. Archaeol Anthropol Sci. https://doi.org/10.1007/s12520-021-01396-z
La Russa M F, Ruffolo S A (2021) Mortars and plasters - how to characterise mortars and plasters degradation. Archaeol Anthropol Sci. https://doi.org/10.1007/s12520-021-01405-1
Lahlil S, Lebon M, Beck L, Rousselière H, Vignaud C, Reiche I, Menu M, Paillet P, Plassard F (2012) The first in situ micro-Raman spectroscopic analysis of prehistoric cave art of Rouffignac St-Cernin, France. J Raman Spectrosc 43:1637–1643
Lancaster L C (2021) Mortars and plasters – how mortars were made. The Literary Sources. Archaeol Anthropol Sci. https://doi.org/10.1007/s12520-021-01395-0
Lewis-Williams JD (1994) Rock art and ritual: southern Africa and Beyond. Complutum 5:277–289
Lombard M (2007) The gripping nature of ochre: the association of ochre with Howiesons Poort adhesives and Later Stone Age mastics from South Africa. J Hum Evol 53(4):406–419
López E, Roldán C, Badal E, Murcia S, Villaverde V (2017) Identification of plant cells in black pigments of prehistoric Spanish Levantine rock art by means of a multianalytical approach. A new method for social identity materialization using chaîne opératoire. PLoS One 12(2):e0172225. https://doi.org/10.1371/journal.pone.0172225
Loy TH, Jones R, Nelson DE, Meehan B, Vogel J, Southon J, Cosgrove R (1990) Accelerator radiocarbon dating of human blood proteins in pigments from the Late Pleistocene art sites in Australia. Antiquity 64:110–116
Madariaga JM (2015) Analytical chemistry in the field of cultural heritage. Anal Methods 7:4848–4876. https://doi.org/10.1039/C5AY00072F
Martín-Gil J, Martín-Gil FJ, Delibes de Castro G, Zapatero P, Sarabia FJ (1995) The first known use of vermillion. Experientia 51:759–761
Martínez M J, Gavilán B, Barrios J, Montealegre L (1999) Materias primas colorants en Murciélago de Zuheros (Córdoba): caracterización y procedencia. In: Bernabeu J, Orozco T (eds.) Actes del II Congrés del Neolític a la Península Ibèrica: Saguntum (PLAV) Extra II, pp 111–16
Mas M, Jorge A, Gavilán B, Solís M, Parra E, Pérez PP (2013) Minateda rock shelters (Albacete) and post-palaeolithic art of the Mediterranean Basin in Spain: pigments, surfaces and patinas. J Archaeol Sci 40:4635–4647
Mastrotheodoros GP, Beltsios KG, Bassiakos Y (forthcoming) Pigments – iron-based red, yellow and brown ochres. Archaeol Anthropol Sci (this Topical Collection)
Mellaart J (1967) Çatal Hüyük, a Neolithic town in Anatolia. Thames and Hudson, London
Menu M (2009) L’analyse de l’art préhistorique - the analysis of prehistoric art. L’anthropologie 113:547–558
Menu M, Walter Ph (1992) Prehistoric cave painting PIXE analysis for the identification of paint “pots.” Nucl Instrum Methods Phys Research B 64:547–552
Menu M, Walter Ph (1996) Les rythmes de l’art préhistorique. Techne, Arts Préhistoriques 3:11–23
Molleson T, Comerford G, Moore A (1992) A Neolithic painted skull from Tell Abu Hureyra, northern Syria. Camb Archaeol J 2(2):230–235
Moya F, Troncoso A, Sepúlveda M, Cárcamo J, Gutiérrez S (2016) Rock art paintings in the semiarid North of Chile: a first physical and chemical approach from the Limari’s River Basin. Boletín Del Museo Chileno De Arte Precolombino 21:47–64
Murat Z (2021) Wall paintings through the ages. The medieval period (Italy, 12th-15th century). Archaeol Anthropol Sci. https://doi.org/10.1007/s12520-021-01410-4
Navarro J V, Gómez Ma L (2003) Resultados analíticos obtenidos en el estudio de pigmentos y posibles materiales colorantes de las pinturas de la Cueva de Tito Bustillo. In: Balbín R, Bueno P (eds.) El Arte Prehistórico desde los inicios del siglo XXI. Ribadesella. Asociación Cultural, pp 161–172
Nigra BT, Faull KF, Barnard H (2015) Analytical chemistry in archaeological research. Anal Chem 87(1):3–18
Ochoa B, García-Diez M, Domingo I, Martins A (2021) Dating Iberian prehistoric rock art: Methods, sampling, data, limits and interpretations. Quat Int 572:88–105. https://doi.org/10.1016/j.quaint.2020.08.048
Olivares M, Castro K, Corchón MS, Gárate D, Murelaga X, Sarmiento A, Etxebarria N (2013) Non-invasive portable instrumentation to study Palaeolithic rock paintings: the case of La Peña Cave in San Roman de Candamo (Asturias, Spain). J Archaeol Sci 40:1354–1360
Pepe CJ, Clottes M, Menu M, Walter P (1991) Le liant des peintures préhistoriques ariégeoises. Comptes Rendues De L’académie Des Sciences De Paris 312:929–934
Pérez-Arantegui J (2021) Not only wall paintings – pigments for cosmetics. Archaeol Anthropol Sci. https://doi.org/10.1007/s12520-021-01399-w
Pike AWG, Hoffmann DL, García-Diez M et al (2012) U-series dating of Paleolithic art in 11 caves in Spain. Science 336:1409–1413. https://doi.org/10.1126/science.1219957pmid:22700921
Pitarch A, Ruiz JF, Fdez-Ortiz de Vallejuelo S, Hernanz A, Maguregui M, Madariaga JM (2014) In situ characterization by Raman and X-ray fluorescence spectroscopy of post-Palaeolithic blackish pictographs exposed to the open air in Los Chaparros shelter (Albalate del Arzobispo, Teruel, Spain). Anal Methods 6:6641–6650. https://doi.org/10.1039/C4AY00539B
Pitarch A, Zilhão J, Ramos-Muñoz J, Weniger G C, Cantalejo-Duarte P, Domínguez-Bella S, d’Errico F, Fullola J (2019) Geochemical characterization of the earliest Palaeolithic paintings from southwestern Europe: Ardales Cave, Spain. In: García Atiénzar G, Barciela Gonzalez V (eds.) Sociedades prehistóricas y manifestaciones artísticas. Imágenes, nuevas propuestas e interpretaciones. Instituto Universitario de Investigación en Arqueología y Patrimonio Histórico, Universidad de Alicante, Petracos 2, pp 313–316
Pomiès M-P, Morin G, Vignaud C (1998) XRD study of the goethite-hematite transformation: application to the identification of heated prehistoric pigments. Eur J Solid State Inorg Chem 35:9–25
Pomiès MP, Menu M, Vignaud C (1999) Red Paleolithic pigments: natural hematite or heated goethite? Archaeometry 41:185–192
Pollard A, Batt C, Stern B, Young S (2007) Analytical chemistry in archaeology (Cambridge Manuals in Archaeology). Cambridge University Press, Cambridge. https://doi.org/10.1017/CBO9780511607431
Prinsloo CL, Barnard W, Meiklejohn I, Hall K (2008) The first Raman spectroscopic study of San rock art in the Ukhahlamba Drakensberg Park, South Africa. J Raman Spectrosc 39:646–654
Rampazzi L (2019) Calcium oxalate films on works of art: a review. J Cult Herit 40:195–214. https://doi.org/10.1016/j.culher.2019.03.002
Ravenscroft M (1985) Methods and materials used in Australian Aboriginal Art. ICCM Bulletin 11(3):93–109
Ridges M, Davidson I, Tucker D (2000) The organic environment of paintings on rock. In: Ward GK, Tuniz C (eds) Advances in dating Australian rock-markings. Australian, Rock Art Association, Melbourne, pp 61–70
Rifkin RF (2011) Assessing the efficacy of red ochre as a prehistoric hide tanning ingredient. J Afr Archaeol 9:131–158
Rifkin RF et al. (2015) Evaluating the photoprotective effects of ochre on human skin by in vivo SPF assessment: implications for human evolution, adaptation and dispersal. PLoS ONE 10:e0136090
Roebroeks W, Sier MJ, Kellberg Nielsen T, De Loecker D, Maria Parés J, Arps CES, Mücher HJJ (2012) Use of red ochre by early Neandertals. PNAS 109(6):1889–1894
Roldán C, Murcia-Mascarón S, Ferrero J, Villaverde V, López E, Domingo I, Martínez R, Guillem PM (2010) Application of field portable EDXRF spectrometry to analysis of pigments of Levantine rock art. Xray Spectrom 30:243–250
Roldán C, Villaverde V, Isabel Ródenas I, Murcia S (2016) A unique collection of Palaeolithic painted portable art: characterization of red and yellow pigments from the Parpalló Cave (Spain). PLoS ONE 11(10):e0163565. https://doi.org/10.1371/journal.pone.0163565
Roldán C, Murcia-Mascarós S, López-Montalvo S, Vilanova C, Porcar M (2018) Proteomic and metagenomic insights into prehistoric Spanish Levantine Rock Art. Sci Rep 8:10011. https://doi.org/10.1038/s41598-018-28121-6
Rosina P, Gomes H, Collado H, Nicoli M, Volpe L, Vaccaro C (2018) Μicro-Raman spectroscopy for the characterization of rock-art pigments from Abrigo del Águila (Badajoz – Spain). Opt Laser Technol 102:274–281
Rosso D (2017) Aproximación etnoarqueológica al uso de colorantes para el tratamiento del cabello: el caso de los Hamar (Etiopía). Pyrenae 48(2):123–149
Rosso D, d’Errico F, Zilhão J (2014) Stratigraphic and spatial distribution of ochre and ochre processing tools at Porc-Epic Cave, Dire Dawa, Ethiopia. Quat Int 343:85–99. https://doi.org/10.1016/j.quaint.2013.10.019
Rowe MW (2012) Bibliography of rock art dating. Rock Art Research 29:118–131
Salomon H, Vignaud C, Coquinot Y, Pagès-Camagna S, Pomiès M-P, Geneste J-M, David F (2008) Les matières colorantes au début du Paléolithique supérieur: Caractérisation chimique et structurale, transformation et valeur symbolique. Techné 15–21
Salomon H, Vignaud C, Coquinot Y, Beck L, Stringer C, Strivay D, D’errico F (2012) Selection and heating of colouring materials in the Mousterian level of Es-Skhul (c. 100 000 years BP, Mount Carmel, Israel). Archaeometry 54(4):698–722
Salomon H, Vignaud C, Lahlil S, Menguy N (2015) Solutrean and Magdalenian ferruginous rocks heat-treatment: accidental and/or deliberate action? J Archaeol Sci 55:100–112
Salvadori M, Sbrolli C (2021) Wall paintings through the ages. The Roman period: Republic and early Empire. Archaeol Anthropol Sci. https://doi.org/10.1007/s12520-021-01411-3
Sauvet G, Sainz CG, Sanchidrián JL, Villaverde V (2014) Europe: prehistoric rock art. In: Smith C. (eds) Encyclopedia of global archaeology. Springer, New York, https://doi.org/10.1007/978-1-4419-0465-2_1278
Schotsman E, Busacca G, Bennison-Chapman L, Lingle A, Milella M, Tibbetts B, Tsoraki Ch, Vasic M, Verapoulidou R (2020) Pigment use at Neolithic Çatalhöyük. Near East Archaeol 83(3):156–167
Sepúlveda M (2021) Making visible the invisible. A microarchaeology approach and an Archaeology of Color perspective for rock art paintings from the southern cone of South America. Quat Int 572:5–23. https://doi.org/10.1016/j.quaint.2020.05.031
Siddall R (2018) Mineral pigments in archaeology: their analysis and the range of available materials. Minerals 8(5):201
Smith DC, Bouchard M, Lorblanchet M (1999) An initial Raman microscopic investigation of prehistoric rock art in caves of the Quercy District, S. W. France. J Raman Spectrosc 30:347–354
Snethlage R, Sterflinger K (2011) Stone conservation. In: Siegesmund S., Snethlage R. (eds) Stone in architecture. Springer, Berlin, Heidelberg. https://doi.org/10.1007/978-3-642-14475-2_7
Soressi M, d’Errico F (2007) Pigments, gravures, parures: les comportements symboliques controversés des Néandertaliens. In: Vandermeersch, B., Maureille, B. (Eds.), Les Néandertaliens. Biologie et cultures. Éditions du CTHS, Paris, pp. 297–309
Spades S, Russ J (2005) GC–MS analysis of lipids in prehistoric rock paints and associated oxalate coatings from the Lower Pecos region. Texas Archaeometry 47(1):115–126. https://doi.org/10.1111/j.1475-4754.2005.00191.x
Steelman KL, Rowe MW (2012) Radiocarbon dating of rock paintings: incorporating pictographs into the archaeological record. In: McDonald J, Veth P (eds.) A companion to rock art, Blackwell, UK, pp 563–582. 1002/9781118253892.ch32
Stringer C, Gamble C (1996) En busca de los Neandertales. Crítica, Madrid
Stuart BH, Thomas PS (2017) Pigment characterization in Australian rock art: a review of modern instrumental methods of analysis. Herit Sci 5:10. https://doi.org/10.1186/s40494-017-0123-8
Švarcová S, Hradil D, Hradilová J, Čermáková Z (2021) Pigments – copper-based greens and blues. Archaeol Anthropol Sci. https://doi.org/10.1007/s12520-021-01406-0
Tortosa JEA, Gallello G, Roldán C, Cavallo G, Pastor A, Murcia-Mascarós S (2020) Characterization and sources of Paleolithic–Mesolithic ochre from Coves de Santa Maira (Valencian Region, Spain). Geoarchaeology. 1–20. https://doi.org/10.1002/gea.21821
Velo J (1984) Ochre as medicine: a suggestion for the interpretation of the archaeological record. Curr Anthropol 25(5):674
Vandenabeele P, Donais MK (2016) Mobile spectroscopic instrumentation in archaeometry research. Appl Spectrosc 70(1):27–41
Vergara F, Troncoso A (2015) Rock art, technique and technology: an exploratory study of hunter-getherer and agrarian communities in Pre-hispanic Chile (500 to 1450 CE). Rock Art Research 32(1):31–45
Velliky EC, MacDonald BL, Porr M, Conard NJ (2020) First large-scale provenance study of pigments reveals new complex behavioural patterns during the upper Palaeolithic of south-western Germany. Archaeometry. https://doi.org/10.1111/arcm.12611
Vignaud C, Salomon H, Chalmin E, Geneste J-M, Menu M (2006) The “back to back bison’s panel” of Lascaux. Access to the artist know-how by pigment analyses. L’anthropologie 110:482–499
Villa P, Pollarolo L, Degano I, Birolo L, Pasero M, Biagioni C, Douka K, Vinciguerra R, Lucejko J, Wadely L (2015) A milk and ochre paint mixture used 49,000 years ago at Sibudu. South Africa Plos ONE 10(6):e0131273. https://doi.org/10.1371/journal.pone.0131273
Vitti P (2021) Mortars and masonry – structural lime and gypsum mortars in Antiquity and Middle Ages. Archaeol Anthropol Sci. https://doi.org/10.1007/s12520-021-01408-y
Wadley L (2005) Putting ochre to the test: replication studies of adhesives that may have been used for hafting tools in the Middle Stone Age. J Hum Evol 49:587–601
Wainaright INM, Helwig K, Rolandi DS, Aschero CA, Gradin C, Podestá MM, Onetto M, Bellelli C (2002) Identification of pigments from rock painting sites in Argentina. In: L’Art avant l’histoire. La conservation de l’Art préhistorique. 10es jouernées d’études de la Section française de l’institut international de conservation, 23–24 mayo 2002, París, pp 15–24
Watchman A, Cole N (1993) Accelerator radiocarbon dating of plant-fiber binders in rock paintings from northeastern Australia. Antiquity 67:355–358
Watts I, Chazan M, Wilkins J (2016) Early evidence for brilliant ritualized display: specularite use in the Northern Cape (South Africa) between ~500 and ~300 Ka. Curr Anthropol 57(3):287–310
Williamson BS (2000) Direct testing of rock art painting pigments for traces of haemoglobin at Rose Cottage Cave, South Africa. J Archaeol Sci 27:755–762
Wolf S, Dapschauskas R, Velliky E, Floss H, Kande AW, Conard NJ (2018) The use of ochre and painting during the Upper Paleolithic of the Swabian Jura in the context of the development of ochre use in Africa and Europe. Open Archaeol 4:185–205
Zilhão J (2020) The origins of art. In: Domingo I, Palomo A (eds) First art Artists from Prehistory. Museu d’Arqueologia de Catalunya, Barcelona, pp 34–43
Zilhão J, Angelucci D, Badal-García E, d’Errico F, Daniel F, Dayet L, Douka K, Higham TFG, Martínez-Sánchez MJ, Montes-Bernárdez R, Murcia-Mascarós S, Pérez-Sirvent C, Roldán-García C, Vanhaeren M, Villaverde V, Wood R, Zapata J (2010) Symbolic use of marine shells and mineral pigments by Iberian Neandertals. Proc Natl Acad Sci U S A 107(3):1023–1028
Zipkin A, Hanchar J, Brooks A, Grabowski M, Thompson J, Chidebvu E (2015) Ochre fingerprints: distinguishing among Malawian mineral pigment sources with homogenized ochre chip LA-ICPMS. Archaeometry 57(2):297–317
Funding
This research has been supported by several research grants directed by Inés Domingo: HAR2016-80693-P funded by the Spanish Ministry of Science, Innovation and Universities and the ERC-CoG LArcHer project, funded by the European Research Council (ERC) under the European Union’s Horizon 2020 research and innovation programme (grant agreement No 819404).
Author information
Authors and Affiliations
Contributions
Both authors contributed equally to this work.
Corresponding author
Ethics declarations
Competing interests
The authors declare no competing interests.
Additional information
Publisher’s note
Springer Nature remains neutral with regard to jurisdictional claims in published maps and institutional affiliations.
This article is part of the Topical Collection on Mortars, plasters and pigments: Research questions and answers
Rights and permissions
Open Access This article is licensed under a Creative Commons Attribution 4.0 International License, which permits use, sharing, adaptation, distribution and reproduction in any medium or format, as long as you give appropriate credit to the original author(s) and the source, provide a link to the Creative Commons licence, and indicate if changes were made. The images or other third party material in this article are included in the article's Creative Commons licence, unless indicated otherwise in a credit line to the material. If material is not included in the article's Creative Commons licence and your intended use is not permitted by statutory regulation or exceeds the permitted use, you will need to obtain permission directly from the copyright holder. To view a copy of this licence, visit http://creativecommons.org/licenses/by/4.0/.
About this article
Cite this article
Domingo, I., Chieli, A. Characterizing the pigments and paints of prehistoric artists. Archaeol Anthropol Sci 13, 196 (2021). https://doi.org/10.1007/s12520-021-01397-y
Received:
Accepted:
Published:
DOI: https://doi.org/10.1007/s12520-021-01397-y