Abstract
In this study, a coupled multi-species transport and chemical equilibrium model has been established. The model is capable of predicting time dependent variation of pore solution and solid-phase composition in concrete. Multi-species transport approaches, based on the Poisson–Nernst–Planck (PNP) theory alone, not involving chemical processes, have no real practical interest since the chemical action is very dominant for cement based materials. Coupled mass transport and chemical equilibrium models can be used to calculate the variation in pore solution and solid-phase composition when using different types of cements. For example, the physicochemical evaluation of steel corrosion initiation can be studied by calculating the molar ratio of chloride ion to hydroxide ion in the pore solution. The model can, further, for example, calculate changes of solid-phase composition caused by the penetration of seawater into the concrete cover. The mass transport part of the model is solved using a non-linear finite element approach adopting a modified Newton–Raphson technique for minimizing the residual error at each time step of the calculation. The chemical equilibrium part of the problem is solved by using the PHREEQC program. The coupling between the transport part and chemical part of the problem is tackled by using a sequential operator splitting technique and the calculation results are verified by comparing the elemental spacial distribution in concrete measured by the electron probe microanalysis (EPMA).










Similar content being viewed by others
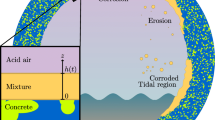
References
Johannesson B (2000) Transport and sorption phenomena in concrete and other porous media. Dissertation, Lund university
Samson E, Marchand J, Robert JL, Bournazel JP (1999) Modelling ion diffusion mechanisms in porous media. Int J Numer Methods Eng 46:2043–2060
Nonat A (2004) The structure and stoichiometry of C–S–H. Cem Concr Res 34:1521–1528
Purkhurst DL et al (1999) User’s guide to PHREEQC (ver. 2). Water Resources Investigations Report 99-4259, Denver
Hosokawa Y, Yamada K, Johannesson B, Nilsson LO (2006) Models for chloride ion bindings in hardened cement paste using thermodynamic equilibrium calculations. In: RILEM 2nd international symposium on advance in concrete through science and engineering, Quebec, Canada
Hosokawa Y, Yamada K, Nonat A (2009) A thermodynamic model with the electrical double layer on the C–S–H particle surface to predict liquid-solid compositions of hardened cement paste. J Res Taiheiyo Cem Corp 157:3–10
Goto S et al (1982) Pore-size distribution and diffusion of ions in hardened cement paste (in Japanese). Cement Sci Concrete Technol 36:49–52
Johannesson B, Yamada K, Nilsson L-O, Hosokawa Y (2007) Multi-species ionic diffusion in concrete with account to interaction between ions in the pore solution and the cement hydrates. Mater Struct 40(7):651–665
Truesdell AH, Jones BF (1974) WATEQ, a computer program for calculating chemical equilibria of natural waters. J Res US Geol Surv 2:233–274
Ball JW, Nordstrom DK (1991) WATEQ4F—user’s manual with revised thermodynamic data base and thest cases for calculating speciation of major, trace and redox elements in natural waters. US Geol Surv Open-File Rep 185:90–129
Lothenbach B, Matschei T, Moschner G, Glasser FP (2008) Thermodynamic modelling of the effect of temperature on the hydration and porosity of Portland cement. Cem Concr Res 38(1):1–18
Reardon EJ (1990) Ion interaction model for the determination of chemical equilibria in cement/water systems. Cem Concr Res 20:175–192
Birnin-Yauri UA, Glasser FP (1998) Friedel’s salt, Ca2Al(OH)6(Cl,OH)2H2O: its solid solutions and their role in chloride binding. Cem Concr Res 28(12):1713–1723
Bénard A, Rose J, Nazemann JL, Proux O, Trotignon L, Borschneck D, Nonat A, Chateau L, Bottero JY (2009) Modelling of Pb release during Portland cement alteration. Adv Cem Res 21(1):1–10
Lothenbach B, Winnefeld F (2006) Thermodynamic modelling of the hydration of Portland cement. Cem Concr Res 36(2):209–226
Pöllmann H (1992) Incorporation of SO4 2−, CO3 2− and OH− in hydration products of tricalciumaluminate. In: International congress on the chemistry of cement, New Dehli, vol 4, pp 361–369
Pöllmann H (1986) Solid solution of complex calcium aluminate hydrates containing Cl−, OH− and CO3 2−-anions. In: Proceedings of the 8th international symposium on the chemistry of cement, Rio de Janeiro, vol 3, pp 300–306
Hong SY, Glasser FP (1999) Alkali binding in cement pastes Part I. The C–S–H phase. Cem Concr Res 29:1893–1903
Hirao H, Yamada K, Takahashi H, Zibara H (2005) Chloride binding of cement estimated by binding isotherms of hydrates. J Adv Concr Technol 3(1):77–84
Heath TG, Ilett DJ, Tweed CJ (1996) Thermodynamic modeling of the sorption of radioelements onto cementitious materials. Mater Res Soc Symp Proc 412:443–449
Sugiyama D, Fujita T (2004) Chemical alteration of calcium silicate hydrates in saline groundwater—mechanism of sorption of Na on C–S–H and effect of NaCl on leaching of Ca from C–S–H. Komae Research Laboratory, Rep. No. T03056 (in Japanese)
Borkovec M, Westall J (1983) Solution of the Poisson–Boltzmann equation for surface excesses of ions in the diffuse layer at the oxide-electrolyte interface. J Electroanal Chem 150:325–337
Labbez C, Jönsson B, Pochard I, Nonat A, Cabane B (2006) Surface charge density and electrokinetic potential of highly charged minerals: experiments and Monte Carlo simulations on calcium silicate hydrate. J Phys Chem 110(18):9219–9230
Johannesson B (1999) Diffusion of a mixture of cations and anions dissolved in water. Cem Concr Res 29:1261–1270
Mori D, Yamada K, Hosokawa Y, Yamamoto M (2006) Applications of electron probe microanalyzer for measurement of Cl concentration profile in concrete. J Adv Concr Technol 4(3):369–383
Dean JA (ed) (1999) Lange’s handbook of chemistry, 15th edn. McGraw-Hill, New York
Mackin JE, Aller RC (1983) The infinite dilution diffusion coefficient for Al(OH)4 − at 25°C. Geochim Cosmochim Acta 47(5):959–961
Hoshino S, Yamada K, Hirao H (2006) XRD/rietveld analysis of the hydration and strength development of slag and limestone blended cement. J Adv Concr Technol 4(3):357–367
Yamada K, Ogawa S, Toriiminami K, Uemura U, Kobayakawa M (2002) A study on chloride penetration under a dry-wet condition considering the chloride binding capacity of cement. In: Third international RILEM workshop on testing and modelling chloride ingress into concrete, Madrid, Spain, pp 433–446
Richardson IG, Groves GW (1993) Microstructure and microanalysis of hardened ordinary Portland cement pastes. J Mater Sci 28(1):265–277
Diamond S (1986) Chloride concentrations in concrete pore solutions resulting from calcium and sodium chloride admixtures. Cem Concr Aggreg 8(2):97–102
Glass GK, Buenfeld NR (1997) The presentation of the chloride threshold level for corrosion of steel in concrete. Corros Sci 39(5):1001–1013
Dickson AG, Goyet C (1994) Handbook of methods for the analysis of the various parameters of the carbon dioxide system in sea water; version 2. ORNL/CDIAC-74
Neville AM (2000) Properties of concrete, 4th edn. Peason Education, Essex, pp 514–516
Yamaji T, Nakano S, Hamada H, Yamada K (2008) Results of concrete quality in existing structures exposed under marine environment for long term. In: Proceedings of the Japan Concrete Institute, vol 30, pp 609–614 (in Japanese)
Acknowledgments
We would like to thank Dr. André Nonat (Université de Bourgogne) for his helpful discussion and advisement on the C–S–H model and Dr. Toru Yamaji for his kind approval to use several measurement results of marine structures.
Author information
Authors and Affiliations
Corresponding author
Rights and permissions
About this article
Cite this article
Hosokawa, Y., Yamada, K., Johannesson, B. et al. Development of a multi-species mass transport model for concrete with account to thermodynamic phase equilibriums. Mater Struct 44, 1577–1592 (2011). https://doi.org/10.1617/s11527-011-9720-2
Received:
Accepted:
Published:
Issue Date:
DOI: https://doi.org/10.1617/s11527-011-9720-2