Abstract
The linear characteristics of polyethylene terephthalate, such as index of refraction, spectral transmittance and transverse distribution of phase and polarization distortions, have been measured. Spectrum broadening of laser pulses (intensity over 1.3 ТW cm−2) after propagation through a 0.7 mm thick sample has been demonstrated in experiment. Taking into consideration almost unlimited aperture, submillimeter thickness and the low cost of polyethylene terephthalate, the obtained results demonstrate that it is a prospective material to be used for spectrum broadening and subsequent time compression of petawatt laser pulses to single cycle regime (Mourou et al 2014 Single cycle thin film compressor opening the door to zeptosecond-exawatt physics Eur. Phys. J. Spec. Top. 223 1181–8).
Export citation and abstract BibTeX RIS
1. Introduction
With the usе of the chirped pulse amplification (CPA) [2] technique it became possible to obtain laser radiation with peak power exceeding 1 PW. Pulse energy in such laser systems usually amounts to tens of joules and pulse duration is tens of femtoseconds [3, 4]. A further increase of laser pulse peak power is possible through energy enhancement or time compression. The first approach demands creation of costly large-aperture amplifiers and high-power pump lasers. The alternative approach implies additional pulse compression due to spectrum broadening as a result of the phase self-modulation effect and subsequent spectrum phase correction [5, 6]. Thin (less than 1 mm) transparent dielectrics (glasses or crystals) must be used as a nonlinear medium, where phase self-modulation occurs, in laser systems with ultra-high-power pulses with an intensity of 1 TW сm−2 and more. It is important to note that this method has been successfully verified in experiments [6, 7], and one of the recent experimental results was a two-fold reduction of radiation duration (from 43 to 20 fs) with energy 28 mJ and a peak intensity of 3 TW cm−2 [7]. However the technique is limited by the spatial beam variations, which impose the use of optical elements like diverging lens that can compensate for the lack of uniformity. This problem disappears with the PW laser that, if they are well designed, exhibits a remarkable top hat beam quality as seen in [1].
The remaining difficulty for achieving time compression of petawatt (PW) radiation resides in the fabrication of nonlinear elements having an aperture over 10 cm and thickness less than 1 mm. The authors of [1] proposed to use nonlinear elements made of plastic, which solves the problem of large aperture and small thickness. However, the linear and nonlinear properties of plastics were not investigated.
In the present work we study the possibilities of using a transparent plastic—polyethylene terephthalate—for spectrum broadening of pulses with intensities of about 1 TW cm−2, which corresponds to the intensity of unfocused PW laser pulses.
2. Linear optical properties of polyethylene terephthalate
Polyethylene terephthalate is a solid, transparent body in amorphous state and a white nontransparent body in crystalline state. We will demonstrate how consumer sheet polyethylene terephthalate may be used in optics. For this, we will present measurement results of optical thickness inhomogeneity, spectrum transmittance and depolarization.
A sample with an aperture of 250 × 250 mm has been cut from a sheet having size 1 × 2 m and thickness 0.7 mm. The interferometric measurement results of the optical thickness deviation are presented in figure 1(a). Here, the deviation of optical thickness is a difference between the local and mean values of the optical thickness. According to figure 1(a), the rms deviation of the optical thickness of the sample with an aperture of 250 mm is 0.73 μm. The optical thickness distribution contains technology-related periodic structures and a cylindrical component. The phase aberrations (a cylindrical lens, in particular), arising as a result of laser pulse propagation through such a sample, may be partially compensated by means of adaptive mirrors (see figure 1(b)). Note that after correction of the cylindrical component, the rms deviation of the optical thickness in figure 1(c) decreases to 0.45 μm, and in the region of 100 mm encircled in figure 1(a), the rms deviation of the optical thickness is only 0.3 μm.
Figure 1. (a) Distribution of optical thickness deviation of the polyethylene terephthalate sample over an aperture of 250 mm; (b) cylindrical component; (c) distribution of optical thickness deviation with correction of the cylindrical component.
Download figure:
Standard image High-resolution imageThe spectral transmittance of a 0.5 mm thick plastic sample was investigated using a broadband light source. The measurement results are presented in figure 2. The plastic has the homogeneous transmission coefficient (the rms deviation is 0.2%) at the measured spectral range. The energy losses in the sample are mainly due to the Fresnel reflection. The measured index of refraction of the studied polyethylene terephthalate sample was n = 1.573 for a wavelength of 0.633 μm. The calculated value of the transmission coefficient is 90.3% and it agrees with the measured value 90.2%.
Figure 2. Transmission coefficient of a 0.5 mm thick plane–parallel polyethylene terephthalate plate as a function of wavelength.
Download figure:
Standard image High-resolution imageWe placed the sample between crossed polarizers and measured the depolarization introduced to the passing laser beam by the sheet of polyethylene terephthalate. A semiconductor laser at a wavelength of 1.06 μm was used as a source and calcite wedges as polarizers. We moved the sample in the transverse direction and rotated it about the z axis, simultaneously measuring its orientation relative to the radiation polarization. The depolarization level was almost the same at all points of the cross-section of each sample and strongly depended on the plastic orientation relative to the polarization. This is evidently associated with the uniformly distributed stresses in the plastic that were formed in the course of its fabrication. The maximum depolarization degree (the eigenpolarizations of the sample were oriented 45° to the input polarization) is slightly varied for different samples and did not exceed 7%. When the eigenpolarizations of the plastic were parallel to the initial polarization, the measured power of the depolarized component was 0.02%. In this case, the radiation depolarization in polyethylene terephthalate may be neglected.
3. Pulse spectrum broadening during propagation in polyethylene terephthalate
Our consideration of the nonlinear properties of polyethylene terephthalate will be restricted by cubic nonlinearity and the related effect of phase self-modulation and spectrum broadening. The physics of these phenomena is well studied [5] and is based on the fact that the index of refraction changes under the action of intense light and the optical pulse propagating in the medium accumulates a nonlinear phase (or B-integral, see equation (1)):
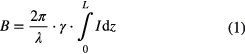
where I is field intensity, γ is cubic nonlinearity, λ is radiation wavelength, and L denotes the thickness of the nonlinear medium. By correcting the nonlinear phase using external correctors it is possible to reduce optical pulse duration. The spectrum broadening of the medium is defined by the value of the B-integral as well as by the residual uncompensated phase in the initial pulse. It is worthwhile noting that the process of spectrum broadening of pulses with equal intensity is different in the cases with and without phase modulation.
The nonlinear properties of polyethylene terephthalate were examined using the radiation at the front end of the petawatt femtosecond laser complex PEARL [4] with the following parameters: pulse energy 1 mJ, duration 70 fs, and intensity 1.3 TW cm−2. A spectrometer recording the radiation frequency spectrum was placed immediately behind the studied sample. The spectra of the input radiation and of the radiation that has passed through the silica sample 1.75 mm thick and the polyethylene terephthalate sample 0.7 mm thick are shown in figure 3.
Figure 3. Spectral intensity (1) of the initial pulse, (2) of the pulse transmitted through samples of polyethylene terephthalate 0.7 mm thick and (3) silica 1.7 mm thick.
Download figure:
Standard image High-resolution imageThe magnitude of the B-integral in silica (γ = (2.5÷3)·10−7 GW cm−2) at an intensity of 1.3 TW cm−2 is 4.2. It is clear from figure 3 that the broadened spectra in silica and in polyethylene terephthalate have the same topology, and the spectrum broadening in these samples differs only insignificantly. Consequently, in conformity with (1), the ratio of cubic nonlinearities of these two media is equal approximately to the ratio of sample thicknesses, i.e. γ of polyethylene terephthalate is approximately two times more for silica and the estimated value is (5÷6)·10−7 cm2 GW−1. Additional studies are required to find a more accurate value of cubic nonlinearity, but they are outside the scope of the present work. It is important that no visible damage of the plastic sample was observed in the course of the experiments on spectrum broadening of femtosecond radiation with a peak intensity exceeding 1 TW сm−2. The number of laser shots exceeded 100.
4. Conclusion
We have demonstrated that polyethylene terephthalate exhibits the qualities necessary to be used for spectrum broadening of pulses with a peak intensity of about 1 TW cm−2. It is a good candidate to obtain single-cycle PW laser pulses at a double stage nonlinear compressor [1] due to insignificant phase aberrations, negligibly small depolarization and losses, large value of cubic nonlinearity, and stability at the action of intense (over 1 TW сm−2) laser beams. Moreover, it has a number of advantages such as almost unlimited aperture, small thickness (samples with a thickness of 0.5 mm and less are commercially available), and low cost.
Acknowledgment
This work was supported in part by the Ministry of Education and Science of the Russian Federation under Contract No. 14.Z50.31.0007 and Program to Increase the Competitiveness of NUST «MISiS» among the World Leading Scientific and Educational Centers (grant № K1-2014-008). Gérard Mourou would like to acknowledge the Ecole Polytechnique et le Commissariat à l' Energie Atomique et aux Energies Alternatives as well as the Russian Megagrant Program № 11.G34.31.0011 for their invaluable support.