Theoretical Insight Into the Ultralong Room-Temperature Phosphorescence of Nonplanar Aromatic Hydrocarbon
- 1Key Laboratory of Flexible Electronics (KLOFE) & Institute of Advanced Materials (IAM), Nanjing Tech University (Nanjing Tech), Nanjing, China
- 2School of Environment, South China Normal University, Guangzhou, China
- 3Hefei National Laboratory for Physical Science at the Microscale, University of Science and Technology of China, Hefei, China
Purely aromatic hydrocarbon materials with ultralong room-temperature phosphorescence (RTP) were reported recently, but which is universally recognized as unobservable. To reveal the inherent luminescent mechanism, two compounds, i.e., PT with a faint RTP and HD with strong RTP featured by nonplanar geometry, were chosen as a prototype to study their excited-state electronic structures by using quantum mechanics/molecular mechanics (QM/MM) model. It is demonstrated that the nonplanar ethylene brides can offer σ-electron to strengthen spin-orbit coupling (SOC) between singlet and triplet excited states, which can not only promote intersystem crossing (ISC) of S1→Tn to increase the population of triplet excitons, but also accelerate the radiative decay rate of T1→S0, and thus improving RTP. Impressively, the nonradiative decay rate only has a small increase, owing to the synergistic effect between the increase of SOC and the reduction of reorganization energy of T1→S0 caused by the restricted torsional motions of aromatic rings. Therefore, a bright and long-lived RTP was obtained in aromatic hydrocarbon materials with twisted structure. This work provided a new insight into the ultralong RTP in pure organic materials.
Introduction
Ultralong room-temperature phosphorescence (RTP) in purely organic materials has been gaining more attention in encryption (An et al., 2015; Ma et al., 2021), display (Wang et al., 2019; Tan et al., 2021), bioimaging (Wang et al., 2020; Wang et al., 2021a) and so on (Yu et al., 2017; He et al., 2019; Zhao et al., 2020). Phosphorescence generally refers to the spin-forbidden radiative transition from triplet to singlet states. RTP is common in coordination complexes, which have a lifetime of μs-scale, owing to the increased radiative transition caused by transition metal (eg, Ir, Pt, etc.). (Yam et al., 2015). In contrast, pure organic compounds, in principle, have an ultralong phosphorescence lifetime of second-scale, however, their RTP phenomenon is almost unobservable due to the weak spin-orbit coupling (SOC) effect. (TurroRamamurthy and Scaiano, 2010). Namely, the ultralong RTP in aromatic hydrocarbon materials is extremely rare, (Clapp, 1939; Bilen et al., 1978), because of the forbidden intersystem crossing (ISC) process between singlet and triplet excited states. To overcome this issue, the heavy atoms (eg., Br and I) (Cai et al., 2018; Wang et al., 2021b) and carbonyl groups (Zhao et al., 2016; Jia et al., 2020) were incorporated into organic molecules to promote ISC process for achieving ultralong RTP, in combination with the suppression of the nonradiative quenching through rigid environment, (Wu et al., 2020; Zheng et al., 2020; Zhou et al., 2020; Chen et al., 2021; Xie et al., 2021), such as crystal engineering and host-guest system. Very recently, Bechtold et al. reported that the nonplanar aromatic hydrocarbon, named as 5,6,11,12,17,18-hexahydrobenzo [2,1-p]chrysene (HD), (Salla et al., 2019), can show an ultralong RTP, which was attributed to the pronounced SOC induced by non-planar configuration. However, the enhanced SOC also reduces the RTP lifetime. Therefore, it is urgent to probe the origin of the ultralong RTP in the nonplanar aromatic hydrocarbons.
The 5′-phenyl-1,1’:3′,1″-terphenyl (PT) has a faint RTP in crystal, while HD shows an efficient RTP with a lifetime of 380 m by introducing saturated ethylene bridges between the central benzene and outer benzene rings of PT. (Li et al., 2015; Salla et al., 2019). Therefore, PT and HD molecules are good prototype to expound the dependence of ultralong RTP on the nonplanar conformation. We thus take these two molecules as example to quantitatively evaluate the nature of the molecular excited states by combing quantum and molecular mechanics (QM/MM) approach and time-dependent density functional theory (TDDFT) coupled with the thermal vibration correlation function (TVCF) formalism and unravel the origin of the ultralong RTP in nonplanar aromatic hydrocarbons, especially the relationship between RTP and the nonplanar conformation.
Materials and Methods
The QM/MM models were built based on the crystal structures from X-ray single-crystal diffraction as shown in Figure 1B. The central one molecule was chosen as active QM part, while the remaining molecules were defined as rigid MM part, which was performed by using ChemShell 3.7 (Sherwood et al., 2003) packages interfacing ORCA (Neese, 2018) for QM and DL_POLY (Smith and Forester, 1996) with the GAFF (Wang et al., 2004) for the MM part. The geometry optimization and harmonic vibrational frequency of the ground state (S0) and the lowest triplet (T1) excited states were calculated at (TD) B3LYP/def2-SVP level. Based on the T1-geometry, TD-B3LYP/def2-SVP method was used to calculate their electronic structure nature, including excitation energies and natural transition orbitals (NTOs) of the low-lying excited states. All the above calculations were implemented by Gaussian 09 software. (Frisch et al., 2009). At the same level, the SOC matrix elements (ξ) were evaluated by using Beijing Density Function package (BDF) (Liu et al., 1997; Liu et al., 2003; Hirao and Ishikawa, 2004; Li et al., 2013).
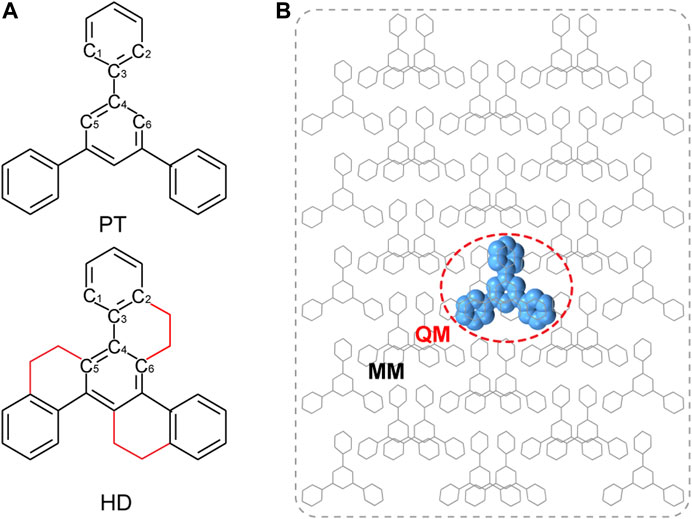
FIGURE 1. (A) Chemical structure of PT and HD; (B) Setup of QM/MM models by taking PT as an example.
The phosphorescence lifetime τp = 1/(kp + knr) is determined by radiative decay rate kp and nonradiative decay rate knr. The former can be estimated by Einstein spontaneous emission relationship
Results and Discussions
The light-emitting behavior of organic phosphors is governed by the molecular excited-state process. We thus explored the excited-state electronic structure, including excitation energy, NTOs and SOC matrix elements, and excited state decay rates of T1→S0 to account for the origin the ultralong RTP in nonplanar aromatic hydrocarbons.
Nature of The Low-Lying Excited States
As seen from Figure 2, the energy gap of S1→T6 is decreased from 0.08 eV in PT to 0.01eV in HD, along with the similar SOC values ξ(S1, T6). While the ξ(S1, Tn) (n = 1–3) are about twice in HD than in PT molecules, which can be ascribed to the introduction of σ→π* transition (>4.0%) caused by the twisted ethylene bridge in HD (Schmidt et al., 2007), see Supplementary Figure S1 and Supplementary Table S3. Thus, it rationally speculated that the ISC process of S1→Tn should be largely promoted, which is responsible for the bright RTP in HD. On the other hand, the ξ(T1, S0) shows an increasing tendency from 0.19 cm−1 in PT to 0.37 cm−1 in HD, which can be attributed to the increased proportion of σ→π* transition for T1 state from 0.0% in PT to 5.47% in HD caused by the twisted ethylene bridge (see Figure 2 and Supplementary Tables S1, S2), and such change is beneficial to the increase of SOC according to El-Sayed’s rule (El‐Sayed, 1963; El-Sayed, 1968). Such enlarged SOC of T1→S0 in HD not only increases the radiative decay rate kp, but also largely accelerates the nonradiative decay rate knr, making the shorten of the RTP lifetime (Ma et al., 2019). In addition, the excitation energy of T1 state is decreased from 2.31 eV in PT to 2.23 eV in HD, where the excitation energy of HD agrees well with the RTP spectra (2.33 eV) in experiment, indicating the promotion of the knr. Namely, both the increase of the SOC value and decrease of the energy gap of T1→S0 are favorable for the acceleration of the nonradiative decay process, and this change is against the ultralong phosphorescence lifetime. Therefore, it is necessary to further expound the effect of vibronic coupling on the ultralong RTP of nonplanar HD compound.
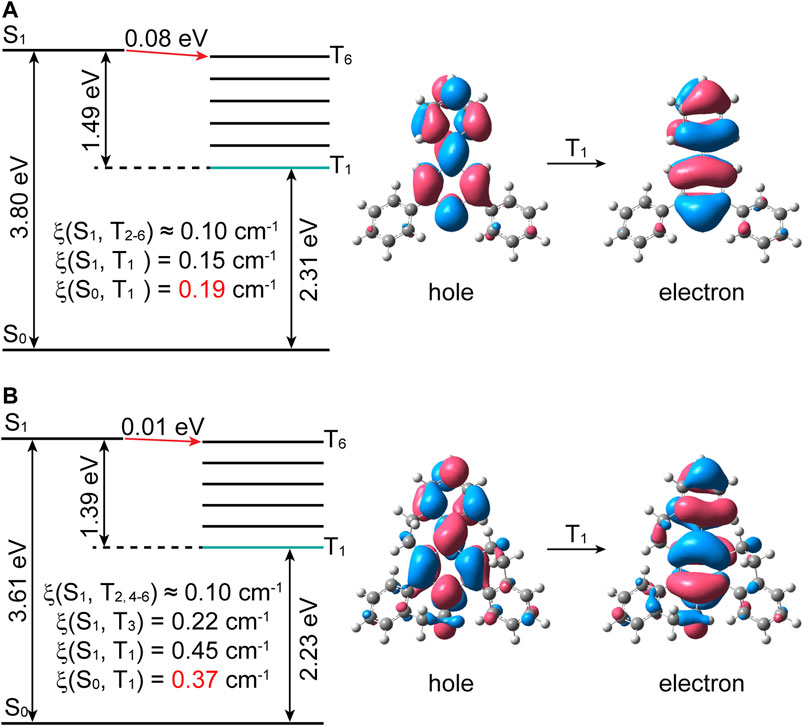
FIGURE 2. Calculated energy diagram and SOC matrix elements (ξ) of the low-lying excited states, and NTOs of T1 states for (A) PT and (B) HD.
Excited State Decay Rates of T1→S0
It is well-known that the phosphorescence lifetime is given by the formula τp = 1/(kp + knr), where the kp can be evaluated by Einstein spontaneous emission relationship, and the knr was calculated by TVCF rate theory using MOMAP program (Niu et al., 2018). Table 1 and Supplementary Figure S2 show that, going from PT to HD, the knr is slightly increased from 4.32 to 9.49 s−1, and it dominates the phosphorescence lifetime, because of the slower kp with a small enlargement from 3.00 × 10–2 to 6.40 × 10–2 s−1. Consequently, the calculated phosphorescence lifetime is reduced from 0.23 s in PT to 0.11 s in HD. Impressively, the RTP lifetime of HD in experiment (0.38 s) are reproduced by the calculated value of 0.11 m. These results demonstrated that the nonplanar ethylene bridges have a little impact on the ultralong RTP lifetime of HD.
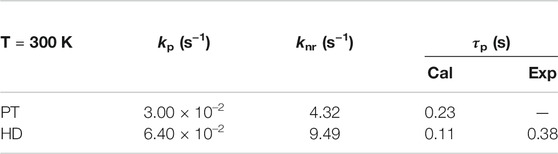
TABLE 1. Calculated radiative kp and nonradiative decay rates knr of T1→S0, as well as the RTP lifetime τp = 1/(kp + knr) for PT and HD. The experimental value was also given as a comparison.
To better understand such a small change, we then focus on the nonradiative decay rate knr, which was not only connected with SOC and energy gap E of T1→S0, but also was governed by the electron-vibration coupling characterized by the reorganization energy λ. (Marian, 2012). As shown in Figure 3, the total reorganization energy has a large decrease, from 3,624.29 cm−1 in PT to 3,096.66 cm−1 in HD. Such a change is primarily originated from the reduced λ in low-frequency regions (ω < 300 cm−1) from 827.37 cm−1 in PT to 307.30 cm−1 in HD, which are mainly associated with the torsional motions of benzene rings. Additionally, the λ in high-frequency regions (∼1,600 cm−1) relating to the C=C stretching vibration also provide a significant contribution, decreasing from 1,388.87 cm−1 in PT to 1,076.33 cm−1 in HD (see Supplementary Figure S3). These results demonstrated that the nonplanar ethylene bridges in HD can reduce the λ, resulting in the decrease of knr. It is worthwhile note that this change is conflict to the acceleration of the knr due to the increased SOC and reduced energy gap of T1→S0 caused by the nonplanar ethylene bridges (see Figure 2). Therefore, the knr has only a slight increase from PT to HD, owing to the balance of the change in SOC, E and λ caused by the nonplanar ethylene bridges.
Next, by projecting the total λ into the internal coordinate relaxation of the compounds, Figure 4A showed that, going from PT to HD, the contributions from the bond length are increased from 74.84 to 78.34%, while the contributions derived from the bond angle have a tiny variation with a value of ca. 2.0%. Impressively, the contributions came from the dihedral angle associated with the torsional motions are reduced from 23.12% in PT to 19.12% in HD. Figure 4B further showed that such a decease is stemmed from the torsional vibrations between the central benzene and one nearby outer benzene (see Supplementary Table S4), for example, the reorganization energy from the dihedral angle of C1-C3-C4-C5 is reduced from 169.06 cm−1 to 98.61 cm−1, and for C2-C3-C4-C6, it is decreased from 164.03 cm−1 to 25.87 cm−1. Based on these features, we concluded that the nonplanar ethylene bridges can suppress the electron-vibration coupling of the torsional vibration modes, largely hindering the enlargement of nonradiative decay rate.
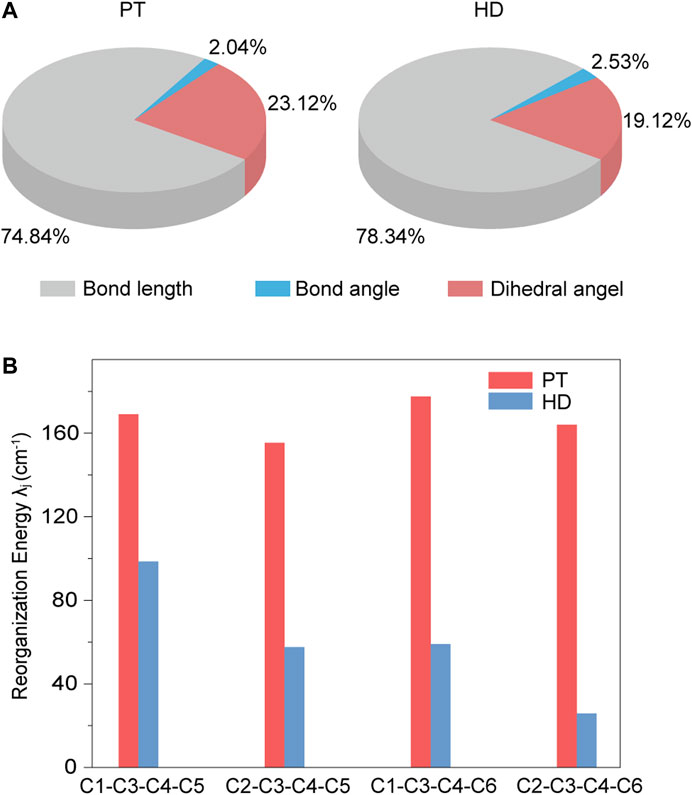
FIGURE 4. (A) Contributions to the total reorganization energy from the bond length, bond angle, and dihedral angle for PT and HD; (B) The mainly contributions to the reorganization energy from the different dihedral angles for PT and HD.
Phosphorescence Spectra Under Ambient Condition
Beyond the RTP lifetime, we further calculated the phosphorescence spectra to verify our proposed ultralong RTP mechanism in nonplanar aromatic hydrocarbon. Figure 5 then displayed the simulated phosphorescence spectrum of HD to compare with the experiment. It is found that the theoretical results agree well with the experimental spectra; the peak maximum at around 570 nm corresponds to the 0–0 transition; and the shoulder peak at around 610 nm is mainly ascribed to the 0–1 transition for the C=C stretching with 1,602 and 1,604 cm−1. Namely, these results demonstrated our theoretical model is reliable, and it is enough to support the above proposed RTP mechanism.
Conclusion
In summary, we explored the nature of low-lying excited states, excited-state decay rates and vibrationally resolved phosphorescence spectra by QM/MM models coupled with the TVCF rate formalism in the MOMAP program, to unravel the origin of the ultralong RTP in nonplanar aromatic hydrocarbon. Theoretical results demonstrated that, from PT to HD, the introduction of the ethylene bridges offer σ-electron to strengthen spin-orbit coupling (SOC) between singlet and triplet excited states, such a change not only largely accelerates the ISC process for efficient RTP, but also increases the radiative and nonradiative decay rates of T1→S0 process, hindering the ultralong phosphorescence lifetime of twisted HD molecule. Beyond the SOC effect, the ethylene bridges also reduce the electron-vibration coupling associated with the torsional vibration modes in low frequency regions (<300 cm−1), strongly reducing the nonradiative decay rate of T1→S0, and thereby facilitating to the ultralong RTP. Therefore, the synergistic effect of SOC and vibronic coupling caused by the ethylene bridges make a slight enlargement of the nonradiative decay rate for nonplanar HD, thereby generating an efficient RTP in twisted hydrocarbon with ultralong lifetime.
Data Availability Statement
The original contributions presented in the study are included in the article/Supplementary Material, further inquiries can be directed to the corresponding author.
Author Contributions
KQ and HM: theoretical calculations and writing manuscript. WG, JG and ZA: data analysis and review. DH: providing the interface between ORCA and ChemShell program.
Funding
This work was supported by the Natural Science Foundation of the Jiangsu Higher Education Institutions (Grant No. 19KJB150010), the National Natural Science Foundation of China (21973043) and the Hefei National Laboratory for Physical Sciences at the Microscale (Grant No. KF2020103).
Conflict of Interest
The authors declare that the research was conducted in the absence of any commercial or financial relationships that could be construed as a potential conflict of interest.
The reviewer BX declared a shared affiliation with one of the authors, DH, to the handling editor at time of review.
Publisher’s Note
All claims expressed in this article are solely those of the authors and do not necessarily represent those of their affiliated organizations, or those of the publisher, the editors and the reviewers. Any product that may be evaluated in this article, or claim that may be made by its manufacturer, is not guaranteed or endorsed by the publisher.
Acknowledgments
We are grateful to the High-Performance Computing Center in Nanjing Tech University for supporting the computational resources.
Supplementary Material
The Supplementary Material for this article can be found online at: https://www.frontiersin.org/articles/10.3389/fchem.2021.740018/full#supplementary-material
References
An, Z., Zheng, C., Tao, Y., Chen, R., Shi, H., Chen, T., et al. (2015). Stabilizing Triplet Excited States for Ultralong Organic Phosphorescence. Nat. Mater 14, 685–690. doi:10.1038/nmat4259
Bilen, C. S., Harrison, N., and Morantz, D. J. (1978). Unusual Room Temperature Afterglow in Some Crystalline Organic Compounds. Nature 271, 235–237. doi:10.1038/271235a0
Cai, S., Shi, H., Tian, D., Ma, H., Cheng, Z., Wu, Q., et al. (2018). Enhancing Ultralong Organic Phosphorescence by Effective π-Type Halogen Bonding. Adv. Funct. Mater. 28, 1705045. doi:10.1002/adfm.201705045
Chen, B., Huang, W., Nie, X., Liao, F., Miao, H., Zhang, X., et al. (2021). An Organic Host-Guest System Producing Room‐Temperature Phosphorescence at the Parts‐Per‐Billion Level. Angew. Chem. Int. Ed. 60, 16970–16973. doi:10.1002/anie.202106204
Clapp, D. B. (1939). The Phosphorescence of Tetraphenylmethane and Certain Related Substances. J. Am. Chem. Soc. 61, 523–524. doi:10.1021/ja01871a504
El‐Sayed, M. A. (1963). Spin-Orbit Coupling and the Radiationless Processes in Nitrogen Heterocyclics. J. Chem. Phys. 38, 2834–2838. doi:10.1063/1.1733610
El-Sayed, M. A. (1968). Triplet State. Its Radiative and Nonradiative Properties. Acc. Chem. Res. 1, 8–16. doi:10.1021/ar50001a002
Frisch, M. J., Trucks, G. W., Schlegel, H. B., Scuseria, G. E., Robb, M. A., et al. (2009). Gaussian 09 Revison C.01. Wallingford: Gaussian, Inc.
He, Z., Gao, H., Zhang, S., Zheng, S., Wang, Y., Zhao, Z., et al. (2019). Achieving Persistent, Efficient, and Robust Room‐Temperature Phosphorescence from Pure Organics for Versatile Applications. Adv. Mater. 31, 1807222. doi:10.1002/adma.201807222
Hirao, K., and Ishikawa, Y. (2004). Recent Advances in Relativistic Molecular Theory. Recent Advances in Computational Chemistry. Singapore: World Scientific, 257–282. doi:10.1142/5433
Jia, W., Wang, Q., Shi, H., An, Z., and Huang, W. (2020). Manipulating the Ultralong Organic Phosphorescence of Small Molecular Crystals. Chem. Eur. J. 26, 4437–4448. doi:10.1002/chem.201904500
Li, L., Chen, M., Zhang, H., Nie, H., Sun, J. Z., Qin, A., et al. (2015). Influence of the Number and Substitution Position of Phenyl Groups on the Aggregation-Enhanced Emission of Benzene-Cored Luminogens. Chem. Commun. 51, 4830–4833. doi:10.1039/c5cc00241a
Li, Z., Suo, B., Zhang, Y., Xiao, Y., and Liu, W. (2013). Combining Spin-Adapted Open-Shell TD-DFT with Spin-Orbit Coupling. Mol. Phys. 111, 3741–3755. doi:10.1080/00268976.2013.785611
Liu, W., Hong, G., Dai, D., Li, L., and Dolg, M. (1997). The Beijing Four-Component Density Functional Program Package (BDF) and its Application to EuO, EuS, YbO and YbS. Theor. Chem. Acc. Theor. Comput. Model. (Theoretica Chim. Acta) 96, 75–83. doi:10.1007/s002140050207
Liu, W., Wang, F., and Li, L. (2003). The Beijing Density Functional (BDF) Program Package: Methodologies and Applications. J. Theor. Comput. Chem. 02, 257–272. doi:10.1142/S0219633603000471
Ma, H., Peng, Q., An, Z., Huang, W., and Shuai, Z. (2019). Efficient and Long-Lived Room-Temperature Organic Phosphorescence: Theoretical Descriptors for Molecular Designs. J. Am. Chem. Soc. 141, 1010–1015. doi:10.1021/jacs.8b11224
Ma, L., Sun, S., Ding, B., Ma, X., and Tian, H. (2021). Highly Efficient Room‐Temperature Phosphorescence Based on Single‐Benzene Structure Molecules and Photoactivated Luminescence with Afterglow. Adv. Funct. Mater. 31, 2010659. doi:10.1002/adfm.202010659
Marian, C. M., and Christel, M. (2012). Spin-Orbit Coupling and Intersystem Crossing in Molecules. Wires Comput. Mol. Sci. 2, 187–203. doi:10.1002/wcms.83
Neese, F. (2018). Software Update: the ORCA Program System, Version 4.0. Wires Comput. Mol. Scimol. Sci. 8, e13271. doi:10.1002/wcms.1327
Niu, Y., Li, W., Peng, Q., Geng, H., Yi, Y., Wang, L., et al. (2018). MOlecular MAterials Property Prediction Package (MOMAP) 1.0: A Software Package for Predicting the Luminescent Properties and Mobility of Organic Functional Materials. Mol. Phys. 116, 1078–1090. doi:10.1080/00268976.2017.1402966
Salla, C. A. M., Farias, G., Rouzières, M., Dechambenoit, P., Durola, F., Bock, H., et al. (2019). Persistent Solid‐State Phosphorescence and Delayed Fluorescence at Room Temperature by a Twisted Hydrocarbon. Angew. Chem. Int. Ed. 58, 6982–6986. doi:10.1002/anie.201901672
Schmidt, K., Brovelli, S., Coropceanu, V., Beljonne, D., Cornil, J., Bazzini, C., et al. (2007). Intersystem Crossing Processes in Nonplanar Aromatic Heterocyclic Molecules. J. Phys. Chem. A. 111, 10490–10499. doi:10.1021/jp075248q
Sherwood, P., de Vries, A. H., Guest, M. F., Schreckenbach, G., Catlow, C. R. A., French, S. A., et al. (2003). QUASI: A General Purpose Implementation of the QM/MM Approach and its Application to Problems in Catalysis. J. Mol. Struct. THEOCHEM 632, 1–28. doi:10.1016/S0166-1280(03)00285-9
Smith, W., and Forester, T. R. (1996). DL_POLY_2.0: A General-Purpose Parallel Molecular Dynamics Simulation Package. J. Mol. Graphics 14, 136–141. doi:10.1016/S0263-7855(96)00043-4
Tan, S., Jinnai, K., Kabe, R., and Adachi, C. (2021). Long‐Persistent Luminescence from an Exciplex‐Based Organic Light‐Emitting Diode. Adv. Mater. 33, 2008844. doi:10.1002/adma.202008844
TurroRamamurthy, N. J. V., and Scaiano, J. C. (2010). Modern Molecular Photochemistry of Organic Molecules. Sausalito: University Science Books.
Wang, J., Wolf, R. M., Caldwell, J. W., Kollman, P. A., and Case, D. A. (2004). Development and Testing of a General Amber Force Field. J. Comput. Chem. 25, 1157–1174. doi:10.1002/jcc.20035
Wang, T., Su, X., Zhang, X., Nie, X., Huang, L., Zhang, X., et al. (2019). Aggregation‐Induced Dual‐Phosphorescence from Organic Molecules for Nondoped Light‐Emitting Diodes. Adv. Mater. 31, 1904273. doi:10.1002/adma.201904273
Wang, X. F., Guo, W. J., Xiao, H., Yang, Q. Z., Chen, B., Chen, Y. Z., et al. (2020). Pure Organic Room Temperature Phosphorescence from Unique Micelle‐Assisted Assembly of Nanocrystals in Water. Adv. Funct. Mater. 30, 1907282. doi:10.1002/adfm.201907282
Wang, X., Shi, H., Ma, H., Ye, W., Song, L., Zan, J., et al. (2021). Organic Phosphors with Bright Triplet Excitons for Efficient X-Ray-Excited Luminescence. Nat. Photon. 15, 187–192. doi:10.1038/s41566-020-00744-0
Wang, Y., Gao, H., Yang, J., Fang, M., Ding, D., Tang, B. Z., et al. (2021). High Performance of Simple Organic Phosphorescence Host-Guest Materials and Their Application in Time‐Resolved Bioimaging. Adv. Mater. 33, 2007811. doi:10.1002/adma.202007811
Wu, X., Huang, C.-Y., Chen, D.-G., Liu, D., Wu, C., Chou, K.-J., et al. (2020). Exploiting Racemism Enhanced Organic Room-Temperature Phosphorescence to Demonstrate Wallach's Rule in the Lighting Chiral Chromophores. Nat. Commun. 11, 2145. doi:10.1038/s41467-020-15976-5
Xie, Z., Zhang, X., Wang, H., Huang, C., Sun, H., Dong, M., et al. (2021). Wide-range Lifetime-Tunable and Responsive Ultralong Organic Phosphorescent Multi-Host/guest System. Nat. Commun. 12, 3522. doi:10.1038/s41467-021-23742-4
Yam, V. W.-W., Au, V. K.-M., and Leung, S. Y.-L. (2015). Light-Emitting Self-Assembled Materials Based on D8 and D10 Transition Metal Complexes. Chem. Rev. 115, 7589–7728. doi:10.1021/acs.chemrev.5b00074
Yu, Z., Wu, Y., Xiao, L., Chen, J., Liao, Q., Yao, J., et al. (2017). Organic Phosphorescence Nanowire Lasers. J. Am. Chem. Soc. 139, 6376–6381. doi:10.1021/jacs.7b01574
Zhao, W., He, Z., Lam, J. W. Y., Peng, Q., Ma, H., Shuai, Z., et al. (2016). Rational Molecular Design for Achieving Persistent and Efficient Pure Organic Room-Temperature Phosphorescence. Chem 1, 592–602. doi:10.1016/j.chempr.2016.08.010
Zhao, W., He, Z., and Tang, B. Z. (2020). Room-temperature Phosphorescence from Organic Aggregates. Nat. Rev. Mater. 5, 869–885. doi:10.1038/s41578-020-0223-z
Zheng, S., Zhu, T., Wang, Y., Yang, T., and Yuan, W. Z. (2020). Accessing Tunable Afterglows from Highly Twisted Nonaromatic Organic AIEgens via Effective Through‐Space Conjugation. Angew. Chem. Int. Ed. 59, 10018–10022. doi:10.1002/anie.202000655
Keywords: ultralong phosphorescence, organic phosphorescence, nonplanar aromatic hydrocarbon, spin-orbit coupling, vibronic coupling
Citation: Qin K, Gong W, Gao J, Hu D, Shi H, Yao W, An Z and Ma H (2021) Theoretical Insight Into the Ultralong Room-Temperature Phosphorescence of Nonplanar Aromatic Hydrocarbon. Front. Chem. 9:740018. doi: 10.3389/fchem.2021.740018
Received: 12 July 2021; Accepted: 25 August 2021;
Published: 06 September 2021.
Edited by:
Tao Yu, Northwestern Polytechnical University, ChinaReviewed by:
Bingjiaxu Xu, South China Normal University, ChinaLei Ji, Northwestern Polytechnical University, China
Yuezhou Zhang, Northwestern Polytechnical University, China
Copyright © 2021 Qin, Gong, Gao, Hu, Shi, Yao, An and Ma. This is an open-access article distributed under the terms of the Creative Commons Attribution License (CC BY). The use, distribution or reproduction in other forums is permitted, provided the original author(s) and the copyright owner(s) are credited and that the original publication in this journal is cited, in accordance with accepted academic practice. No use, distribution or reproduction is permitted which does not comply with these terms.
*Correspondence: Huili Ma, iamhlma@njtech.edu.cn