Volatile Organic Compounds Gas Sensors Based on Molybdenum Oxides: A Mini Review
- 1College of Engineering and Technology, Southwest University, Chongqing, China
- 2Chongqing Electric Power Research Institute, State Grid Chongqing Electric Power Company, Chongqing, China
- 3College of Materials Science and Engineering, Chongqing University, Chongqing, China
As a typical n-type semiconductor, MoO3 has been widely applied in the gas-detection field due to its competitive physicochemical properties and ecofriendly characteristics. Volatile organic compounds (VOCs) are harmful to the atmospheric environment and human life, so it is necessary to quickly identify the presence of VOCs in the air. This review briefly introduced the application progress of an MoO3-based sensor in VOCs detection. We mainly emphasized the optimization strategies of a high performance MoO3, which consists of morphology-controlled synthesis and electronic properties functional modification. Besides the general synthesis methods, its gas-sensing properties and mechanism were briefly discussed. In conclusion, the application status of MoO3 in gas-sensing and the challenges still to be solved were summarized.
Introduction
Volatile organic compounds (VOCs) mainly come from the exhaust gases generated by fuel combustion and transportation, as well as emissions from building materials, decorative materials, and furniture. People can suffer headaches, nausea, and even more severe issues such as convulsions and comas when exposed to a certain concentration of VOCs (Chu et al., 2010; Sui et al., 2015). Moreover, many carcinogens that damage the liver, kidneys, brain, and nervous system were found in VOCs. Therefore, the problem of air pollution by VOCs has attracted extensive attention in many countries (Yang et al., 2018; He S. H. et al., 2019). Currently, there are two common techniques—photo ionization detector (PID) and flame ionization detector (FID)—to detect VOCs, however, the application of these methods in industry are limited due to the relatively high cost and complicated maintenance. Considering the characteristics of small size, low cost, and convenient fabrication, semiconductor gas sensor technology plays an important role in many fields (Lu et al., 2018; Xiao et al., 2018; Zhang D. Z. et al., 2018; Zhang Q. Y. et al., 2018; Zhou et al., 2018c, 2019; Wang et al., 2019a; Wei et al., 2020), so it is reasonable to propose the employment of a gas sensor to realize the online monitoring of VOCs.
As a typical n-type semiconductor material with a suitable band gap (2.39–2.9 eV) (Yan et al., 2016), MoO3 has attracted wide attention because of its distinctive gas sensing performances in the detection of many gases (Liu et al., 2015; Xia et al., 2016; Li, 2017; Zhou et al., 2017; Yang et al., 2018). Researchers have been devoted to designing nanomaterials with more suitable properties, and two methods have proved effective through unremitting efforts. One is to synthesize materials with larger specific surface areas, which is attributed to the conclusion that the micromorphology features have an impact on the gas-sensitive process (Zhou et al., 2018b; Zhu et al., 2018; Wang et al., 2019b; Wei Z. J. et al., 2019). Considering the changes in the materials that are caused by doping and compounding or the catalytic effect of the introduced material on the sensing process, it is also desirable to improve the properties of the materials by introducing other elements or substances (Mousavi-Zadeh and Rahmani, 2018; Zhou et al., 2018a; Wang et al., 2019c; Xu et al., 2019). Therefore, the optimization strategies of MoO3 based on controllable morphology synthesis and functional modification were comprehensively summarized in this mini review. Besides, several typical synthesis pathways of MoO3 nanomaterials, as well as the gas-sensing performances and mechanism to VOCs, were introduced.
Synthesis Methods of MOO3
The preparation of materials with more useful properties has always been a research hotspot in the field of gas detection, and has attracted extensive attention from scholars. In recent years, material preparation craft is constantly updated and developed with the emergence of new technology. At present, solid phase method, liquid phase method, and template method are mainly employed to synthesize MoO3 materials with admirable performance.
The process of preparing materials by solid phase method is to transform the solid phase raw materials into target powders. Using ammonium molybdate as raw material, Qin et al. (2017) successfully obtained MoO3 nanoplate arrays in the air through a solid phase chemical synthesis route. By hydrolyzing a mixture of one or more soluble metal salts solution and then evaporating and sublimating them, the liquid phase method adopts a series of processes to separate the solute from the solvent, where finally the nanoparticles with uniform shape are produced by crystallizing metal ions. Nowadays, spray pyrolysis technique, sol-gel route, and hydrothermal method have been reported as common liquid phase methods for the preparation of MoO3 materials. Sau et al. (2019) used the sol-gel method to heat the solution after the molybdenum source was fully dissolved to a gelatinous state under specific PH (7–8) conditions. Finally they prepared α-MoO3 nanoparticles through annealing and drying. Pandeeswari and Jeyaprakash (2014) successfully obtained MoO3 thin films with a thickness of 520 nm on a glass substrate maintained at 250°C by spray pyrolysis route. Zhu et al. (2019) synthesized hollow MoO3 microcages by a facile one-step hydrothermal process, which had gone through four steps of heating, cooling, centrifugation, and washing. The template method is designed to generate nanomaterials based on the template of appropriate structure, which can effectively influence the growth direction and morphology of the materials. Zhang et al. (2017) deposited MoS2@MnCO3 powder based on an MnCO3 template, and prepared MoS2 by adding hydrochloric acid to remove MnCO3. Finally, they obtained hierarchical MoO3 microboxes by calcining MoS2 powder.
Optimization Strategies OF MOO3
The sensitivity of the gas sensors is closely related to the changes in the resistance that is attributed to the adsorption and desorption of target gas molecules on the surface of materials, which implies that the gas-sensing properties mainly depend on their own electronic characteristics (carrier concentration, energy band structure, etc.) and morphological characteristics (specific surface area, aperture, etc.). Based on this, the main strategies to enhance the gas-sensing performances of materials are morphology control and electronic properties improvement.
Controllable Synthesis of Diversified MoO3
In recent years, the production of MoO3 gas-sensitive materials with high quality morphology has become an important research approach for performance enhancement. In this regard, MoO3 gas-sensitive materials with different morphologies and dimensions have been prepared through different methods to conduct gas-sensing experiments. In this section, we review and summarize the excellent achievements of MoO3 morphology control and several typical morphologies are shown in Figures 1A–C.
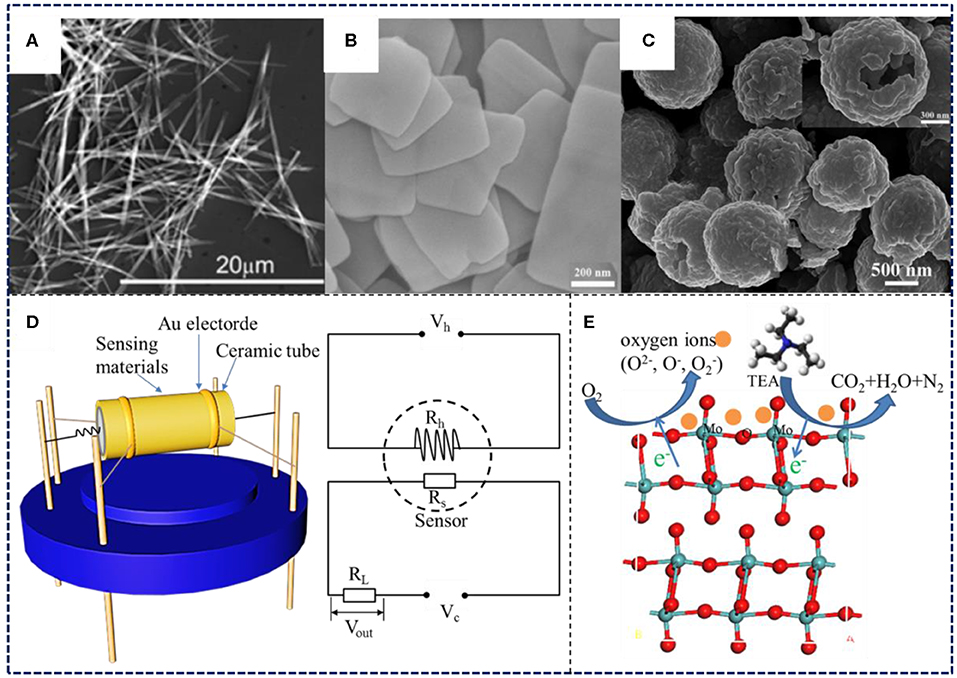
Figure 1. (A) Nanoribbons. Reprinted with permission from Kwak et al. Copyright (2019) American Chemical Society. (B) Nanosheets. Reprinted with permission from Shen et al. Copyright (2019) American Chemical Society. (C) Hollow microspheres. Reprinted with permission from Zhang et al. Copyright (2019) American Chemical Society. (D) Schematic diagram of sensor and detection circuit. (E) Gas sensing mechanism.
One-dimensional (1D) structures, such as nanofibers, nanorods, and nanoribbons, have limited specific surface areas, but their reaction sites are directly exposed to external environments, leading to the susceptive variation of interface ion transport when some changes occur to environmental factors. Hence, 1D material has the potential to be applied as excellent sensors. The remarkable selectivity and response-recovery characteristics of α-MoO3 nanorods gas sensors to triethylamine was reported (He S. H. et al., 2019). Besides, the rapid adsorption/desorption processes were confirmed to be the reason for prominent gas-sensing characteristics. By optimizing reaction time and pulse temperature in hydrothermal reaction, Mandal et al. (2019) synthesized uniform MoO3 nanobelts and MoO3 nanofibers. The experimental results illustrated that the enhanced ethanol detection performances of nanofibers could be ascribed to the larger specific surface area and surface defects compared with the nanowires.
Materials with two-dimensional structures such as nanoplates and nanosheets have a larger specific surface area, which further improves the gas-sensitive responses. The prominent electron depletion layer that derived from the thin morphologic features of nanoplates was verified as beneficial due to the ultrahigh gas-sensing performances. Moreover, the porous structure caused by the combination of nanoplates could effectively facilitate gas diffusion on the surface of MoO3, providing convenience for the gas-sensing process (Cho et al., 2014). The study of Jiang et al. (2018) demonstrated that MoO3 microsheets with a large number of oxygen vacancies exhibit superior sensing properties, and the strong reaction between adsorbed oxygen and target gases to be confirmed was also an important factor to promote the gas-sensing performances.
Three-dimensional structures with large specific surface areas are generally assembled from low-dimensional structures, which have more reaction sites for gas adsorption, leading to better gas-sensitive responses and lower gas detection limits. Furthermore, more gas diffusion channels are provided by the assembled porous structure, which are conducive to gas sensitivity reaction. Common three-dimensional structures of MoO3 include nanoarrays, nanoflowers, and nanospheres. For example, Ji et al. (2019a) synthesized three hierarchical MoO3 flower-like samples with different petal shapes and found that the material with the thinnest petals had the most outstanding gas-sensing performance. Specifically, the thin nanosheets assembled flower-like MoO3 has a larger specific surface area that provides more potential for gas-sensitive reactions, and the thinness allows electrons to transfer rapidly across the surface, which implied the procedure of gas-sensing became easier. In addition, the curved edges of thin petals make it harder for gas molecules to leave the surface, facilitating the full process of adsorption. Cong et al. (2016) proved the sensors based on MoO3 nanoarrays assembled from a large number of long nanorods exhibited more reactive sites and more active surface electrical properties than the nanoarrays with fewer nanorods.
Functional Modification Methods
Functional modification is of great significance to improving the properties of gas-sensitive materials. In the present studies, the modification of MoO3 mainly includes two approaches of element doping and multi-component compounding, which have been proven to be effective methods in optimizing the electronic properties of materials. In this section, the advances of MoO3 modification research were briefly summarized.
Metal doping is an alternative approach to acquire the modified characteristics of MoO3. On the one hand, suitable metal doping can effectively reduce the activation energy of chemisorption reaction for the measured gases. On the other hand, the metal elements play the role of the catalytic activity center, leading to the optimization of the gas-sensing performances. Scholars have studied the influence of different doping elements on the properties of MoO3 materials. For instance, Cr-doped MoO3 nanorods had more oxygen vacancy induced by doping, which is meaningful for the promotion of sensitivity (Li et al., 2019). Similar results were obtained when the W element was doped in MoO3 nanobelts (Li et al., 2017b). As for the dope of Ni, not only did more adsorbed oxygen that can promote the change of resistance in the sensing process appear on the surface of MoO3, but also the morphology changed with the increase of the doping amount, which is related to the inhibition of lattice growth by the introduction of doped Ni elements (Jiang et al., 2019). Yang et al. (2017) prepared Zn-doped MoO3 nanobelts using the hydrothermal method. They found that the doped zinc caused the reduction of the band gap of MoO3 and increased the amount of adsorbed ethanol molecules, which contributed to the excellent performance.
Many researchers have been devoted to the preparation of hybrid structures that affected properties such as grain boundary barrier, energy band, carrier concentration, and depletion layer, thus improving the performance of gas sensing. For instance, the Au nanoparticle with a larger work function than MoO3 received electrons from MoO3 nanosheets, leading to the appearance of electron depletion layer at the Au/MoO3 Schottky junction, and the enhanced ethanol detection capabilities were attributed to the resulting high resistance (Yan et al., 2016). Considering Pt nanoparticles combined MoO3 nanobelts, the superior selectivity to formaldehyde was conducted, which was ascribed to the catalytic effect of loaded particles on formaldehyde during the surface gas sensing process (Fu et al., 2019). As for the RuO2 nanoparticles modified MoO3 nanobelts, oxygen vacancies produced on the surface, creating more adsorption-desorption sites for gas molecules (Wei Q. et al., 2019). With regard to heterostructure, Li et al. (2018) synthesized the CoMoO4/MoO3 nanostructure with p-n heterojunction using the dipping-annealing method. The enhancement of the adsorption to oxygen by p-type CoMoO4 and the barrier formed at the p-n junction were verified to be favorable to the improved gas response.
Above all, previous studies have focused on the optimization of MoO3 through synthesizing different samples with multiple morphologies, the doping of transition metals such as Cr, W, Ag, Au, Fe, Zn, and Ni, and decorating with other nanomaterials (Au nanoparticles, RuO2 nanoparticles, Fe2O3 nanoparticles, CoMoO4 nanoparticles, NiCo2O4 nanosheets, etc.). Thus, in order to improve the gas sensitivity of MoO3, many potential materials for the modification of innovative synthesis methods with controllable morphology need to be explored.
Gas-Sensing Application of MOO3
Gas-sensing Mechanism of MoO3
The theory of sensitivity generated by changes in material resistance during the gas-sensing process has been widely accepted by scholars in the investigation of the gas-sensitive mechanism of metal oxides. As shown in Figure 1E, the reaction between the target gas molecules and the adsorbed oxygen ions (, O−, O2−) on the surface of the gas-sensitive materials leads to the change of the electrical conductivity, which is key to detecting the corresponding response (Li et al., 2015). MoO3 is an n-type semiconductor with electrons as internal carriers. Mass of oxygen molecules in the air tends to be adsorbed by the MoO3, forming adsorbed oxygen ions accompanied by the acceptance of electrons from the conduction band of MoO3. Thus, the electron depletion layer was formed on the surface of MoO3, which caused the increase of resistance (Ji et al., 2019a). The specific process can be expressed by the following equation:
When the MoO3 sensor was exposed to the atmosphere of the target gas, the adsorbed oxygen ions underwent a reduction reaction. Further, electrons are released back into the conduction band of MoO3, and the depletion layer narrows, which results in a decrease in the resistance of the material and completes the whole gas sensing process. Take triethylamine for example, this process can be expressed as the following equation (Wei Q. et al., 2019).
Gas Sensing Properties of MoO3
Nowadays, many researchers focus on the application of MoO3 materials in gas sensors, while the ultimate goal of the investigation is to obtain higher performance MoO3-based sensing materials. Notably, MoO3 with prominent gas-sensing properties has been proven to be an alternative sensing material to detect VOCs. We summarized the representative research on VOCs detection, which mainly focused on the use of formaldehyde (HCHO), methanol (CH3OH), ethanol (CH3CH2OH), xylene (CH3C6H4CH3), trimethylamine ((CH3)3N), and triethylamine ((C2H5)3N), and listed them in Table 1. Figure 1D shows the structure diagram of the side heat sensor and the gas-sensing test circuit. The sensing-materials were coated on the alumina ceramic tube. A Ni-Cr resistance wire that could conveniently control the current was inserted in the coating tube and the change of resistances was tested by electrodes. The gas response (S) of MoO3 based sensors to reducing gas is calculated by S = Ra/Rg, while to oxidizing gas it is S = Rg/Ra (Jiang et al., 2019).
Conclusion
This mini review focused on the latest advances in synthetic methods, morphological control, functional modification, and gas-sensing application including properties and mechanism of MoO3 materials in the detection of VOCs. The studies of morphologically-controlled synthesis proved that MoO3 with a high specific surface area possesses superior gas-sensing performances and provided reference experience for further MoO3 gas-sensing material design. Further, appropriate element doping or material hybridization could improve properties of MoO3, such as energy band gap and adsorbed oxygen content, which is advantageous to the gas sensing process. Scholars have made great efforts to develop more efficient MoO3-based VOCs sensors and have shown objective achievement, but there are still challenges in practical application. The design of porous structures or hierarchical structures with more reactive gas pathways and reaction sites to further improve the specific surface area of MoO3 material is an issue that needs to be further explored. In addition, more alternative modification materials should be selected through experimental verification. All of these issues should continue to be addressed to obtain MoO3-based materials with a higher response, better selectivity, superior stability, and lower operating temperature. Finally, the gas-sensing mechanism was not complete. By combining theoretical calculation and analysis, the changes of electronic properties at the micro level should be analyzed, which will allow for further understanding of the nature of gas sensing and provide guidance for designing MoO3 materials with better gas-sensing performances in the future.
Author Contributions
All authors listed have made a substantial, direct and intellectual contribution to the work, and approved it for publication.
Funding
This work has been supported in part by the National Natural Science Foundation of China (No. 51507144), Fundamental Research Funds for the Central Universities (No. XDJK2019B021), the Chongqing Science and Technology Commission (CSTC) (No. cstc2016jcyjA0400), and the project of China Scholarship Council (CSC).
Conflict of Interest
The authors declare that the research was conducted in the absence of any commercial or financial relationships that could be construed as a potential conflict of interest.
References
Cho, Y. H., Ko, Y. N., Kang, Y. C., Kim, I. D., and Lee, J. H. (2014). Ultraselective and ultrasensitive detection of trimethylamine using MoO3 nanoplates prepared by ultrasonic spray pyrolysis. Sens. Actuat. B Chem. 195, 189–196. doi: 10.1016/j.snb.2014.01.021
Chu, X. F., Liang, S. M., Sun, W. Q., Zhang, W. B., Chen, T. Y., and Zhang, Q. F. (2010). Trimethylamine sensing properties of sensors based on MoO3 microrods. Sens. Actuat. B Chem. 148, 399–403. doi: 10.1016/j.snb.2010.05.049
Cong, S. R., Sugahara, T., Wei, T. T., Jiu, J. T., Hirose, Y., Nagao, S., et al. (2016). Diverse adsorption/desorption abilities originating from the nanostructural morphology of VOC gas sensing devices based on molybdenum trioxide nanorod arrays. Adv. Mater. Interf. 3:1600252. doi: 10.1002/admi.201600252
Fu, X. X., Yang, P. Y., Xiao, X. F., Zhou, D., Huang, R., Zhang, X. H., et al. (2019). Ultra-fast and highly selective room-temperature formaldehyde gas sensing of Pt-decorated MoO3 nanobelts. J. Alloy. Compd. 797, 666–675. doi: 10.1016/j.jallcom.2019.05.145
He, K., He, S. H., Yang, W., and Tian, Q. F. (2019). Ag nanoparticles-decorated α-MoO3 nanorods for remarkable and rapid triethylamine-sensing response boosted by pulse-heating technique. J. Alloy. Compd. 808:151704. doi: 10.1016/j.jallcom.2019.151704
He, S. H., Li, W. D., Feng, L., and Yang, W. (2019). Rational interaction between the aimed gas and oxide surfaces enabling high-performance sensor: the case of acidic α-MoO3 nanorods for selective detection of triethylamine. J. Alloy. Compd. 783, 574–582. doi: 10.1016/j.jallcom.2018.12.349
Ji, H. C., Zeng, W., and Li, Y. Q. (2019a). Assembly of 2D nanosheets into lower-like MoO3: New insight into the petal thickness affect on gas-sensing properties. Mater. Res. Bull. 118:110476. doi: 10.1016/j.materresbull.2019.05.001
Ji, H. C., Zeng, W., and Li, Y. Q. (2019b). New insight into the gas-sensing properties of nanofiber-assembled and nanosheet-assembled hierarchical MoO3 structures. Phys. E. 114:113646. doi: 10.1016/j.physe.2019.113646
Jiang, W. H., Meng, L. L., Zhang, S. F., Chuai, X. H., Zhou, Z. J., Hu, C. H., et al. (2019). Design of highly sensitive and selective xylene gas sensor based on Ni-doped MoO3 nano-pompon. Sens. Actuat. B Chem. 299:126888. doi: 10.1016/j.snb.2019.126888
Jiang, W. H., Wei, D. D., Zhang, S. F., Chuai, X. H., Sun, P., Liu, F. M., et al. (2018). The facile synthesis of MoO3 microsheets and their excellent gas-sensing performance toward triethylamine: high selectivity, excellent stability and superior repeatability. New J. Chem. 42:15111. doi: 10.1039/c8nj03539c
Kwak, D., Wang, M. J., Koski, K. J., Zhang, L., Sokol, H., Maric, R., et al. (2019). Molybdenum trioxide (α-MoO3) nanoribbons for ultrasensitive ammonia (NH3) gas detection: integrated experimental and density functional theory simulation studies. ACS Appl. Mater. Interf. 11, 10697–10706. doi: 10.1021/acsami.8b20502
Li, T. M., Zeng, W., Zhang, Y. Y., and Hussain, S. (2015). Nanobelt-assembled nest-like MoO3 hierarchical structure: hydrothermal synthesis and gas-sensing properties. Mater. Lett. 160, 476–479. doi: 10.1016/j.matlet.2015.08.031
Li, W. D., He, S. H., Feng, L., and Yang, W. (2019). Cr-doped α-MoO3 nanorods for the fast detection of triethylamine using a pulse-heating strategy. Mater. Lett. 250, 143–146. doi: 10.1016/j.matlet.2019.05.006
Li, Y. Q. (2017). Morphology-controllable synthesis of three-dimensional α-MoO3 and their applications in gas sensing. Phys. E. 94, 22–24. doi: 10.1016/j.physe.2017.07.010
Li, Z. Q., Song, P., Yang, Z. X., and Wang, Q. (2018). In situ formation of one-dimensional CoMoO4/MoO3 heterojunction as an effective trimethylamine gas sensor. Ceram. Int. 44, 3364–3370. doi: 10.1016/j.ceramint.2017.11.126
Li, Z. Q., Wang, W. J., Zhao, Z. C., Liu, X. R., and Song, P. (2017a). One-step hydrothermal preparation of Ce-doped MoO3 nanobelts with enhanced gas sensing Properties. RSC Adv. 7:28366. doi: 10.1039/c7ra02893h
Li, Z. Q., Wang, W. J., Zhao, Z. C., Liu, X. R., and Song, P. (2017b). Facile synthesis and enhanced trimethylamine sensing performances of W doped MoO3 nanobelts. Mat. Sci. Semicond. Proc. 66, 33–38. doi: 10.1016/j.mssp.2017.04.002
Liu, C. Y., Xu, H. Y., Chen, Z. R., Ye, Q., Wu, X. W., Wang, J. Q., et al. (2019). Enhanced triethylamine sensing properties by designing an α-Fe2O3/α-MoO3 nanostructure directly grown on ceramic tubes. ACS Appl. Nano Mater. 2, 6715–6725. doi: 10.1021/acsanm.9b01630
Liu, Y. L., Yang, S., Lu, Y., Podval'naya, N. V., and Chen, W.S. . (2015). Hydrothermal synthesis of h-MoO3 microrods and their gas sensing properties to ethanol. Appl. Surf. Sci. 359, 114–119. doi: 10.1016/j.apsusc.2015.10.071
Lu, Z. R., Zhou, Q., Wang, C. S., Wei, Z. J., Xu, L. N., and Gui, Y. G. (2018). Electrospun ZnO–SnO2 composite nanofibersand enhanced sensing properties to SF6 decomposition byproduct H2S. Front. Chem. 6:540. doi: 10.3389/fchem.2018.00540
Mandal, B., Aaryashree Das, M., Htay, M. T., and Mukherjee, S. (2019). Architecture tailoring of MoO3 nanostructures for superior ethanol sensing performance. Mater. Res. Bull. 109, 281–290. doi: 10.1016/j.materresbull.2018.09.041
Mandal, B., Aaryashree Singh, R., and Mukherjee, S. (2018). Highly selective and sensitive methanol sensor using rose-like ZnO microcube and MoO3 micrograss-based composite. IEEE Sens. J. 18, 2659–2666. doi: 10.1109/JSEN.2018.2803682
Mo, Y. F., Tan, Z. P., Sun, L. P., Lu, Y. B., and Liu, X. Z. (2020). Ethanol-sensing properties of α-MoO3 nanobelts synthesized by hydrothermal method. J. Alloy. Compd. 812:152166. doi: 10.1016/j.jallcom.2019.152166
Mousavi-Zadeh, S. H., and Rahmani, M. B. (2018). Synthesis and ethanol sensing characteristics of nanostructured MoO3: Zn thin films. Surf. Rev. Lett. 25:11850046. doi: 10.1142/S0218625X18500464
Pandeeswari, R., and Jeyaprakash, B. G. (2014). Nanostructured α-MoO3 thin film as a highly selective TMA sensor. Biosens. Bioelectron. 53, 182–186. doi: 10.1016/j.bios.2013.09.057
Qin, H. Y., Cao, Y. L., Xie, J., Xu, H., and Jia, D. Z. (2017). Solid-state chemical synthesis and xylene-sensing properties of α-MoO3 arrays assembled by nanoplates. Sens. Actuat. B Chem. 242, 769–776. doi: 10.1016/j.snb.2016.11.081
Qu, F. D., Zhou, X. X., Zhang, B. X., Zhang, S. D., Jiang, C. J., Ruan, S. P., et al. (2019). Fe2O3 nanoparticles-decorated MoO3 nanobelts for enhanced chemiresistive gas sensing. J. Alloy. Compd. 782, 672–678. doi: 10.1016/j.jallcom.2018.12.258
Sau, S., Chakraborty, S., Das, T., and Pal, M. (2019). Ethanol sensing properties of nanocrystalline α-MoO3. Front. Mater. 6:285. doi: 10.3389/fmats.2019.00285
Shen, J. L., Guo, S. J., Chen, C., Sun, L., Wen, S. P., Chen, Y., et al. (2017). Synthesis of Ni-doped-MoO3 nanolamella and their improved gas sensing properties. Sens. Actuat. B Chem. 252, 757–763. doi: 10.1016/j.snb.2017.06.040
Shen, S. K., Zhang, X. F., Cheng, X. L., Xu, Y. M, Gao, S., Zhao, H., et al. (2019). Oxygen-vacancy-enriched porous α-MoO3 nanosheets for trimethylamine sensing. ACS Appl. Nano Mater. 2, 8016–8026. doi: 10.1021/acsanm.9b02072
Sui, L. L., Xu, Y. M., Zhang, X. F., Cheng, X. L., Gao, S., Zhao, H., et al. (2015). Construction of three-dimensional flower-like-MoO3 with hierarchical structure for highly selective triethylamine sensor. Sens. Actuat. B Chem. 208, 406–414. doi: 10.1016/j.snb.2014.10.138
Wang, J. X., Zhou, Q., Lu, Z. R., Wei, Z. J., and Zeng, W. (2019a). Gas sensing performances and mechanism at atomic level of Au-MoS2 microspheres. Appl. Surf. Sci. 490, 124–136. doi: 10.1016/j.apsusc.2019.06.075
Wang, J. X., Zhou, Q., Lu, Z. R., Wei, Z. J., and Zeng, W. (2019b). The novel 2D honeycomb-like NiO nanoplates assembled by nanosheet arrays with excellent gas sensing performance. Mater. Lett. 255:26523. doi: 10.1016/j.matlet.2019.126523
Wang, J. X., Zhou, Q., and Zeng, W. (2019c). Competitive adsorption of SF6 decompositions on Ni-doped ZnO (100) surface: computational and experimental study, Appl. Surf. Sci. 479, 185–197. doi: 10.1016/j.apsusc.2019.01.255
Wang, L. W., Wang, S. P., Fu, H., Wang, Y. H., and Yu, K. F. (2018). Synthesis of Au nanoparticles functionalized 1D α-MoO3 nanobelts and their gas sensing properties. NANO: Brief Rep. Rev.13:1850115. doi: 10.1142/S1793292018501151
Wang, S. Q., Xie, J., Hu, J. D., Qin, H. Y., and Cao, Y. L. (2020). Fe-doped α-MoO3 nanoarrays: Facile solid-state synthesis and excellent xylene-sensing performance. Appl. Surf. Sci. 512:145722. doi: 10.1016/j.apsusc.2020.145722
Wei, Q., Song, P., Li, Z. Q., Yang, Z. X., and Wang, Q. (2019). Enhanced triethylamine sensing performance of MoO3 nanobelts by RuO2 nanoparticles decoration. Vacuum 162, 85–91. doi: 10.1016/j.vacuum.2019.01.028
Wei, Z. J., Zhou, Q., Wang, J. X., Lu, Z. R., Xu, L. N., and Zeng, W. (2019). Hydrothermal synthesis of SnO2 nanoneedle-anchored NiO microsphere and its gas sensing performances. Nanomaterials 9:1015. doi: 10.3390/nano9071015
Wei, Z. J., Zhou, Q., and Zeng, W. (2020). Hierarchical WO3–NiO microflower for high sensitivity detection of SF6 decomposition byproduct H2S. Nanotechnology 31:215701. doi: 10.1088/1361-6528/ab73bd
Xia, Y. C., Wu, C. S., Zhao, N. Y., and Zhang, H. (2016). Spongy MoO3 hierarchical nanostructures for excellent performance ethanol sensor. Mater. Lett. 171, 117–120. doi: 10.1016/j.matlet.2015.12.159
Xiao, S. H., Liu, B., Zhou, R., Liu, Z. W., Li, Q. H., and Wang, T. H. (2018). Room-temperature H2 sensing interfered by CO based on interfacial effects in palladium-tungsten oxide nanoparticles. Sens. Actuat. B Chem. 254, 966–972. doi: 10.1016/j.snb.2017.07.169
Xu, K., Wei, W. Y., Sun, Y., Lu, W. H., Yu, T., Yang, Y., et al. (2019). Design of NiCo2O4 porous nanosheets/α-MoO3 nanorods heterostructures for ppb-level ethanol detection. Powder Technol. 345, 633–642. doi: 10.1016/j.powtec.2019.01.051
Yan, H. H., Song, P., Zhang, S., Zhang, J., Yang, Z. X., and Wang, Q. (2016). Au nanoparticles modified MoO3 nanosheets with their enhanced properties for gas sensing. Sens. Actuat. B Chem. 236, 201–207. doi: 10.1016/j.snb.2016.05.139
Yang, S. A., Liu, Y. L., Chen, T., Jin, W., Yang, T. Q., Cao, M. C., et al. (2017). Zn doped MoO3 nanobelts and the enhanced gas sensing properties to ethanol. Appl. Surf. Sci. 393, 377–384. doi: 10.1016/j.apsusc.2016.10.021
Yang, S. A., Liu, Y. L., Chen, W., Jin, W., Zhou, J., Zhang, H., et al. (2016). High sensitivity and good selectivity of ultralong MoO3 nanobelts for trimethylamine gas. Sens. Actuat. B Chem. 226, 478–485. doi: 10.1016/j.snb.2015.12.005
Yang, S. L., Wang, Z., Hu, Y. M., Cai, Y. X., Huang, R., Li, X. X., et al. (2018). Defect-original room-temperature hydrogen sensing of MoO3 nanoribbon: experimental and theoretical studies. Sens. Actuat. B Chem. 260, 21–32. doi: 10.1016/j.snb.2017.12.166
Zhang, D. Z., Jiang, C. X., and Wu, J. F. (2018). Layer-by-layer assembled In2O3 nanocubes/flower-like MoS2 nanofilm for room temperature formaldehyde sensing. Sens. Actuat. B Chem. 273, 176–184. doi: 10.1016/j.snb.2018.06.044
Zhang, F. D., Dong, X., Cheng, X. L., Xu, Y. M., Zhang, X. F., and Huo, L. H. (2019). Enhanced gas-sensing properties for trimethylamine at low temperature based on MoO3/BI2MO3O12 hollow microspheres. ACS Appl. Mater. Interfaces 11, 11755–11762. doi: 10.1021/acsami.8b22132
Zhang, J., Song, P., Li, J., Yang, Z. X., and Wang, Q. (2017). Template-assisted synthesis of hierarchical MoO3 microboxes and their high gas-sensing performance. Sens. Actuat. B Chem. 249, 458–466. doi: 10.1016/j.snb.2017.04.137
Zhang, J., Song, P., Li, Z. Q., Zhang, S., Yang, Z. X., and Wang, Q. (2016). Enhanced trimethylamine sensing performance of single-crystal MoO3 nanobelts decorated with Au nanoparticles. J. Alloy. Compd. 685, 1024–1033. doi: 10.1016/j.jallcom.2016.06.257
Zhang, Q. Y., Zhou, Q., Lu, Z. R., Wei, Z. J., Xu, L. N., et al. (2018). Recent advances of SnO2-based sensors for detecting fault characteristic gases extracted from power transformer oil. Front. Chem. 6:364. doi: 10.3389/fchem.2018.00364
Zhou, Q., Chen, W. G., Xu, L. N., Kumar, R., Gui, Y. G., Zhao, Z. Y., et al. (2018a). Highly sensitive carbon monoxide (CO) gas sensors based on Ni and Zn doped SnO2 nanomaterials. Ceram. Int. 44, 4392–4399. doi: 10.1016/j.ceramint.2017.12.038
Zhou, Q., Umar, A., Sodki, E., Amine, A., Xu, L. N., and Gui, Y. G. (2018b). Fabrication and characterization of highly sensitive and selective sensors based on porous NiO nanodisks. Sens. Actuat. B Chem. 259, 604–615. doi: 10.1016/j.snb.2017.12.050
Zhou, Q., Xu, L. N., Umar, A., Chen, W. G., and Kumar, R. (2018c). Pt nanoparticles decorated SnO2 nanoneedles for efficient CO gas sensing applications. Sens. Actuat. B Chem. 256, 656–664. doi: 10.1016/j.snb.2017.09.206
Zhou, Q., Zeng, W., Chen, W. G., Xu, L. N., Kumar, R., and Umar, A. (2019). High sensitive and low-concentration sulfur dioxide (SO2) gas sensor application of heterostructure NiO-ZnO nanodisks. Sens. Actuat. B Chem. 298:126870. doi: 10.1016/j.snb.2019.126870
Zhou, Q., Zhang, Q. Y., Liu, H. C., Hong, C. X., Wu, G. L., Peng, S. D., et al. (2017). Research on gas sensing properties of orthorhombic molybdenum oxide based sensor to hydrogen sulfide. J. Nanoelectr. Optoelectr. 12, 1072–1076. doi: 10.1166/jno.2017.2119
Zhu, L., Zeng, W., Li, Y. Q., and Yang, J. D. (2019). Enhanced ethanol gas-sensing property based on hollow MoO3 microcages. Phys. E. 106, 170–175. doi: 10.1016/j.physe.2018.10.038
Keywords: MoO3, gas sensors, volatile organic compounds, functional modification, gas-sensing mechanism
Citation: Wang J, Zhou Q, Peng S, Xu L and Zeng W (2020) Volatile Organic Compounds Gas Sensors Based on Molybdenum Oxides: A Mini Review. Front. Chem. 8:339. doi: 10.3389/fchem.2020.00339
Received: 27 February 2020; Accepted: 01 April 2020;
Published: 05 May 2020.
Edited by:
Liwei Wang, Guangxi University, ChinaReviewed by:
Zhaoxiong Yan, Jianghan University, ChinaZhe Chen, Jilin Institute of Chemical Technology, China
Copyright © 2020 Wang, Zhou, Peng, Xu and Zeng. This is an open-access article distributed under the terms of the Creative Commons Attribution License (CC BY). The use, distribution or reproduction in other forums is permitted, provided the original author(s) and the copyright owner(s) are credited and that the original publication in this journal is cited, in accordance with accepted academic practice. No use, distribution or reproduction is permitted which does not comply with these terms.
*Correspondence: Qu Zhou, zhouqu@swu.edu.cn; Wen Zeng, wenzeng@cqu.edu.cn