A Review on Synthesis and Optoelectronic Applications of Nanostructured ZnO
- School of Chemical and Materials Engineering, National University of Sciences and Technology (NUST), Islamabad, Pakistan
Nanostructured ZnO has gained a lot of interest as a suitable material for various applications, especially sensing, energy conversion, and storage. ZnO nanostructures can be synthesized in several ways. It is one of the materials that can be prepared in a variety of morphologies including hierarchical nanostructures. This review article presents a review of current research activities on the growth of ZnO Nanorods. The article covers various water-based routes of synthesis and is further characterized by the type of substrate used for the growth. The growth factors involved in the hydrothermal and chemical bath deposition methods are discussed. These factors include the variety of precursors, time, temperature, and the seeding method employed. At the end, applications such as gas sensing and improvement in Opto-electric properties are discussed.
Introduction
Zinc Oxide (ZnO) is a wide-band-gap (3.37 eV) semiconductor of the II-VI semiconductor group. The native doping of the semiconductor due to oxygen vacancies or zinc interstitials is n-type (Ozgur et al., 2005). The application of these nanorods depends on factors like crystallinity and surface morphology, orientation, optical, and electrical properties. The synthesis of one-dimensional (1D) nanostructures of ZnO, has attracted much attention because ZnO is a potential candidate for Gas Sensing (Cittadini et al., 2015; Zhang et al., 2020), UV sensing (Fang et al., 2009; Zhou et al., 2020), and Immuno-sensors for Viruses (Sanguino et al., 2014; Han et al., 2016), in LEDs (Lupan et al., 2010; Mohammad et al., 2020), in solar cell applications such as an electron transporting layer in Perovskite (Huang et al., 2017; Yun et al., 2020), and Dye Sensitized Solar cells (Thambidurai et al., 2012; Jung et al., 2020) etc.
Major Advantages for 1D Zinc Oxide Nanostructures Include
(i) Their growth can be carried out on a wide variety of substrates (including polymers which can be amorphous but offer the advantage of being flexible or/and biodegradable).
(ii) There is a wide variety of synthesis routes for the growth of these nanostructures, each offering a certain niche advantage(Ray et al., 2020).
(iii) The common defects or stress elevators in these nanorods are oxygen vacancies (Ozgur et al., 2005). The nanorods can relieve the stresses by elastic relaxation (Huang et al., 2001).
(iv) Unique properties such as high bulk electron mobility or direct band equivalent to the energy of UV (ultraviolet) light. Other favorable properties include a wide band gap, good transparency, room-temperature luminescence, and high electron mobility (Roza et al., 2020).
(v) Quantum confinement can be exploited by the use of heterostructures of ultrathin layers in a single nanorod exhibiting atomically abrupt interfaces (Park et al., 2003).
(vi) ZnO has a tunable optical band gap, which can be altered by changing the composition, morphology, size etc.
(vii) Another distinct advantage is that ZnO is considered a bio-safe and biocompatible compound, as a trace amount of Zinc is present in the human body (Jayaprakash et al., 2020).
Based on the above facts, ZnO is a very important material. Its 1D nanostructures have been proven to give improved performances in a wide variety of applications. This review article focuses on the growth methods of 1D ZnO nanorod arrays in terms of seeding layer, alignment, and the aspect ratio of the grown 1D nanorod arrays.
Various Routes for Growth of Zinc Oxide Nanorod Structures
There are many different routes for the manufacturing of 1D nanostructures of ZnO. The present review deals with the solution-based methods only. The solution-based synthesis routes for ZnO nanorod arrays under discussion are:
(i) Hydrothermal Growth
(ii) Chemical Bath Deposition
(iii) Electrochemical Deposition
(iv) Pulsed LASER Deposition
(v) Spray Pyrolysis
As the present study deals with the orientation and the aspect ratio of the ZnO nanorods, the nature of the substrate plays an important role in the growth process. Most substrates have a large difference in lattice parameters with ZnO, so a seed layer is deposited on the substrate before the growth of nanorods is carried out. The seed layer required for the growth can be deposited in the form of ZnO thin film of various thicknesses by sputtering, spin, or dip coating and/or drop casting, Atomic layer deposition etc. The following section provides a detailed description of various solution-based methods to grow aligned nanorod arrays of ZnO via solution-based methods.
Hydrothermal Method
Hydrothermal synthesis is a high-pressure aqueous solution-based growth process performed in a sealed steel vessel (autoclave). The process involves crystallizing substances at a high temperature and pressure.
The hydrothermal process offers the advantages of providing uniform growth (Qiaoping et al., 2020; Sutradhar et al., 2020). The growth temperature plays an important role in the overall crystallinity and aspect ratio of the grown nanorods. The commonly used growth temperatures range between 80 and 100°C, while the synthesis duration usually ranges from 1 to 4 h (Abdelouhab et al., 2020; Ghosh et al., 2020). The growth substrates commonly include silicon wafer or coated glass slides. Table 1 enlists the reported work about hydrothermal synthesis of ZnO nanorods. Hassanpour et al. (Hassanpour et al., 2017) compare the growth on both glass and Si (100) wafer substrate; the seed layer was drop casted on both the substrates, then masked by PMMA which was patterned by Electron Beam Lithography followed by annealing for 10 min at 90°C. The growth occurs in 50 mM solutions of zinc nitrate hexahydrate and 50 mM hexamethylenetetramine (HMTA) in deionized water at 85°C for 2 h. They evaluate the effect of the seed layer on the growth and report that annealing at 300°C provides the best seed layer for growth, as shown in Figures 1A–C.
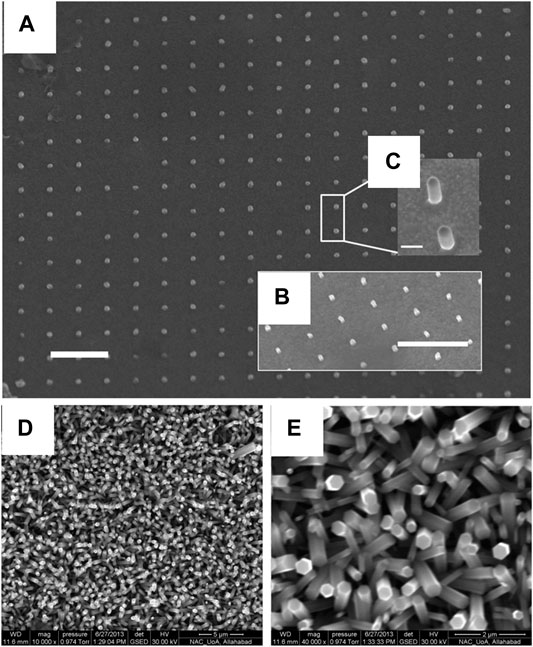
FIGURE 1. SEM images of patterned nanorods on silicon substrates. Panel (A) is a view from the top, while insets (B) and (C) are 60°- tilted views. The scale bars indicate 1 μm for (A) and (B), and 200 nm for inset (C) (Hassanpour et al., 2017)., SEM image of ZnO nanorods with (D) lower magnification (10000 x) and (E) higher magnification (40000 x). (Srivastava et al., 2015).
Growth of Zinc Oxide Rods on Si Wafer Substrate
The growth of ZnO nanorods on a silicon wafer requires a seamless seed layer to overcome the lattice difference between the ZnO and the silicon wafer. An unbroken seed layer is required to grow vertically aligned nanorods with fewer defects (Tang et al., 2020). The deposition techniques like sputtering and atomic layer deposition offer an unbroken layer. Sputtering also has the advantage of in situ monitoring and allows for control of the thickness of the thin film of ZnO being deposited as the seed layer.
Growth of Seed Layer via Spin Coating
In spin coating, the substrates were coated, by spinning the seed solution on the sample (Al-Asedy and Al-Khafaji, 2020). The viscosity and overall lamination of the layer are dependent upon the solution used, and the temperature at which the seed layer was annealed. Srivastava et al. (Srivastava et al., 2015) reported spin coating for making a seed layer by spinning solution (0.5 M of zinc acetate in 20 ml of isopropanol with dropwise addition of 0.5 M of ethanolamine followed by room temperature aging and then stirring it at 50°C for 1 h) on a 1 × 1 cm Si wafer at 3,000 rpm for 30 s. The spinning process was repeated three times followed by an annealing step (80°C for 30 min) after every deposition and was finally annealed at 1100°C for 1 h. The growth of nanorods occurs when the substrate was placed vertically in 0.05 M Zinc Nitrate and 0.05 M Hexamethylene Tetramine (HMTA) at 80°C for 4 h, as shown in Figures 1D,E.
Growth of Seed Layer via Sputtering
In sputtering, the target purity and the chamber pressure, i.e. the pressure of the argon gas, plays an important role in the deposition of the seed layer (Sannakashappanavar et al., 2020). The role of chamber pressure is that it effects the sputtering efficiency, while for deposition of ZnO, if the target used is of metallic zinc, the oxygen environment will ensure the deposition of ZnO, moreover, it counteracts the most common defect of oxygen vacancies in the growth of ZnO. Mbuyisa et al. (Mbuyisa et al., 2015) reported the use of metallic zinc target (Purity of 99.995% and Diameter 50.8 mm) and an oxygen environment in the direct current magnetron sputtering system for the preparation of ZnO film on Si (100) substrate at room temperature. The substrate was cleaned by ultra-sonication and pre-sputtered for 2 min to clean it further in the argon atmosphere. The deposition occurs at a sputtering power of 60 W for 15 min with three different chamber pressures (0.4, 1.2, and 8 Pa) followed by an in-situ annealing process (400°C for 2 h at 0.4 Pa oxygen pressure). For nanorod growth, they followed J. X. Wang et al. (Wang et al., 2006) (Figures 2A–C) and growth took place in 0.05 M zinc chloride and ammonia at pH 11 at 90°C for 60 min followed by air drying of the samples. The characterization of the samples reveals that with an increasing chamber pressure (0.4–12 Pa) the nanorods become highly oriented and their aspect ratio decreases.
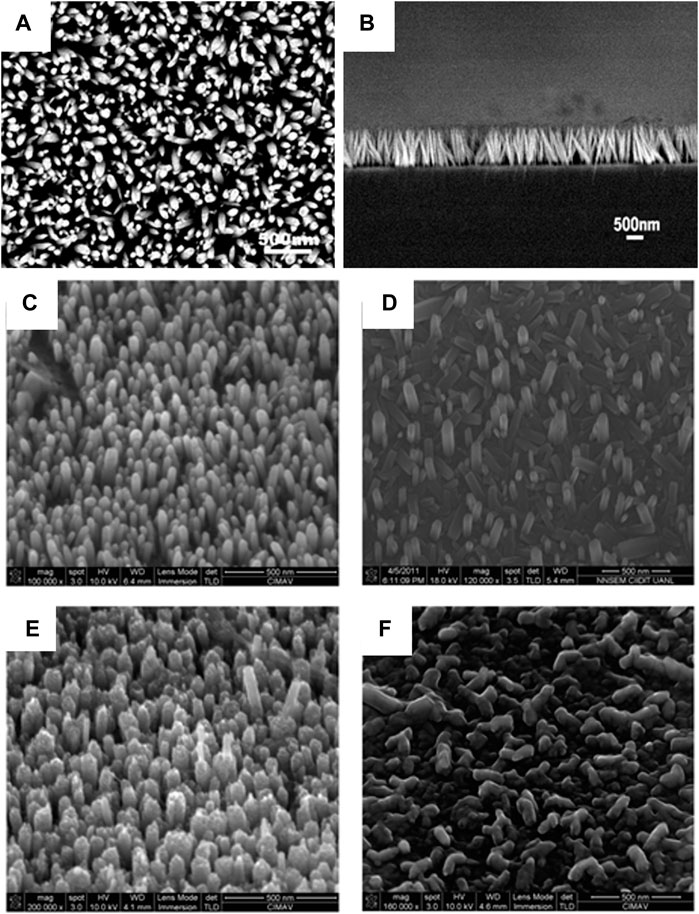
FIGURE 2. (A) Typical SEM image of ZnO nanorod arrays. (B) SEM image of the cross section of the ZnO nanorod arrays (Wang et al., 2006).; Tilted SEM images. Tilted images of ZnO nanorods grown on ALD-ZnO films of (C) 40 nm, (D) 80 nm, (E) 120 nm, and (F) 180 nm grown at 90°C, 4 h (Solís-Pomar et al., 2011).
Growth of Seed Layer via Atomic Layer Deposition
Atomic layer deposition is a type of Chemical Vapor Deposition (CVD), that deposits atoms layer by layer. The seed layer formed by this process has fewer defects as one atomic layer is being deposited allowing for the atoms to arrange precisely. The thickness of the seed layer plays an important role in the growth of the nanorods. The optimum thickness is required for the growth to occur properly. This has been observed, by Solís-Pomar et al. (Solís-Pomar et al., 2011) who reported the deposition of ZnO films with different thicknesses, 40, 80, 120, and 180 nm on Si (100) substrates by ALD and the subsequent nanorod growth in 0.01 M of zinc nitrate and 0.01 M hexamine solution placed in a water bath at 90°C for 4 h. They observed that the texture of the seed layer determines the growth of the nanorods, as the long term ALD (more thick–120 nm) seed layer promotes the growth of nanorods, as shown in Figures 2C–F.
Growth of Zinc Oxide Rods on Glass Substrates
The glass substrates include ordinary glass or some coated glass slides (ITO, FTO). The coated glass slides offer the distinct advantage of being conductive and as such the growth of the nanorods can be done on these substrates and provide an electrical application as they can work as electrodes in various devices (Umar et al., 2009) (solar cells or sensors).
Growth of Seed Layer via Drop Casting
Drop casting, requires the use of less sophisticated equipment, as the solution is deposited on the substrates and then dried either by heating or leaving it to air dry. Using this method the thickness of the layer cannot be accurately controlled and can affect the overall crystallinity and growth of the Nanorods (Aé et al., 2010; Farooqi and Srivastava, 2019). Q. Hussain et al. (Hussain et al., 2015) deposited the seed layer using a solution of 5 mM Zinc acetate dihydrate and 5 mM anhydrous KOH via drop casting. The growth of ZnO Nanorods occurs in 25 mM Zinc Nitrate 0.025 M Hexamethylene Tetramine (HMTA) and 0.1 M poly-ethylenimine (PEI) at 90°C for 1–4 h. When the growth time increases from 1 to 2 h the haze ratio increases (78.21%) but after a successive increase in the growth time the roughness also increases, causing a decrease in haze ratio, as shown in Figure 3.
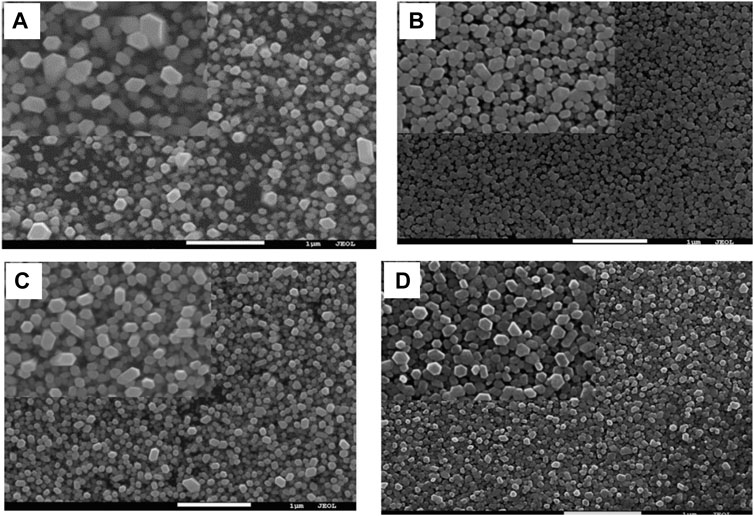
FIGURE 3. SEM images of ZnO nanorods with various growth times, Top view (A) 1 h, (B) 2 h, (C) 3 h, (D) 4 h (Hussain et al., 2015).
Growth of Seed Layer via Sputtering
S. Sarkar and D. Basak (Sarkar and Basak, 2011) deposited a ZnO seed layer of 40 nm on the ordinary glass substrate using the DC reactive magnetron sputtering technique. The growth of the nanorods occurred in 10 mM Zinc acetate di-hydrate, 10 mM HMTA, and 1 mM CTAB solution at 80°C for 12 h. The addition of Zinc metal powder during growth causes an excess of zinc metal (5, 10, 15, 20, and 30 mM) for enhancing the ultraviolet emission properties, as shown in Figure 4 (Dwivedi et al., 2020).
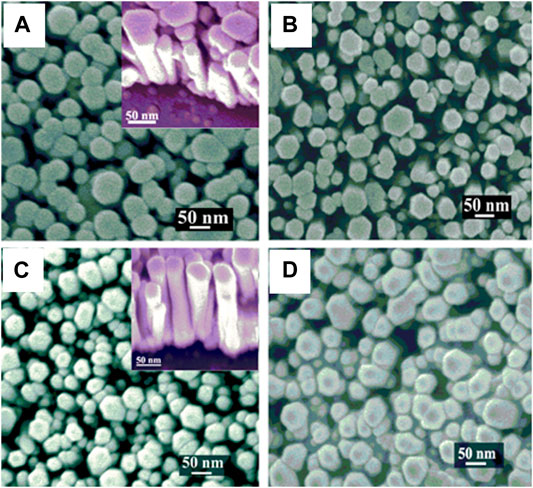
FIGURE 4. FESEM images of ZnO NRs (A) without excess zinc; (B) 5 mM excess zinc; (C) 15 mM excess zinc; (D) 30 mM excess zinc. The insets in (A) and (C) show the tilted view of the growth (Sarkar and Basak, 2011).
Other Substrates
Common substrates include silicon wafer or glass slides and polymers. The polymer substrates have additional properties of being lightweight, flexible, or biodegradable depending upon the application.
Growth of Seed Layer via Sputtering
Gaddam et al. (Gaddam et al., 2015) used Phynox alloy substrate for the growth of ZnO nanorods. The substrate was electrically conductive and biocompatible. The seed layer of 100 nm was deposited by DC reactive-magnetron sputtering and the growth was carried out in 25 mM zinc nitrate and 25 mM hexamethylenetetramine solution at growth temperatures 60°C, 70°C, 80°C, and 90°C for 4 h. An increase in the length and diameter of the ZnO nanorods was observed with an increasing growth temperature, as shown in Figure 5A–D.
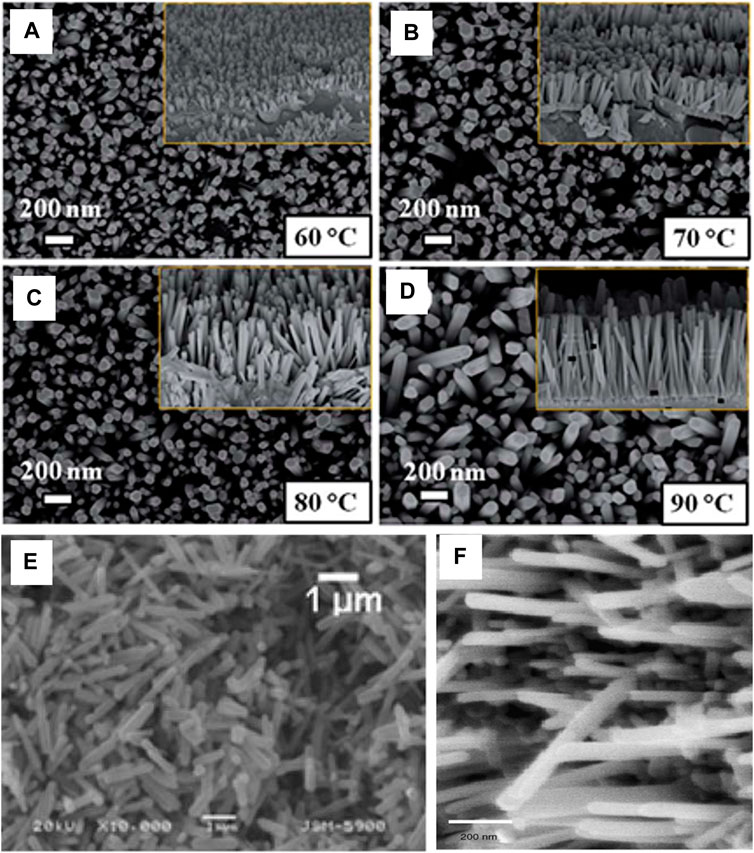
FIGURE 5. FE-SEM images for the as-grown ZnO nanorods at different growth temperatures of (A) 60°C, (B) 70°C, (C) 80°C, (D) 90°C (Note: inset figures show the corresponding cross sectional images)(Gaddam et al., 2015).; (E) SEM images of ZnO prepared in the presence of CTAB (Rai et al., 2009) (F) SEM image of the ZnO nanorods (Jiaqiang et al., 2006).
Growth of Powder
The growth of ZnO Nanorods can also be performed without a substrate and the rods grown are collected as precipitates at the end of the procedure(Bhushan et al., 2019). The growth of these nanorods without a seeded substrate requires the use of surfactant, which allows the c-axis growth which is paramount in the formation of ZnO nanorods. Jiaqiang et al. (Jiaqiang et al., 2006; Akshaykranth et al., 2020) and Prabhakar Rai (Rai et al., 2009) both synthesized ZnO nanorods in the presence of cetyltrimethylammonium bromide (CTAB) using Zinc powder at 182°C for 24 h and Zinc Nitrate at 200°C for 10 h, respectively, in the presence of ammonia. At the end of the reaction, the precipitates were collected and air dried at a temperature of 60–80°C. Duo et al. (Duo et al., 2017) synthesized ZnO hexagonal nanosheets and nanorods using Zinc Sulfate, Urea (0.05 and 0.12), and hydrazine (0.2, 0.4, 0.8, 1.5, 2.5, 5.0, and 8 ml) at 150°C for 24 h after the reaction precipitates were collected and dried at 60°C for 2 h, as shown in Figures 5E,F.
Chemical Bath Deposition
Chemical Bath Deposition (CBD) requires only solution containers and substrate mounting devices. It involves two steps, nucleation and particle growth, and is based on the formation of a solid phase from a solution(Abdulrahman et al., 2020; Alshamarti and Alkhayatt, 2020). The substrate is immersed in a solution containing the precursors. This method depends upon parameters like Bath temperature, pH of the solution, molarity, and time. Boukous et al. (Boukos et al., 2007) reported the growth of ZnO nanorods on Si (111) substrate (with and without deposition of ZnO seed layer) and glass slides. The seed layer of ZnO (100 nm) was deposited using an electron beam in the presence of Zinc Metal foil and formamide for 1–24 h, and temperature was varied (40–80°C). Their results indicate that the most defects occur in the bare Si wafer (without seed layer) while all other samples show lower defects at higher temperatures (Ungula and Swart, 2019). Table 2 gives a summary of the chemical bath deposition of ZnO nanorods.
Growth of Zinc Oxide Nanorods on Silicon Wafer
Growth of Seed Layer via Sputtering
Qu et al. (Qu et al., 2017) used polycrystalline Silicon (20 × 20*0.2 mm3) as the substrate for the growth of ZnO nanorods, as shown in Figures 6A–C. The seed layer of ZnO (about 50 nm) is deposited using RF magnetron Sputtering (Power = 75 W and Ar pressure = 6 mTorr). The substrate with the seed layer facing downwards was placed in the precursor solution (Zinc Nitrate hexahydrate and HMTA) at 99°C for 1 h to grow the ZnO nanorods.
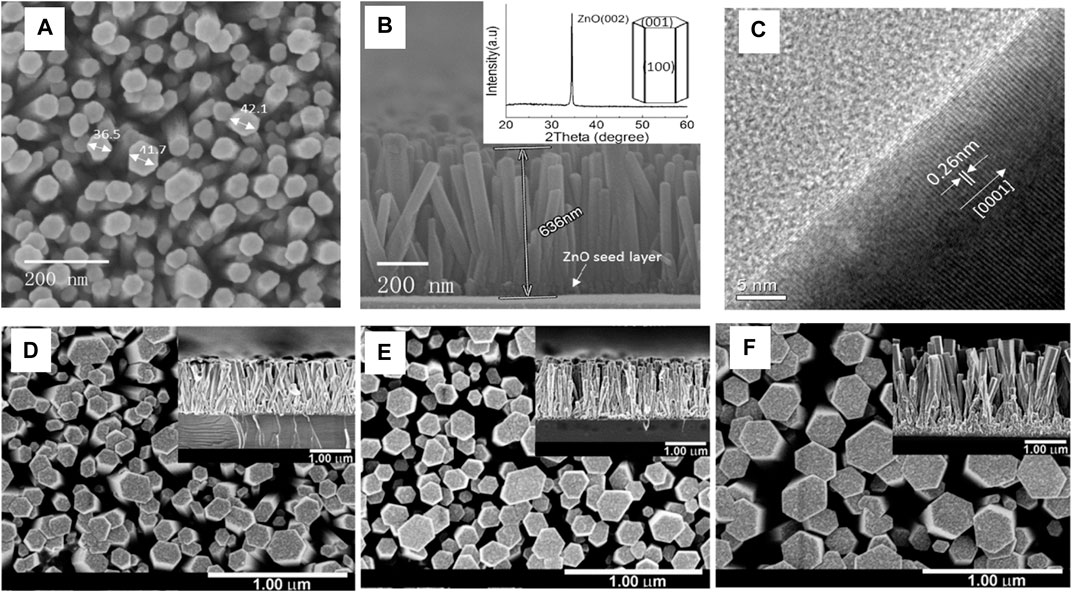
FIGURE 6. (A) A typical top-view SEM image of ZnO nanorod arrays; (B) A typical cross-sectional SEM image of ZnO nanorod arrays (inset shows a typical X-ray diffraction pattern of ZnO nanorod arrays and theoretical growth habit of ZnO crystal) (C) A typical plane-view HRTEM image of a single ZnO nanorod detached from the sample (Qu et al., 2017); Top-view and cross-sectional (inset) FE-SEM images of ZnO nanorods grown on 13 ZnO seed layers deposited for (D) 10, (E) 20 and (F) 30 min (Yu et al., 2017).
Growth of Seed Layer via Pulsed LASER Deposition
Yu et al. (Yu et al., 2017) used a N-type Si (100) wafer and deposited thin ZnO films (for 10, 20 and 30 min) via PLD, as shown in Figures 6D–F. For nanorod’s growth, they used Zinc metal foils as a source of formamide solution and the bath temperature was kept constant at 65°C for 24 h and annealed at 600°C for 1 h.
Growth of Zinc Oxide Nanorods on Glass Substrate
Growth of Seed Layer via Dip Coating
The dip coating method, as the name suggests, requires the substrates to be dipped in the seed solution for a short period. The dipping action is performed for a short time and the substrate is taken out and is either air dried, heated, or a blow of N2 inert gas is used after every successive dipping step followed by annealing. Senthil et al. (Senthil et al., 2013) fabricated the ZnO seed layer on ITO (Indium Tin Oxide) coated glass substrate by dipping it in 0.2 M zinc acetate dihydrate dissolved in a 10 ml ethanol and 0.2 M ethanolamine and then the substrates were annealed at 200°C for 30 min. Growth of ZnO nanorods is carried out by placing the seeded substrate vertically in an aqueous solution of 0.1 M zinc nitrate and 1 M hexamine at four different temperatures [1) 70°C, 2) 80°C, 3) 90°C, and 4) 100°C], and are subsequently annealed at 450°C for 30 min.
Growth of Seed Layer via Spin Coating
Zhaolin Yuan (Yuan, 2015) produced a Gold/Zinc Oxide nanorods array based schottky barrier diode by evaporating Gold (Au - 150 nm) under pressure (2.2 × 10–3 Pa) on top of ZnO nanorods, as shown in Figures 7A,B. The seed layer is deposited by spin coating the seed layer solution (5 mM Zinc Acetate and ethanol) on ITO substrate and annealed at 350°C for 20 min. The substrates were then immersed in 0.05 M Zinc nitrate and HMTA in a flask at 93°C for 1 h, air drying at 50°C.
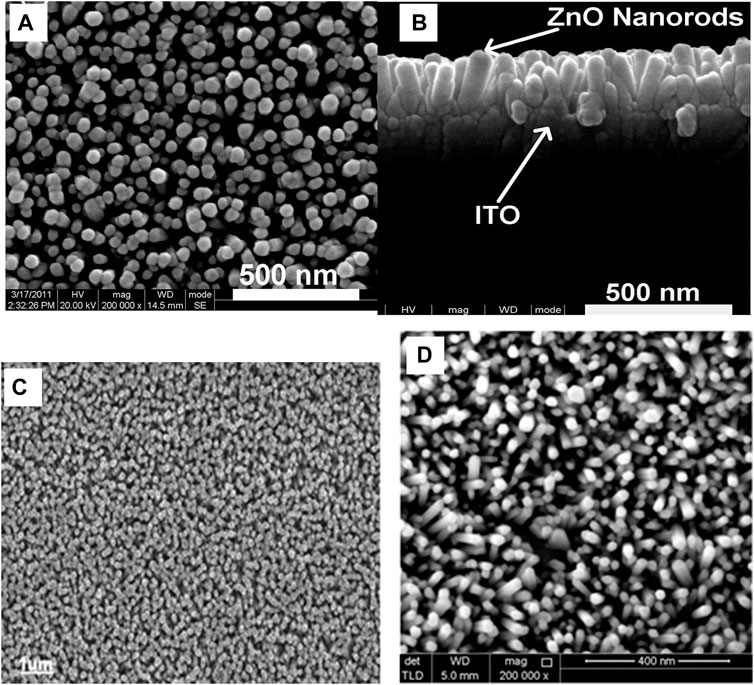
FIGURE 7. (A) high-resolution top-view FESEM images of the densely packed aligned ZNRA, and (B) cross-sectional FESEM images of the ZNRA. (Yuan, 2015); (C) Field emission scanning electron micrographs (SEM) of a ZnO nanorods grown at 50°C on TFB/PFO polymer layers on top of PEDOT:PSS/plastic flexible substrate (Zainelabdin et al., 2010), (D) Surface FESEM image of the ZnO nanorods on PEN substrate (Shabannia and Hassan, 2014).
Other Substrates
Chemical bath deposition of ZnO nanorods on polymer or graphite substrates has also been reported. The shift from conventional substrates is due to the requirement of some specific property that these substrates provide because of the focused application for the ZnO nanorods. Zainelabdin et al. (Zainelabdin et al., 2010) reported using PEDOT:PSS substrate and coated two polymers by spin coating. TFB (poly (9,9-dioctylfluorene-co–N-(4-butylpheneylamine) diphenylamine) is spin coated first and then PFO (poly (9,9-dioctyl-fluorene)) polymer is spin coated on TFB, as shown in Figure 7C. ZnO nanoparticles are then spin coated on top and placed in a chemical bath of 0.15 M Zinc Nitrate hexahydrate and 0.01 M HMTA as the growth medium at 50°C.
Shabannia et al. used Polyethylene Naphthalate (PEN) (Shabannia and Hassan, 2014) and Porous Silicon (PS) (Shabannia and Hassan, 2013) as substrates for the growth of ZnO nanorods. RF magnetron sputtering is employed to deposit the ZnO seed layer of thickness 70 nm and the growth occurs by placing the substrates vertically in 0.05 M zinc nitrate hexahydrate and 0.05 M HMTA at 80°C, as shown in Figure 7D.
Shabania (Shabannia, 2016) reported the effect of growth time by varying the time (2, 3.5, 5, and 8 h) which affected diameter, varying from 22 to 55 nm from 2 to 8 h, while the nanorods formed at 5 h had better surface morphology), as shown in Figure 8.
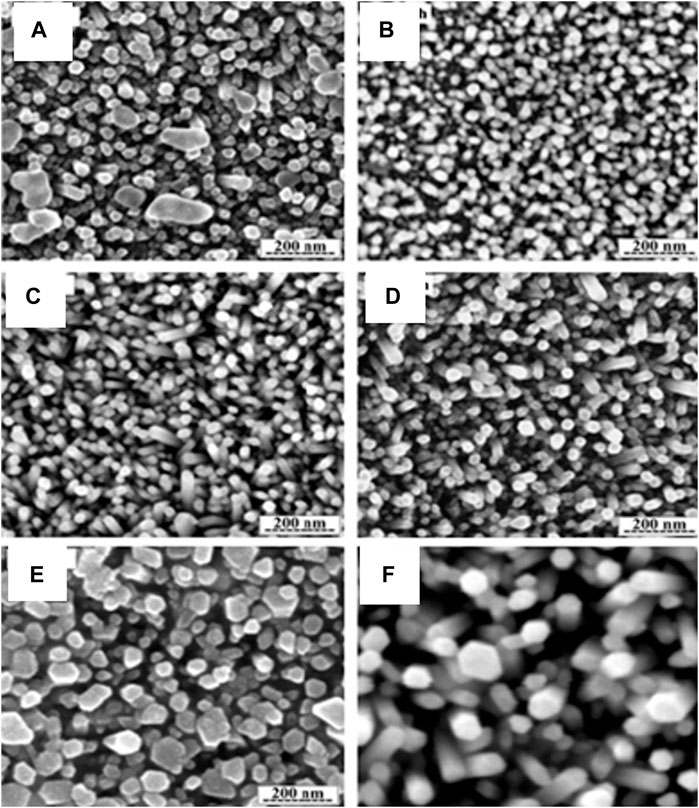
FIGURE 8. FESEM images of the ZnO nanorods with 0.050 M for different growth time: (A) 2, (B) 3.5, (C) 5, (D) 6.5, (E) 8 h and (F) show the hexagonal shape of ZnO nanorods (Shabannia, 2016).
B. Yuliarto et al. (Yuliarto et al., 2017) developed a SO2 sensor on alumina substrate with a Silver inter-digitated array electrode. The substrate was dip coated in seed solution (0.2 M Zinc Acetate tetrahydrate in ethylene glycol and Diethanol Amine) and dried at 120°C for 15 min and annealed at 450°C for 30 min. The bath precursor for growth included 0.015 M Zinc Nitrate hexahydrate in 3:1 Deionized Water:ethanol and 0.02 M Hexamethylene Tetramine (HMTA). The Seeded substrate was immersed in the bath for 1 h at 90°C and Annealed at 450°C for 1 h. Two-Times CBD sample is obtained by immersing the substrate obtained after annealing in the bath for 16 h and then annealed again. For a three time CBD sample the process of immersing the substrate was carried out again for 16 h at 90°C, as shown in Figure 9A.
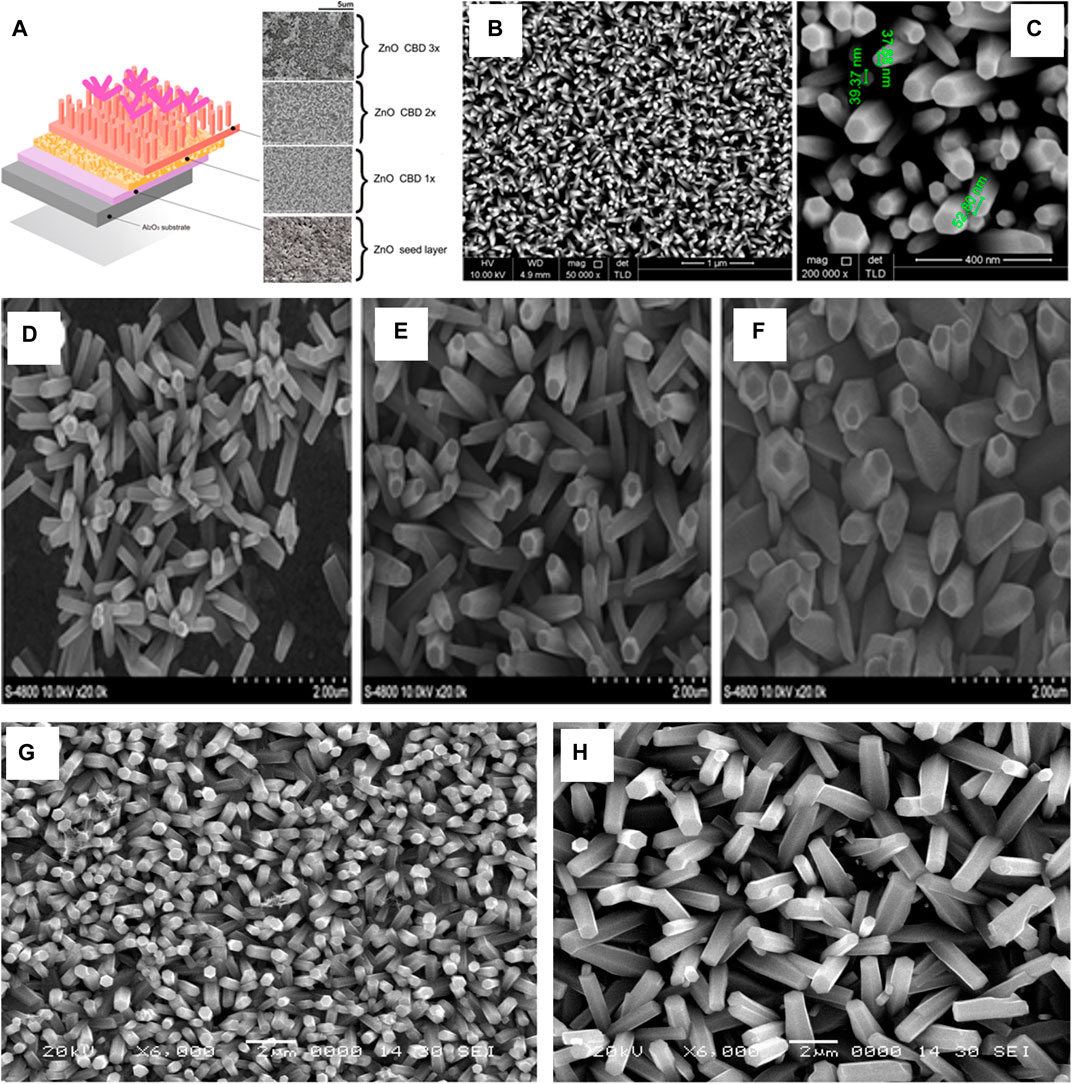
FIGURE 9. (A) The SEM of layer by layer of ZnO nanorod thin films in different times of deposition (Yuliarto et al., 2017) (B,C) FESEM images of the ZnO nanorods grown on ZnO seed layer/PTFE substrates (Farhat et al., 2015); SEM image of Substrate subjected to different densities of deposition current: (D) 0.1 mA/cm2,(E) 0.6 mA/cm2, (F)1.2 mA/cm2 [34]. ZnO Nanorods grown on (G) 70°C and (H) 80°C (Rokade et al., 2016).
O. F. Farhat et al. (Farhat et al., 2015) reported a flexible Low power UV sensor and used Teflon (PTFE) as a substrate and RF Magnetron Sputtering (Power = 150 W, Ar Pressure = 5.5 mTorr, Temperature = 200°C). After deposition of the seed layer, the substrate was annealed at 200°C for 1 h. The growth occurred by placing this annealed substrate vertically in 0.05 M Zinc Nitrate and 0.05 M Hexamethylene Tetramine (which were heated separately at 80°C before mixing them). The bath temperature was maintained at 95°C for 3 h for the growth of the Nanorods.
Z. Zhang et al. (Zhang et al., 2013) reported growth of ZnO Nanorods, on graphite substrate using a ceramic ZnO target to deposit the seed layer on the substrate using RF magnetron sputtering (Power = 120 W and Ar Pressure = 3.0 Pa) and growth was performed in equimolar (25 mM) of Zinc Acetate Nanorods and Hexamethylene Tetramine at 95°C for 3 and 5 h, as represented in Figures 9B,C.
Electrochemical Deposition
For electrochemical deposition, the basic requirement for the electrolytic path to be completed is that the substrate should be conductive (Wang et al., 2019; Mohamed et al., 2020; Zhou et al., 2020). Gao et al. (Gao et al., 2007) reported the growth of ZnO Nanorods by Electrodeposition, on a Glass substrate exclusively for a seed layer by 20 deposition cycles. T. H. C. Son et al. (Son et al., 2014) studied the effect of current densities for different periods on the growth of ZnO Nanorods on ITO substrate. The precursors for the growth were 5 mM Zinc Nitrate hexahydrate and 5 mM Hexamethylene Tetramine (HMTA). They reported a strong influence of the current density on the morphology and orientation. At a low current, the rods that formed were tilted and hexagonal in shape while the increase in current density resulted in rods that were aligned with an obelisk shape. When a sequence of low and high current density was introduced, the features became controllable. A. Rokade et al. (Rokade et al., 2016) reported the controlled growth of ZnO Nanorods in Chlorine medium rather than the acetate or nitrate medium conventionally used. They deposited the nanorods at two different bath temperatures [1) 70°C, 2) 80°C] in 5 mM Zinc Chloride and 50 mM Potassium Chloride. The effect of temperature was observed, as the increase in temperature improved crystallinity, and led to an increase in diameters of the rods formed, as shown in Figures 9D–H. Table 3 presents a summary of reported work on electrochemical deposition of ZnO nanorods.
Microwave Irradiation
Denthaje Krishna Bhat (Bhat, 2008) reported a microwave assisted growth method for the synthesis of ZnO Nanorods, using a molar ratio of 1:4 of Zinc Acetate to hydrazine Hydrate. The mixture is irradiated (Power = 150 W, Time = 10 min) leading to clear white precipitates, which are filtered, washed, and dried (60°C for 4 h) in a vacuum, as shown in Figure 10A.
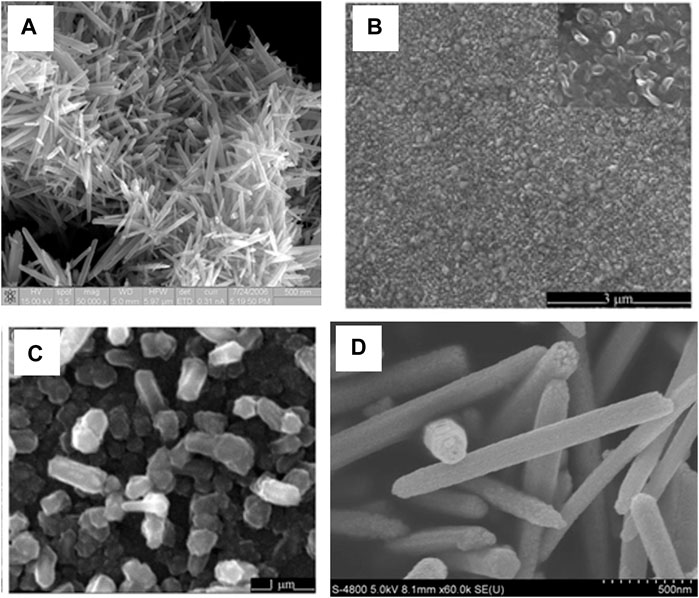
FIGURE 10. (A) FESEM image of ZnO Nanorods (Bhat, 2008); SEM image of Seed layer (B) and ZnO nanorods (C) (Yu et al., 2009); (D) SEM image of ZnO nanorods formed (Li et al., 2011).
Pulsed LASER Deposition
In this method, a pulsing LASER hits the target and then forms a plume, which is then condensed on a substrate. Qi et al. (Yu et al., 2009) used Indium phosphide (InP) substrate and deposited the ZnO thin film as a seed layer via the RF sputtering method. The growth of ZnO nanorods occurs using a ceramic ZnO target while the substrate temperature is 450°C for 90 min, as represented in Figures 10B,C.
Sol-Gel Reflux Method
The sol-gel reflux method allows for better temperature control than the commonly used Chemical bath deposition. The reflux allows for the temperature to be maintained in the case of the sol-gel reflux method. The growth results in precipitates of ZnO Nanorods being formed. The difficulty can be observed in growth on the substrate as the substrate has to be placed in the bottom of solution container for growth to occur (Amakali et al., 2020; Babar et al., 2020). Li et al. (Li et al., 2011) reported a synthesis method that yields ZnO nanorod growth in the solution of 2 mM zinc acetate dodecyl benzene sulfuric acid sodium salt (DBS), dimethyl benzene, and hydrazine monohydrate (diluted by ethanol) stirred for 1 h, then boiled, and then refluxed for 5 h. The precipitates are filtered, washed, and dried (at 70°C in air), as shown in Figure 10D.
Other Techniques
Other techniques for the synthesis of ZnO nanorods include spray pyrolysis, thermal evaporation, and chemical vapor deposition. One Step pyrolytic synthesis of ZnO nanorods involves the precursor zinc acetate, whose pyrolysis occurs at 550°C(Huang et al., 2015). Pneumatic spray pyrolysis technique with precursors: ZnCl2 and thiocarbamide has been established and leads to the formation of thinner ZnO nanorods(Dedova et al., 2007). A thermal evaporation process on a patterned Si wafer (110) which has silver (Ag) is a template for growth (Panda and Jacob, 2009), at 700°C for 2 h on Nickel based buffer layer (Sputtered layer and Spin coated) on Silicon wafer and sapphire substrate (Kuo et al., 2012). Chemical Vapor Deposition, for growth of ZnO nanorods has also been established using ZnSe particles as the seed layer (Guo et al., 2006). Catalyst free growth on Si substrates has also been reported (Wang et al., 2008). Vertically aligned epitaxial ZnO nanorods have been grown using a Diethyl zinc and oxygen environment on seeded Alumina substrate (Park et al., 2004), as shown in Figure 11.
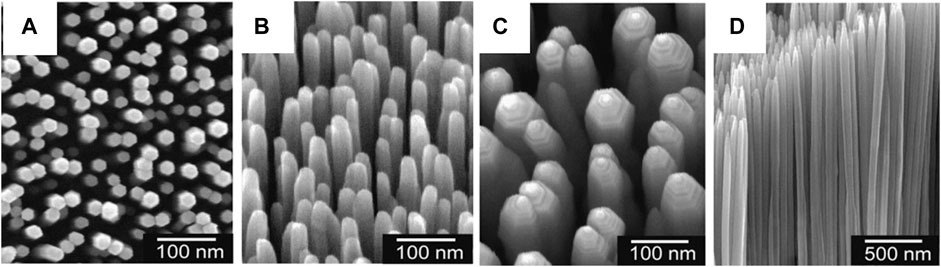
FIGURE 11. FESEM images of Aligned ZnO nanorods (Park et al., 2004).
Modification of Zinc Oxide Nanorods
The elementary ZnO properties can be improved by modifying the surface or doping the ZnO Nanorods with different entities. The improved properties result in various improved properties of the device based on Nanorods. The photochemical properties of ZnO nanorods are affected by sensitizing the rods with lead sulfide (PbS) nanoparticles. The ZnO nanorods are synthesized on Soda lime and FTO (Fluorine doped Tin oxide) coated glass substrates, and synthesis occurred via the reflux method and PbS thin films were deposited by the SILAR (successive ionic layer adsorption and reaction) method (Khot et al., 2015). Liquid Junction solar cells based on ZnO/Al:ZnO/ZnS or ZnSe/CZTS (Copper–zinc–tin–sulfide), lead to efficient photon absorption and shorter pathways for charge transport (Akram et al., 2016). A one-dimensional nanoarray of ZnO/CuInxGa1−xSe2 allows for better carrier separation and transport without using a toxic CdS buffer layer (Akram et al., 2015).
Sensing Applications
Ultraviolet (UV) light sensing can be performed using ZnO Nanorods, when the light shines on ZnO, whose direct band gap is 3.3eV, equivalent to UV energy, it allows the system (ZnO) to experience an increase in conductivity. The increase in the conductivity correlates to the intensity of the UV light. Gas sensing can also be performed using ZnO Nanorods. The gasses that oxidize can be sensed, as, when they chemically interact they induce a change in conductivity, just as in the case of UV, and are therefore sensed. A volatile organic compound sensor, that works at room temperature by doping ZnO Nanorods with Palladium in an electrochemical deposition technique, using the precursors Zinc chloride, potassium chloride, palladium acetate, dimethyl sulfoxide (DMSO) has been reported (Öztürk et al., 2016). Similarly, Hydrogen gas can be sensed by loading Platinum-Gold (Pt-Au) nanoparticles on ZnO Nanorods. The loading was done by ultra-sonicating the nanorods in presence of Pt-Au nanoparticle suspension. This loading improves the gas sensing properties of the Nanorods by several orders compared to sensing response observed in pure ZnO or ZnO supported Au and/or ZnO supported Pt (Fan et al., 2017). Core shell nanorods of Copper oxide and Zinc Oxide (CuO: ZnO) synthesized using chloride salts of Zinc and Copper, have been reported as a selective sensor (nanorods are deposited on flat silver electrode) with a fast response to selective bicarbonate in the buffer system (Rahman and Asiri, 2015) as shown in Figures 12A,B.
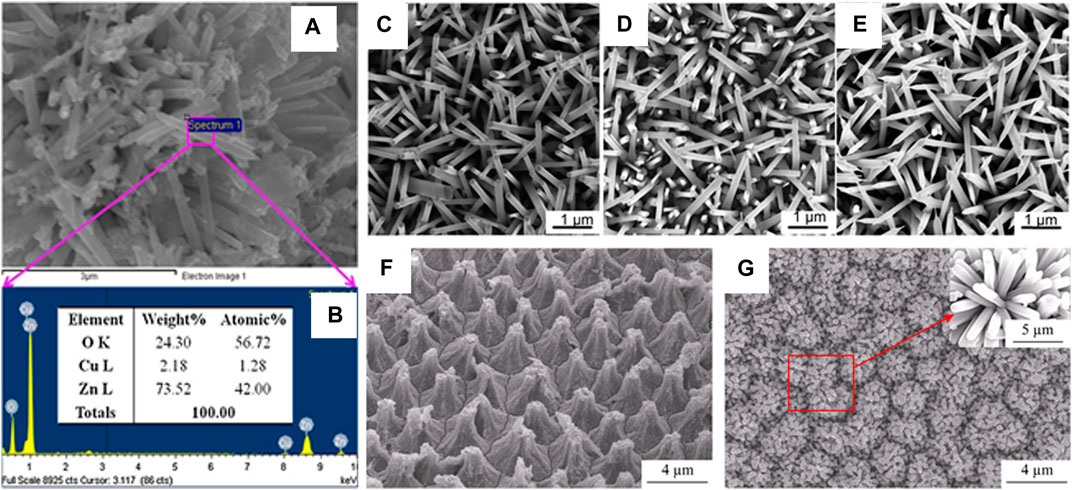
FIGURE 12. (A,B) Elemental analysis by XEDS of doped CuO-ZnO nanorods (Rahman and Asiri, 2015).; The SEM of Na-doped ZnO nanorods with doping concentrations. (C) 0% (D) 0.5 at. % (E) 2.1 at.% (Ye et al., 2017); SEM images of a PSP (F) and (G) Mn-doped ZnO/PSPA (Mn-to-Zn molar ratio of 2.0:100.0) (Wu et al., 2017).
Improvement in Opto-Electronic Properties
The Opto-electronic properties allow for the application of ZnO Nanorods in the field of LEDs etc. Doping of Sodium (Na) on ZnO nanorods grown on Silicon substrates has been reported to improve the optoelectronic properties. The improvement occurs because of the formation of an acceptor, which introduces p-type conductivity (Ye et al., 2017), as shown in Figures 12C–E.
The growth of Mn-doped ZnO nanorods on a Porous silicon pillar array (PSPA) is shown in Figure 12F,G, and it can potentially add a red section of the visible emission, improving the color rendering index of ZnO(Wu et al., 2017).
Conclusion
This paper provides an overview of a variety of solution-based techniques and touches on a few physical techniques that have been developed for fabricating ZnO Nanorods. Every technique offers a certain advantage and has inescapable weaknesses or disadvantages. This paper compares the effect of time, temperature, and various salts on the morphology and structure of the nanorods and summarizes those in tabulated form. If the various factors in these solutions-based techniques are manipulated to the fullest, low-cost growth of ZnO Nanorods is possible, which is highly desirable in future research and applications.
Author Contributions
All authors listed have made a substantial, direct, and intellectual contribution to the work and approved it for publication.
Funding
This work is funded by HEC NRPU grant no. 6014.
Conflict of Interest
The authors declare that the research was conducted in the absence of any commercial or financial relationships that could be construed as a potential conflict of interest.
References
Abdelouhab, Z. A., Djouadi, D., Chelouche, A., Hammiche, L., and Touam, T. J. M. S.-P. (2020). Structural and morphological characterizations of pure and Ce-doped ZnO nanorods hydrothermally synthesized with different caustic bases. Nanotechnology 38, 228–235. doi:10.2478/msp-2020-0038
Abdulrahman, A. F., Ahmed, S. M., Ahmed, N. M., and Almessiere, M. A. (2020). Enhancement of ZnO nanorods properties using modified chemical bath deposition method: effect of precursor concentration. Crystals 10, 386. doi:10.3390/cryst10050386
Aé, L., Kieven, D., Chen, J., Klenk, R., Rissom, T., Tang, Y., et al. (2010). ZnO nanorod arrays as an antireflective coating for Cu(In,Ga)Se2thin film solar cells. Prog. Photovolt: Res. Appl. 18, 209–213. doi:10.1002/pip.946
Akram, M. A., Javed, S., Islam, M., Mujahid, M., and Safdar, A. (2016). Arrays of CZTS sensitized ZnO/ZnS and ZnO/ZnSe core/shell nanorods for liquid junction nanowire solar cells. Solar Energ. Mater. Solar Cell 146, 121–128. doi:10.1016/j.solmat.2015.11.034
Akram, M. A., Javed, S., Xu, J., Mujahid, M., and Lee, C.-S. (2015). Arrays of ZnO/CuInxGa1−xSe2 nanocables with tunable shell composition for efficient photovoltaics. J. Appl. Phys. 117, 205306. doi:10.1063/1.4921825
Akshaykranth, A., Rao, T. V., and Kumar, R. R. (2020). Growth of ZnO nanorods on biodegradable poly (lactic acid) (PLA) substrates by low temperature solution method. Mater. Lett. 259, 126807. doi:10.1016/j.matlet.2019.126807
Al-Asedy, H. J., and Al-Khafaji, S. a. J. a. P. A. (2020). Gallium-doped zinc oxide films with diverse nanomorphologies grown via sol–gel united spin coating technique. Appl. Phys. A. 126, 1–10. doi:10.1007/s00339-020-03891-x
Alshamarti, H. A., and Omran Alkhayatt, A. H. (2020). Enhancement characterization of the MSM detector based on Mn doped-ZnO NRS synthesized by microwave assisted chemical bath deposition. Mater. Sci. Semiconductor Process. 114, 105068. doi:10.1016/j.mssp.2020.105068
Amakali, T., Daniel, L. S., Uahengo, V., Dzade, N. Y., and De Leeuw, N. H. (2020). Structural and optical properties of ZnO thin films prepared by molecular precursor and sol-gel methods. Crystals 10, 132. doi:10.3390/cryst10020132
Babar, U., Garad, N., Mohite, A., Babar, B., Shelke, H., Kamble, P., et al. (2020). Study the photovoltaic performance of pure and Cd-doped ZnO nanoparticles prepared by reflux method. doi:10.1016/j.matpr.2020.08.008
Bhat, D. K. (2008). Facile synthesis of ZnO nanorods by microwave irradiation of zinc-hydrazine hydrate complex. Nanoscale Res. Lett. 3, 31. doi:10.1007/s11671-007-9110-4
Bhushan, B., Murty, B. S., and Mondal, K. (2019). A new approach for synthesis of ZnO nanorod flowerets and subsequent pure free-standing ZnO nanorods. Adv. Powder Tech. 30, 30–41. doi:10.1016/j.apt.2018.10.004
Boukos, N., Chandrinou, C., Giannakopoulos, K., Pistolis, G., and Travlos, A. (2007). Growth of ZnO nanorods by a simple chemical method. Appl. Phys. A. 88, 35–39. doi:10.1007/s00339-007-3940-x
Cittadini, M., Sturaro, M., Guglielmi, M., Resmini, A., Tredici, I. G., Anselmi-Tamburini, U., et al. (2015). ZnO nanorods grown on ZnO sol-gel seed films: characteristics and optical gas-sensing properties. Sensors Actuators B: Chem. 213, 493–500. doi:10.1016/j.snb.2015.03.002
Dedova, T., Volobujeva, O., Klauson, J., Mere, A., and Krunks, M. (2007). ZnO nanorods via spray deposition of solutions containing zinc chloride and thiocarbamide. Nanoscale Res. Lett. 2, 391. doi:10.1007/s11671-007-9072-6
Duo, S., Li, Y., Liu, Z., Zhong, R., Liu, T., and Xu, H. (2017). Preparation of ZnO from 2 D nanosheets to diverse 1 D nanorods and their structure, surface area, photocurrent, optical and photocatalytic properties by simple hydrothermal synthesis. J. Alloys Comp. 695, 2563–2579. doi:10.1016/j.jallcom.2016.11.162
Dwivedi, J., Srivastava, A., and Kumar, N. J. T. S. F. (2020). Gold nanoparticles decorated radio-frequency sputtered ZnFe2O4/ZnO nanostructures for photoelectrochemical applications. Thin. Solid. Films. 709, 138227. doi:10.1016/j.tsf.2020.138227
Fan, F., Zhang, J., Li, J., Zhang, N., Hong, R., Deng, X., et al. (2017). Hydrogen sensing properties of Pt-Au bimetallic nanoparticles loaded on ZnO nanorods. Sensors Actuators B: Chem. 241, 895–903. doi:10.1016/j.snb.2016.11.025
Fang, X., Bando, Y., Liao, M., Gautam, U. K., Zhi, C., Dierre, B., et al. (2009). Single-Crystalline ZnS nanobelts as ultraviolet-light sensors. Adv. Mater. 21, 2034–2039. doi:10.1002/adma.200802441
Farhat, O. F., Halim, M. M., Abdullah, M. J., Ali, M. K. M., Ahmed, N. M., and Allam, N. K. (2015). Growth of vertically aligned ZnO nanorods on Teflon as a novel substrate for low-power flexible light sensors. Appl. Phys. A. 119, 1197–1201. doi:10.1007/s00339-015-9177-1
Farooqi, M. M. H., and Srivastava, R. K. (2019). Effect of annealing temperature on structural, photoluminescence and photoconductivity properties of ZnO thin film deposited on glass substrate by sol–gel spin coating method. Proc. Natl. Acad. Sci. India Section A: Phys. Sci. 90, 845–859. doi:10.1007/s40010-019-00648-x
Gaddam, V., Kumar, R. R., Parmar, M., Nayak, M. M., and Rajanna, K. (2015). Synthesis of ZnO nanorods on a flexible Phynox alloy substrate: influence of growth temperature on their properties. RSC Adv. 5, 89985–89992. doi:10.1039/c5ra12773d
Gao, X.-D., Li, X.-M., Yu, W.-D., Li, L., and Qiu, J.-J. (2007). Seed layer-free synthesis and characterization of vertically grown ZnO nanorod array via the stepwise solution route. Appl. Surf. Sci. 253, 4060–4065. doi:10.1016/j.apsusc.2006.09.002
Ghosh, R., Kundu, S., Majumder, R., and Chowdhury, M. P. (2020). Hydrothermal synthesis and characterization of multifunctional ZnO nanomaterials. Mater. Today Proc. 26, 77–81. doi:10.1016/j.matpr.2019.04.217
Guo, C., Choy, W. C. H., Leung, Y. P., Cheah, K. W., and Fang, Y. (2006). ZnO nanorods grown on ZnSe particles by the chemical vapor deposition method. Appl. Phys. A. 83, 421–425. doi:10.1007/s00339-006-3563-7
Han, J.-H., Lee, D., Chew, C. H. C., Kim, T., and Pak, J. J. (2016). A multi-virus detectable microfluidic electrochemical immunosensor for simultaneous detection of H1N1, H5N1, and H7N9 virus using ZnO nanorods for sensitivity enhancement. Sensors Actuators B: Chem. 228, 36–42. doi:10.1016/j.snb.2015.07.068
Hassanpour, A., Bogdan, N., Capobianco, J. A., and Bianucci, P. (2017). Hydrothermal selective growth of low aspect ratio isolated ZnO nanorods. Mater. Des. 119, 464–469. doi:10.1016/j.matdes.2017.01.089
Huang, M. H., Wu, Y., Feick, H., Tran, N., Weber, E., and Yang, P. (2001). Catalytic growth of zinc oxide nanowires by vapor transport. Adv. Mater. 13, 113–116. doi:10.1002/1521-4095(200101)13:2<113::aid-adma113>3.0.co;2-h
Huang, N., Shu, J., Wang, Z., Chen, M., Ren, C., and Zhang, W. (2015). One-step pyrolytic synthesis of ZnO nanorods with enhanced photocatalytic activity and high photostability under visible light and UV light irradiation. J. Alloys Comp. 648, 919–929. doi:10.1016/j.jallcom.2015.07.039
Huang, Z.-L., Chen, C.-M., Lin, Z.-K., and Yang, S.-H. (2017). Efficiency enhancement of regular-type perovskite solar cells based on Al-doped ZnO nanorods as electron transporting layers. Superlattices and Microstructures 102, 94–102. doi:10.1016/j.spmi.2016.12.012
Hussain, S. Q., Yen, C., Khan, S., Kwon, G. D., Kim, S., Ahn, S., et al. (2015). Uniform 3D hydrothermally deposited zinc oxide nanorods with high haze ratio. Mater. Sci. Semiconductor Process. 37, 99–104. doi:10.1016/j.mssp.2015.02.024
Jayaprakash, N., Suresh, R., Rajalakshmi, S., Raja, S., Sundaravadivel, E., Gayathri, M., et al. (2020). One-step synthesis, characterisation, photocatalytic and bio-medical applications of ZnO nanoplates. Mater. Tech. 35, 112–124. doi:10.1080/10667857.2019.1659533
Jiaqiang, X., Yuping, C., Daoyong, C., and Jianian, S. (2006). Hydrothermal synthesis and gas sensing characters of ZnO nanorods. Sensors Actuators B: Chem. 113, 526–531. doi:10.1016/j.snb.2005.03.097
Jung, K., Lim, T., and Martinez-Morales, A. A. (2020). CuO shell as a protective layer to improve the stability of ZnO nanorods-based photoelectrode in DSSCs. Appl. Surf. Sci. 507, 144510. doi:10.1016/j.apsusc.2019.144510
Khot, K. V., Mali, S. S., Mane, R. M., Patil, P. S., Hong, C. K., Kim, J. H., et al. (2015). Synthesis, characterization and photoelectrochemical properties of PbS sensitized vertically aligned ZnO nanorods: modified aqueous route. J. Mater. Sci. Mater. Electron. 26, 6897–6906. doi:10.1007/s10854-015-3307-6
Kuo, D.-H., He, J.-Y., and Huang, Y.-S. (2012). Synthesis of vertically aligned ZnO nanorods on Ni-based buffer layers using a thermal evaporation process. J. Elec Materi 41, 451–456. doi:10.1007/s11664-011-1823-6
Li, W.-M., Yin, P.-G., and Guo, L. (2011). Soft template synthesis of ZnO nanorods and their optical properties. Res. Chem. Intermed 37, 125–130. doi:10.1007/s11164-011-0241-y
Lupan, O., Pauporté, T., Viana, B., Tiginyanu, I. M., Ursaki, V. V., and Cortès, R. (2010). Epitaxial electrodeposition of ZnO nanowire arrays on p-GaN for efficient UV-light-emitting diode fabrication. ACS Appl. Mater. Inter. 2, 2083–2090. doi:10.1021/am100334c
Mbuyisa, P. N., Ndwandwe, O. M., and Cepek, C. (2015). Controlled growth of zinc oxide nanorods synthesised by the hydrothermal method. Thin Solid Films 578, 7–10. doi:10.1016/j.tsf.2015.02.002
Mohamed, M., Alshammari, A. S., and Almokhtar, M. J. a. P. A. (2020). Structure, morphology and photocatalytic activity of ZnO nanorods fabricated by electrochemical deposition. Appl. Phys. A 126, 1–8. doi:10.1007/s00339-020-03786-x
Mohammad, S. M., Hassan, Z., Abd-Alghafour, N. M., Ahmed Ali, A. M., Ahmed, N. M., Abdalrheem, R., et al. (2020). Ultraviolet electroluminescence from flowers-like n-ZnO nanorods/p-GaN light-emitting diode fabricated by modified chemical bath deposition. J. Lumin. 226, 117510. doi:10.1016/j.jlumin.2020.117510
Ozgur, U., Alivov, Y. I., Liu, C., Teke, A., Reshchikov, M. A., Dogan, S., et al. (2005). A comprehensive review of ZnO materials and devices. J. Appl. Phys. 98, 041301. doi:10.1063/1.1992666
Öztürk, S., Kösemen, A., Kösemen, Z. A., Kılınç, N., Öztürk, Z. Z., and Penza, M. (2016). Electrochemically growth of Pd doped ZnO nanorods on QCM for room temperature VOC sensors. Sensors Actuators B: Chem. 222, 280–289. doi:10.1016/j.snb.2015.08.083
Panda, S. K., and Jacob, C. (2009). Catalytic synthesis of ZnO nanorods on patterned silicon wafer-An optimum material for gas sensor. Bull. Mater. Sci. 32, 493. doi:10.1007/s12034-009-0073-1
Park, W. I., Kim, J. S., Yi, G.-C., Bae, M. H., and Lee, H.-J. (2004). Fabrication and electrical characteristics of high-performance ZnO nanorod field-effect transistors. Appl. Phys. Lett. 85, 5052–5054. doi:10.1063/1.1821648
Park, W. I., Yi, G.-C., Kim, M. Y., and Pennycook, S. J. (2003). Quantum confinement observed in ZnO/ZnMgO nanorod heterostructures. Adv. Mater. 15, 526–529. doi:10.1002/adma.200390122
Qiaoping, L., Xin, L., Yanning, Y., Fuchun, Z., and Qi, Z. (2020). Hydrothermal synthesis and optical properties of Fe doped ZnO nanorods. Ferroelectrics 564, 59–69. doi:10.1080/00150193.2020.1761702
Qu, Y., Huang, X., Li, Y., Lin, G., Guo, B., Song, D., et al. (2017). Chemical bath deposition produced ZnO nanorod arrays as an antireflective layer in the polycrystalline Si solar cells. J. Alloys Comp. 698, 719–724. doi:10.1016/j.jallcom.2016.12.265
Rahman, M. M., and Asiri, A. M. (2015). Development of selective and sensitive bicarbonate chemical sensor based on wet-chemically prepared CuO-ZnO nanorods. Sensors Actuators B: Chem. 214, 82–91. doi:10.1016/j.snb.2015.02.113
Rai, P., Tripathy, S. K., Park, N.-H., O, K.-J., Lee, I.-H., and Yu, Y.-T. (2009). Synthesis of violet light emitting single crystalline ZnO nanorods by using CTAB-assisted hydrothermal method. J. Mater. Sci. Mater. Electron. 20, 967–971. doi:10.1007/s10854-008-9816-9
Ray, P. G., Das, M., Wan, M., Jacob, C., Roy, S., Basak, P., et al. (2020). Surfactant and catalyst free facile synthesis of Al-doped ZnO nanorods–An approach towards fabrication of single nanorod electrical devices. Appl. Surf. Sci. 512, 145732. doi:10.1016/j.apsusc.2020.145732
Rokade, A., Rondiya, S., Gabhale, B., Diwate, K., Karpe, S., Mayabadi, A., et al. (2016). Electrodeposition of template free hierarchical ZnO nanorod arrays via a chloride medium. J. Mater. Sci. Mater. Electron. 27, 12357–12364. doi:10.1007/s10854-016-5447-8
Roza, L., Febrianti, Y., Iwan, S., and Fauzia, V. (2020). The role of cobalt doping on the photocatalytic activity enhancement of ZnO nanorods under UV light irradiation. Surf. Inter. 18, 100435. doi:10.1016/j.surfin.2020.100435
Sanguino, P., Monteiro, T., Bhattacharyya, S. R., Dias, C. J., Igreja, R., and Franco, R. (2014). ZnO nanorods as immobilization layers for interdigitated capacitive immunosensors. Sensors Actuators B: Chem. 204, 211–217. doi:10.1016/j.snb.2014.06.141
Sannakashappanavar, B. S., Byrareddy, C., Varma, S., Pattanshetti, N. A., and Yadav, A. B. (2020). “Deposition of ZnO thin film at different substrate temperature using RF sputtering for growth of ZnO nanorods using hydrothermal method for UV detection,” in Control instrumentation systems (Springer), 91–98. doi:10.1007/978-981-13-9419-5_8
Sarkar, S., and Basak, D. (2011). A low temperature in situ facile technique to enhance ultraviolet emission of zinc oxide nanorods and its mechanistic insights. Chem. Phys. Lett. 516, 192–198. doi:10.1016/j.cplett.2011.09.070
Senthil, T., Kim, A.-Y., Muthukumarasamy, N., and Kang, M. (2013). Effect of bath temperature on the performance of ZnO nanorod-based thin film solar cells. J. nanoparticle Res. 15, 1926. doi:10.1007/s11051-013-1926-5
Shabannia, R., and Abu Hassan, H. (2013). Growth and characterization of vertically aligned ZnO nanorods grown on porous silicon: effect of precursor concentration. Superlattices and Microstructures 62, 242–250. doi:10.1016/j.spmi.2013.07.025
Shabannia, R. (2016). Effects of growth duration and precursor concentration on the growth of ZnO nanorods synthesized by chemical bath deposition. Iran. J. Sci. Technol. Trans. Sci. 40, 19–25. doi:10.1007/s40995-016-0005-z
Shabannia, R., and Hassan, H. A. (2014). Characteristics of photoconductive UV photodetector based on ZnO nanorods grown on polyethylene naphthalate substrate by chemical bath deposition method. Electron. Mater. Lett. 10, 837–843. doi:10.1007/s13391-014-3245-0
Solís-Pomar, F., Martínez, E., Meléndrez, M. F., and Pérez-Tijerina, E. (2011). Growth of vertically aligned ZnO nanorods using textured ZnO films. Nanoscale Res. Lett. 6, 524. doi:10.1186/1556-276x-6-524
Son, T. H. C., Top, L. K., Tri, N. T. D., Nhan, H. T. C., Vinh, L. Q., Phan, B. T., et al. (2014). Control of morphology and orientation of electrochemically grown ZnO nanorods. Met. Mater. Int. 20, 337–342. doi:10.1007/s12540-014-2013-x
Srivastava, S., Srivastava, A. K., Singh, P., Baranwal, V., Kripal, R., Lee, J.-H., et al. (2015). Synthesis of zinc oxide (ZnO) nanorods and its phenol sensing by dielectric investigation. J. Alloys Comp. 644, 597–601. doi:10.1016/j.jallcom.2015.04.220
Sutradhar, S., Bandyopadhyay, A., Debnath, T., Chakraborty, T., Majumdar, S., Chakraborty, S., et al. (2020). Effect of hydrothermal synthesis on physical property modulation and biological activity of ZnO nanorods. Mater. Res. Express 6, 1250f7. doi:10.1088/2053-1591/ab4dd0
Tang, C., Sun, F., Chen, Z., Yu, H., Chen, D., and Liu, Z. (2020). Facile synthesis and nanoscale related physical properties of core-shell structured CuO/ZnO nanorods on Si substrate. Appl. Surf. Sci. 509, 144903. doi:10.1016/j.apsusc.2019.144903
Thambidurai, M., Muthukumarasamy, N., Velauthapillai, D., Lee, C., and Kim, J. Y. (2012). Synthesis of ZnO nanorods and their application in quantum dot sensitized solar cells. J. Sol-gel Sci. Technol. 64, 750–755. doi:10.1007/s10971-012-2907-3
Umar, A., Ribeiro, C., Al-Hajry, A., Masuda, Y., and Hahn, Y. B. (2009). Growth of highly c-axis-oriented ZnO nanorods on ZnO/glass substrate: growth mechanism, structural, and optical properties. J. Phys. Chem. C. 113, 14715–14720. doi:10.1021/jp9045098
Ungula, J., and Swart, H. C. (2019). Structural, morphological and optical properties of ZnO nanorods grown on a ZnO:Ga seeded thin film: the role of chemical bath deposition precursor concentration at constant and varying II/VI molar ratios. Thin Solid Films 687, 137483. doi:10.1016/j.tsf.2019.137483
Wang, C., Wang, L.-J., Zhang, L., Xi, R., Huang, H., Zhang, S.-H., et al. (2019). Electrodeposition of ZnO nanorods onto GaN towards enhanced H2S sensing. J. Alloys Comp. 790, 363–369. doi:10.1016/j.jallcom.2019.03.084
Wang, H., Zhang, Z. P., Wang, X. N., Mo, Q., Wang, Y., Zhu, J. H., et al. (2008). Selective growth of vertical-aligned ZnO nanorod arrays on Si substrate by catalyst-free thermal evaporation. Nanoscale Res. Lett. 3, 309. doi:10.1007/s11671-008-9156-y
Wang, J. X., Sun, X. W., Yang, Y., Huang, H., Lee, Y. C., Tan, O. K., et al. (2006). Hydrothermally grown oriented ZnO nanorod arrays for gas sensing applications. Nanotechnol. 17, 4995. doi:10.1088/0957-4484/17/19/037
Wu, Z.-L., Tian, B., and Xu, H.-J. (2017). Improving color rendering index of Mn-doped ZnO nanorods on silicon-based substrate. Rare Met. 36, 711–717. doi:10.1007/s12598-016-0786-4
Ye, Z., Wang, T., Wu, S., Ji, X., and Zhang, Q. (2017). Na-doped ZnO nanorods fabricated by chemical vapor deposition and their optoelectrical properties. J. Alloys Comp. 690, 189–194. doi:10.1016/j.jallcom.2016.08.100
Yu, D., Hu, L., Li, J., Hu, H., Zhang, H., Len, S.-E. A., et al. (2009). Self-catalyst synthesis of aligned ZnO nanorods by pulsed laser deposition. Sci. China Ser. G-phys. Mech. Astron. 52, 207–211. doi:10.1007/s11433-009-0040-2
Yu, Z., Li, H., Qiu, Y., Yang, X., Zhang, W., Xu, N., et al. (2017). Size-controllable growth of ZnO nanorods on Si substrate. Superlattices and Microstructures 101, 469–479. doi:10.1016/j.spmi.2016.12.005
Yuan, Z. (2015). Low-temperature growth of well-aligned ZnO nanorod arrays by chemical bath deposition for Schottky diode application. J. Elec Materi 44, 1187–1191. doi:10.1007/s11664-015-3661-4
Yuliarto, B., Ramadhani, M. F., Nugraha, N. L. W., Septiani, N. L. W., and Hamam, K. A. (2017). Enhancement of SO2 gas sensing performance using ZnO nanorod thin films: the role of deposition time. J. Mater. Sci. 52, 4543–4554. doi:10.1007/s10853-016-0699-5
Yun, S., Guo, T., Li, Y., Gao, X., Huang, A., and Kang, L. J. M. R. B. (2020). Well-ordered vertically aligned ZnO nanorods arrays for high-performance perovskite solar cells, 110935.
Zainelabdin, A., Zaman, S., Amin, G., Nur, O., and Willander, M. (2010). Stable white light electroluminescence from highly flexible polymer/ZnO nanorods hybrid heterojunction grown at 50°C. Nanoscale Res. Lett. 5, 1442. doi:10.1007/s11671-010-9659-1
Zhang, S., Li, H., Wang, X., Liu, Y., Dai, J., and Chen, C. (2020). Highly integrated in situ photoenergy gas sensor with deep ultraviolet LED. ACS Omega 5, 9985–9990. doi:10.1021/acsomega.0c00317
Zhang, Z., Zhang, Y., Bian, J., Sun, J., Qin, F., Wang, Y., et al. (2013). High-quality ZnO nanorods grown on graphite substrates by chemical solution method. Appl. Phys. A. 111, 1071–1076. doi:10.1007/s00339-012-7504-3
Keywords: zinc oxide, heirarchical nanostructures, nanorods, optoelectronic, nanostructures, photo anode, LED
Citation: Shahzad S, Javed S and Usman M (2021) A Review on Synthesis and Optoelectronic Applications of Nanostructured ZnO. Front. Mater. 8:613825. doi: 10.3389/fmats.2021.613825
Received: 03 October 2020; Accepted: 21 January 2021;
Published: 27 April 2021.
Edited by:
Muhammad Asif, University of Limerick, IrelandReviewed by:
Muhammad Yasir, Institute of Space Technology, PakistanKhan Abdul Sammed, Dalian University, China
Copyright © 2021 Shahzad, Javed and Usman. This is an open-access article distributed under the terms of the Creative Commons Attribution License (CC BY). The use, distribution or reproduction in other forums is permitted, provided the original author(s) and the copyright owner(s) are credited and that the original publication in this journal is cited, in accordance with accepted academic practice. No use, distribution or reproduction is permitted which does not comply with these terms.
*Correspondence: Sofia Javed, sofia.javed@scme.nust.edu.pk