- 1Department of Integrated Traditional Chinese and Western Medicine, Sichuan Provincial Pancreatitis Centre and West China-Liverpool Biomedical Research Centre, West China Hospital of Sichuan University, Chengdu, China
- 2Surgical and Translational Research Centre, Faculty of Medical and Health Sciences, University of Auckland, Auckland, New Zealand
- 3Applied Surgery and Metabolism Laboratory, School of Biological Sciences, University of Auckland, Auckland, New Zealand
- 4Liverpool Pancreatitis Research Group, Liverpool University Hospitals NHS Foundation Trust and Institute of Systems, Molecular and Integrative Biology, University of Liverpool, Liverpool, United Kingdom
- 5Division of Endocrinology and Metabolism, State Key Laboratory of Biotherapy, West China Hospital, Sichuan University and Collaborative Innovation Center of Biotherapy, Chengdu, China
- 6School of Pharmacy, University of Otago, Dunedin, New Zealand
- 7West China-Washington Mitochondria and Metabolism Center, West China Hospital, Sichuan University, Chengdu, China
Macrophage migration inhibitory factor (MIF) is a pleiotropic cytokine implicated in the pathogenesis of inflammation and cancer. It is produced by various cells and circulating MIF has been identified as a biomarker for a range of diseases. Extracellular MIF mainly binds to the cluster of differentiation 74 (CD74)/CD44 to activate downstream signaling pathways. These in turn activate immune responses, enhance inflammation and can promote cancer cell proliferation and invasion. Extracellular MIF also binds to the C-X-C chemokine receptors cooperating with or without CD74 to activate chemokine response. Intracellular MIF is involved in Toll-like receptor and inflammasome-mediated inflammatory response. Pharmacological inhibition of MIF has been shown to hold great promise in treating inflammatory diseases and cancer, including small molecule MIF inhibitors targeting the tautomerase active site of MIF and antibodies that neutralize MIF. In the current review, we discuss the role of MIF signaling pathways in inflammation and cancer and summarize the recent advances of the role of MIF in experimental and clinical exocrine pancreatic diseases. We expect to provide insights into clinical translation of MIF antagonism as a strategy for treating acute pancreatitis and pancreatic cancer.
Introduction
Macrophage migration inhibitory factor (MIF) was originally discovered in 1966 as a lymphokine derived from activated T cells during delayed-type hypersensitivity (Bloom and Bennett, 1966; David, 1966), exhibiting inhibition function of macrophage migration. Since being cloned in the early 1990s (Bernhagen et al., 1993; Bernhagen et al., 1994), numerous researchers have investigated its association with disease, multifaceted versatile functions, receptors, and downstream signaling pathways. MIF is now known to have a pivotal role in metabolic (Morrison and Kleemann, 2015), acute inflammatory (Hertelendy et al., 2018), autoimmune (Greven et al., 2010) and infectious diseases (Leaver et al., 2010), and cancers (O’reilly et al., 2016) including colorectal (He et al., 2009), malignant melanoma (Oliveira et al., 2014), lung (Tomiyasu et al., 2002), breast (Tomiyasu et al., 2002), and prostate (Meyer-Siegler et al., 2002) cancers as well as glioblastomas (Munaut et al., 2002).
Acute pancreatitis (AP) is one of the most common gastroenterological diseases with an increasing global incidence and is complicated by considerable comorbidity, mortality, and financial burden (Peery et al., 2019; Petrov and Yadav, 2019). In the course of the disease, injured pancreatic acinar cells secrete inflammatory mediators such as interleukin-6 (IL-6), tumor necrosis factor-alpha (TNF-α), interleukin-1 beta (IL-1β), MIF, chemokines and their ligands that mediate recruitment and infiltration of neutrophils and monocytes at the injury site (Lugea et al., 2017), further aggravating local injury and systemic inflammation (Linkermann et al., 2014). As a result, anti-inflammatory treatment strategies have been tested in AP (Gukovskaya et al., 2017). Despite an enormous amount of pre-clinical research (Habtezion et al., 2019; Lee and Papachristou, 2019; Saluja et al., 2019) and clinical trials (Moggia et al., 2017), no effective targeted pharmacological treatment for AP has been discovered. Therefore, the current treatment of AP is limited to supportive care as well as the management of local and systemic complications (Vege et al., 2018).
AP, chronic pancreatitis, and pancreatic cancer are the common diseases of the exocrine pancreas (Petrov and Yadav, 2019). About 10% of AP patients will develop chronic pancreatitis (Sankaran et al., 2015) and its global incidence is 10 cases per 100,000 general population per year (Xiao et al., 2016). The estimates of incidence and mortality for pancreatic cancer are 8.14 cases and 6.92 deaths per 100,000 persons annually, respectively (Xiao et al., 2016). In China, pancreatic ductal adenocarcinoma (PDAC) is expected to be the second leading cause of cancer-related death by 2030 (Siegel et al., 2019).
Emerging evidence suggests that inflammatory cytokines including MIF, TNF-α, interferon gamma, and transforming growth factor beta are increased in the setting of cancer (Lippitz, 2013). An elevated cytokine concentration profile is associated with reduced survival in pancreatic cancer patients (Babic et al., 2018). There remains no effective pharmacological treatment for PDAC and the prognosis is still extremely poor (Lippitz, 2013; Ryan et al., 2014). There appears to be a compelling role for MIF in pancreatic diseases. Circulating MIF levels are significantly higher in obese or type 2 diabetic populations compared to healthy controls (Morrison and Kleemann, 2015), are significantly elevated in experimental and human AP and correlated with disease severity (Sakai et al., 2003), and are also highly up-regulated in exosomes (Costa-Silva et al., 2015) and in PDAC tissue (Funamizu et al., 2013; Lippitz, 2013; Tan et al., 2014). Small molecular MIF inhibitors, anti-MIF antibodies, and genetic ablation of MIF have all been tested and show protective effects in experimental AP (Sakai et al., 2003; Matsuda et al., 2006; Guo et al., 2018; Li et al., 2019; Zhu et al., 2020) and PDAC (Winner et al., 2007; Denz et al., 2010; Funamizu et al., 2013; Tan et al., 2014; Costa-Silva et al., 2015; Guo et al., 2016; Yang et al., 2016; Wang et al., 2018; Suresh et al., 2019) models.
The aim of this review is to describe what is known about the structure and function of MIF with a particular focus on signaling pathways involved in inflammation and cancer. The role of MIF in AP and PDAC and the potential for MIF targeted treatment strategies are also emphasised.
Literature Search
A systematic literature search was conducted in Ovid Medline (PubMed), Scopus, Science Citation Index expanded, and Google Scholar to find related articles. The key words were “acute pancreatitis,” “pancreatitis,” “chronic pancreatitis,” or “pancreatic cancer” in combination with “macrophage migration inhibitory factor” or “D-Dopachrome tautomerase.” All studies investigating MIF in experimental and clinical exocrine pancreatic diseases were collated. Reference lists of relevant reviews and other non-primary data sources regarding this context captured by the search strategy were also manually screened. Only publications in English were included. In total, 15 studies investigating MIF in AP and 14 studies in PDAC were summarized in this review. We did not identify original study investigating MIF and chronic pancreatitis.
Structure and Function of MIF
MIF is a highly conserved protein of 12.5 kDa, with evolutionarily ancient homologues in plants, protozoans, nematodes, and invertebrates (Sparkes et al., 2017). The MIF protein is a 115-amino acid polypeptide that folds to form two antiparallel α-helices that pack against a 4-stranded β-sheet (Trivedi-Parmar and Jorgensen, 2018). On the basis of X-ray crystallography data, the biologically active form of MIF is a homotrimer (Suzuki et al., 1996). MIF is different from other cytokines because its structure contains three evolutionarily stable catalytic sites that are associated with tautomerase and oxidoreductase activities (Rosengren et al., 1996; Sun et al., 1996; Suzuki et al., 1996).
MIF is secreted by the anterior pituitary and immune cells (Calandra et al., 1994) and is ubiquitously stored and expressed in a variety of cells including epithelial, endothelial, mesenchymal, dendritic, and other cell types (Jankauskas et al., 2019). Constitutive release of MIF from cells results in its high concentration in the extracellular space (Lee et al., 2016; Lang et al., 2018). However, the process by which MIF is released is not fully understood. Unlike other cytokines, MIF exists as a pre-formed type in multiple cell sub-populations throughout the body (Calandra et al., 1994; Bacher et al., 1997), and is particularly distributed and expressed in cells of the nervous (Nishibori et al., 1996) and endocrine systems (Calandra and Roger, 2003) that have direct contact with the natural environment, (e.g. lung, skin, and gastrointestinal). MIF production is largely facilitated in response to an array of stimuli including hypoxia, hydrogen peroxide, lipopolysaccharide (LPS), TNF-α, thrombin, and angiotensin II (Jankauskas et al., 2019). Waeber et al. (Waeber et al., 1997) reported that MIF was highly expressed in several insulin-secreting cell lines, colocalized with insulin-containing secretory granules, and was secreted in response to glucose stimulation in a time- and concentration-dependent manner. Immunoneutralization of MIF by anti-MIF IgG reduced the first and second phase of the glucose-induced insulin secretion response by 39 and 31%, respectively. Whether pancreatic acinar cells produce and release MIF remains to be determined.
Pathophysiologic Role of MIF
MIF circulates normally at levels from 2 ng/ml to 6 ng/ml, following a circadian rhythm that correlates with plasma cortisol under physiological conditions (Petrovsky et al., 2003). This is of particular clinical relevance as low concentration of glucocorticoids induce release of MIF into circulation and in turn, circulating MIF overrides glucocorticoid-mediated inhibition of cytokine secretion, and has been shown to fully abolish the protective effect of glucocorticoids in a lethal model of endotoxin-induced inflammation (Calandra et al., 1995; Bloom et al., 2016a). In the case of acute inflammatory diseases, MIF has been demonstrated to be implicated in the pathogenesis of glomerulonephritis, acute lung injury, sepsis, and AP, and its elevation is closely associated with disease severity or progression (Harris et al., 2019). In addition to its cytokine activity, mammalian MIF also harbors diverse catalytic functions. In this regard, tautomerase activity is the most widely studied function, exhibiting the ability to catalyze tautomerization of phenylpyruvate, p-hydroxyphenylpyruvate, and D-dopachrome (Rosengren et al., 1996; Rosengren et al., 1997). Moreover, MIF interferes in cell cycle regulation by negatively interacting with c-Jun activation binding protein-1 (JAB1, also referred to the fifth component of the constitutive photomorphogenic-9 signalosome, CSN5)-dependent pathways, resulting in degradation of cyclin-dependent kinase inhibitor p27Kip1 and cell cycle progression (Kleemann et al., 2000).
D-Dopachrome Tautomerase
D-dopachrome tautomerase (D-DT, also referred to MIF-2) comprises 117 amino acids with a molecular weight of 13 kDa (Zhang et al., 1995; Nishihira et al., 1998). It has a highly homologous tertiary structure and similar biological properties to MIF (Sugimoto et al., 1999; Merk et al., 2011). Whereas plasma D-DT and MIF circulate in similar concentrations under basal or pathological conditions, LPS-treated macrophages release 20-fold more MIF than D-DT, indicating D-DT derived from nonmacrophage sources prominently contribute to plasma D-DT expressions in vivo (Merk et al., 2011). The differences and coincidences between D-DT and MIF have been reviewed by Illescas et al. (Illescas et al., 2020). Regardless of the similarities, D-DT seems to play different or even opposed role from MIF under some circumstances. For example, D-DT lacks both the CXXC redox motif and pseudo (E)LR motifs present on MIF, while the former one is important in sensing redox signals and the latter one is essential for its chemokine function (Esumi et al., 1998; Bernhagen et al., 2007; Merk et al., 2011). In adipose tissues, D-DT and MIF are differentially expressed and have distinct roles in adipogenesis. While D-DT is negatively correlated with obesity and reverses glucose intolerance, MIF is positively correlated with obesity and insulin resistance (Kim et al., 2015). D-DT also binds to JAB1 and the interaction affinity between JAB1 and D-DT is comparable to that observed between JAB1 and MIF (Merk et al., 2011).
MIF Signalling Pathways in Inflammation and Cancer
The MIF-related key signaling pathways in inflammation and cancer are delineated in Figure 1.
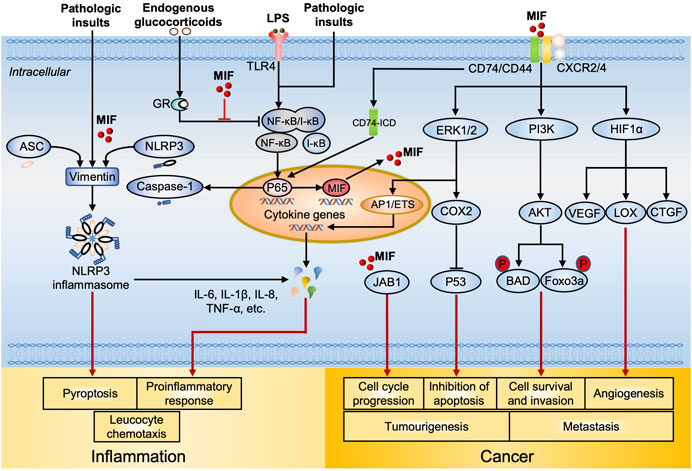
FIGURE 1. MIF-mediated signal transduction and regulation in inflammation and cancer. Intracellularly, MIF overrides glucocorticoids induced suppression of NF-ĸB synthesis and nuclear translocation, leading to increased cytokine production, (e.g. IL-6 and TNF-α). MIF also facilitates NLRP3 inflammasome assembly through interacting with ASC, vimentin and NLRP3, resulting in pyroptosis and elevated production of IL-1β and IL-18. MIF upregulates the expression of TLR4 by facilitating the transcription of NF-ĸB, which allows rapid recognition of LPS by TLR4, promoting the production of cytokines (including MIF). Intracellular MIF also binds to JAB1, resulting in tumor cell cycle progression. After MIF is released, extracellular MIF binds to the transmembrane receptor complex CD74 and CD44, resulting in the subsequent phosphorylation of ERK/MAPK and PI3K/AKT. ERK1/2 activates transcription elements AP1/ETS, which leads to expression of pro-inflammatory cytokines. On the other side, the activation of ERK/COX2 inhibits p53-dependent apoptosis, promoting tumor cell proliferation. PI3K/AKT activation phosphorylated Bcl-2 family member protein BAD and Foxo transcription factor Foxo3a, enhancing cancer cell survival and invasion. During hypoxia, the binding of MIF to CD74 contributes to HIF1α activation and stabilization, which then upregulates the expression of angiogenic growth factors including VEGF, LOX, and CTGF that consequently promote angiogenesis. Meanwhile, CD74/CD44 receptor complex releases CD74 intracellular domain (CD74-ICD), it translocates into the nucleus and increases NF-ĸB activation, leading to increased caspase-1 activation and NLRP3 production. Extracellular MIF also binds to G-protein-coupled chemokine receptors CXCR2, CXCR4, and CXCR7 individually or dependent on CD74 to form MIF/CXCR2 and MIF/CXCR4 complex, activating leucocyte chemotaxis. Abbreviations: NF-ĸB, nuclear factor kappa B; IL, interleukin; TNF, tumor necrosis factor; NLRP3, NLR Family Pyrin Domain Containing three; TLR4, toll-like receptor four; LPS, lipopolysaccharide; CD74, Cluster of Differentiation 74; ERK, extracellular signal-regulated kinase; MAPK, mitogen-activated protein kinase; PI3K, phosphoinositide 3-kinase; AP1, activator protein 1; COX2, cyclooxygenase-2; BAD, Bcl-2 agonist of cell death; FoxO3a, forkhead box O3a; HIF1α, hypoxia inducible factor 1 alpha; VEGF, vascular endothelial growth factor; LOX, lysyl oxidase; CTGF, connective tissue growth factor; CXCR, C-X-C chemokine receptor.
MIF Receptor-Mediated Signaling Pathways
When extracellular MIF binds to its primary receptor cluster of differentiation 74 (CD74) (Leng et al., 2003) on the cell membrane, co-receptors including CD44 or C-X-C chemokine receptors (CXCRs; CXCR2, CXCR4, and CXCR7) (Bernhagen et al., 2007; Alampour-Rajabi et al., 2015) are also required to activate downstream signaling pathways (Shi et al., 2006). Once the CD74/CD44 complex is activated by MIF through the proto-oncogene tyrosine-protein kinase (SRC) (Leng et al., 2003), mitogen-activated protein kinase (MAPK) family members such as the extracellular signal-related kinase 1/2 (ERK1/2), phosphoinositide 3-kinase (PI3K), and protein kinase B (PKB, also known as AKT) are phosphorylated and subsequently activated (Leng et al., 2003; Shi et al., 2006; Lue et al., 2007; Gore et al., 2008). Sustained ERK1/2 activation promotes cancer cell invasion and inhibits cell death (Mitchell et al., 1999). AKT activation leads to phosphorylation of the proapoptotic proteins including Bcl-2 agonist of cell death (BAD) and transcription factor forkhead box O-3a (FoxO3a), promoting cancer cell survival (Lue et al., 2007). MIF-induced cyclo-oxygenase-2 (COX-2)/prostaglandin E2 (PGE-2) activation enhances tumor growth, cancer cell viability, and metastasis. MIF downregulates tumor suppressor protein p53, leading to inhibition of p53-dependent apoptosis, accumulation of mutation, and proliferation of cancer cells (Hudson et al., 1999; Mitchell et al., 2002). ERK1/2 and PI3K/AKT also activate transcription factors including nuclear factor-kappa B (NF-κB) and activator protein-1 (AP1), which result in the release of pro-inflammatory cytokines such as IL-6, IL-8, IL-10, and TNF-α. IL-6 and IL-8 also exhibit pro-tumourigenic functions including promotion of tumor formation by enhancing proliferation, reducing apoptosis, and promoting invasiveness (Taniguchi and Karin, 2014; Johnson et al., 2018). Besides, MIF inhibits p53-mediated apoptosis in macrophage with the induction of increased cytoplasmic phospholipase A2 (PLA2), arachidonic acid, COX2 and PGE2, which sustains the macrophage pro-inflammatory function (Mitchell. et al., 1999; Mitchell et al., 2002). MIF production is upregulated in hypoxic conditions associated with tumor development and progression (Winner et al., 2007). During hypoxia, MIF binding to CD74 contributes to hypoxia inducible factor 1 alpha (HIF1α) activation and stabilization, which then upregulates the expression of angiogenic growth factors including vascular endothelial growth factor (VEGF), lysyl oxidase (LOX), and connective tissue growth factor (CTGF) (Coleman et al., 2008; Oda et al., 2008; Xu et al., 2008; O’reilly et al., 2016). Therefore, MIF supports tumor growth through significantly enhancing angiogenesis. These pathways provide mechanistic bases to explain the role of MIF in the action of pro-inflammatory effects and cancer progression.
MIF can individually bind to CD74 or CXCR2/4 independent of whether the other receptor type is co-expressed (Bernhagen et al., 2007; Schwartz et al., 2009). Extracellular MIF triggers mononuclear (monocytes, neutrophils, and T cells) cell chemotaxis via MIF/CXCR2 and MIF/CXCR4. Monocyte arrest elicited by MIF depends on CD74 and the complex formed by MIF/CXCR2 or MIF/CXCR4 in the context of inflammation and atherosclerosis (Bernhagen et al., 2007). This effect can be suppressed by either a MIF genetic deficiency or antibodies to MIF, CXCR2 or CD74 (Bernhagen et al., 2007). Moreover, MIF can directly interact with CXCR7 (Alampour-Rajabi et al., 2015), resulting in MIF/CXCR7-mediated functional responses that include promotion of human CXCR7 internalization, activation of MIF-mediated ERK1/2 zeta-chain associated protein kinase-70 signaling pathway as well as B cell chemotaxis. It is not completely elucidated whether CD44 is also involved in the receptor complexes of CD74 with the CXCRs. Therefore, tissue responsiveness to MIF depends on its specific expression of MIF receptors and co-receptors, (i.e. CD44 and CXCRs).
Intracellular MIF-Mediated Signaling Pathways
In addition to its extracellular activities, it is reported that endocytic MIF can transcriptionally and post-transcriptionally override the immunosuppressive effects of glucocorticoids (Calandra and Roger, 2003). Other studies (Roger et al., 2001; Galvão et al., 2016; Lang et al., 2018; Shin. et al., 2019) published in the past few years collectively support a regulatory effect of endogenous MIF on Toll-like receptor 4 (TLR4) and the NLR family pyrin domain containing 3 (NLRP3) inflammasome signaling pathways. These are thought to play a critical role in AP for developing further pancreatic injury and systemic inflammation (Sharif et al., 2009; Sendler et al., 2020). In response to the stimulation by LPS and Gram-negative bacteria (canonical TLR4 activators), the MIF-deficient macrophages were found to be hyposensitive (reduced production of pro-inflammatory cytokines, e.g., TNF-α and IL-6) (Roger. et al., 2001), highlighting a role for MIF in modulation of TLR4 downstream signaling pathways.
MIF as a regulator of the NLRP3-mediated inflammatory response has been described in recent studies (Lang et al., 2018; Shin. et al., 2019). One study showed that MIF colocalized with ASC (apoptosis-associated speck-like protein containing a CARD), vimentin and NLRP3, potentially modulating the interaction between NLRP3 and vimentin to facilitate the NLRP3 inflammasome assembly, leading to acceleration of downstream cytokine release and pyroptosis (Lang et al., 2018). Accordingly, depletion or inhibition of MIF in macrophages and dendritic cells resulted in the inhibition of IL-1α, IL-1β, and IL-18 release in response to NLRP3-activating stimuli. Another study illustrated the link between MIF and NLRP3 activation in human peripheral blood monocytes using U1 small nuclear ribonucleoprotein immune complex, a NLRP3 inflammasome activator (Shin et al., 2012; Shin. et al., 2019), which can stimulate MIF and IL-1β production in human monocytes. MIF levels were increased in synovial fluid and positively associated with IL-1β in a murine acute gout model and human patients (Galvão et al., 2016). Taken together, these studies highlight the role of MIF in modulating activation of downstream events through TLR4/NLRP3 signaling pathways.
Moreover, intracellular MIF binds to JAB1 and results in tumor cell cycle progression and proliferation. MIF-JAB1 interaction also stabilize HIF1α by preventing its hydroxylation (Winner et al., 2007), resulting in increased expression of pro-angiogenic factors such as VEGF and IL-8 (Oda et al., 2008). It subsequently promotes tumor angiogenesis.
MIF and Exocrine Pancreatic Diseases
MIF and Experimental AP
Results from studies investigating MIF and experimental AP are summarized in Table 1. Investigation of MIF in AP began in 2003, when Sakai and colleagues demonstrated a functional role for MIF in experimental models of AP induced by taurocholic acid (TCA-AP), caerulein (CER-AP), and choline-deficient, ethionine-supplemented diet (CDE-AP) (Sakai et al., 2003). In isolated peritoneal macrophages from ascitic fluid of TCA-AP rats, co-incubation with anti-MIF antibodies significantly inhibited IL-8 production (Sakai et al., 2003). MIF levels of serum, ascitic fluid, and lung tissue, but not pancreas or liver, were significantly increased in the TCA-AP in rats. Similarly, MIF levels in the lung were also significantly increased in the CDE-AP in mice. As for CER-AP in rats, the MIF levels were only markedly elevated in pancreatic ascites and thus peritoneal macrophages were considered to be the cellular sources of ascitic MIF. In 2006, Matsuda et al. (Matsuda et al., 2006) reported that plasma and lung MIF levels were increased by 7-fold and 4.7-fold, respectively, in a severe AP model induced by three injections of caerulein and a low dose of LPS in mice (CER/LPS-AP). The MIF levels in pancreatic tissue and serum were increased in l-arginine-induced AP (ARG-AP) in mice (Ohkawara et al., 2017) and their expression was up-regulated in the intrahepatic bile duct cells in a sodium taurocholate-induced AP (STC-AP) in rats (Wang et al., 2019).
Compared with wild type littermates, Mif−/− mice reduced pancreatic and serum pro-inflammatory indices as well as severity in ARG-AP (Zhu et al., 2020) and acute lung injury in CER/LPS-AP (Matsuda et al., 2006). Pre-treatment with anti-MIF antibody decreased lung TNF-α levels in the TCA-AP (Sakai et al., 2003) and suppressed the AP-induced elevation of lung TLR4 expression in CER/LPS-AP (Matsuda et al., 2006). Furthermore, anti-MIF antibody significantly reduced lethality in TCA-AP (Sakai et al., 2003), CDE-AP (Sakai et al., 2003), and CER/LPS-AP (Matsuda et al., 2006). MIF specific inhibitor ISO-1 ((S, R)3-(4-hydroxyphenyl)-4, 5-dihydro-5-isoxazole acetic acid methyl ester) significantly mitigated pancreatic (Zhou et al., 2018), lung (Zhou et al., 2018), liver (Guo et al., 2018), and kidney (Li et al., 2019) injury with simultaneous reduction of MIF and a spectrum of pro-inflammatory mediators in STC-AP in pregnant rats. In addition, administration of anti-protease-activated receptor-2 antibody (CER/LPS-AP) (Matsuda et al., 2006), glucocorticoid agonist (ARG-AP) (Paszt et al., 2008), chlorogenic acid (ARG-AP) (Ohkawara et al., 2017), and ginkgo biloba extract (STC-AP) (Xu et al., 2014) also reduced the severity of AP and circulating, pancreatic, or alveolar macrophage MIF levels in experimental models.
MIF and Human AP
Clinical studies of MIF and AP are displayed in Table 2. Clinical studies (Sakai et al., 2003; Makhija et al., 2007; Rahman et al., 2007; Dambrauskas et al., 2010; Deng et al., 2017) that measured circulating MIF levels collectively and consistently demonstrated that the admission circulating MIF levels were proportionally associated with severity of AP patients. Sakai et al. (Sakai et al., 2003) determined serum MIF levels of 28 patients with mild AP and 18 with severe AP within 72 h disease onset in parallel with 12 healthy individuals, revealing that severe AP were associated with markedly higher serum MIF levels compared to mild AP and healthy controls (both p < 0.01). But there was no statistical difference between mild AP and controls. Similar results were observed in the study conducted by Rahman et al. (Rahman et al., 2007), although the serum samples were collected earlier at 24 h after AP onset. In addition, serum MIF levels were significantly raised in patients who developed pancreatic necrosis or multiple organ failure, indicating MIF could act as a sensitive biomarker to predict local and systemic complications for AP. Another study (Dambrauskas et al., 2010) comprising 108 AP patients (60 mild, 48 severe) also found that serum MIF levels within 72 h disease onset could be used as discriminator of severe and necrotizing AP. A study performed by our group (Deng et al., 2017) identified MIF as an early predictor for discriminating AP patients who had persistent organ failure (n = 20) from those without (n = 50) and healthy controls (n = 10) with an area under the receiver operating characteristic curve (AUC) of 0.90 (95% confidence interval [CI], 0.81–0.96). A most recent study (Shen et al., 2021) demonstrated that MIF at a cut-off value of 2.3 ng/ml has the best discriminative power (AUC, 0.950; 95% CI, 0.914–0.987) for predicting severe AP which was higher than Acute Physiology and Chronic Health Evaluation II (AUC, 0.899), Bedside Index for Severity in Acute Pancreatitis (AUC, 0.886), and serum IL-6 (AUC, 0.826). Therefore, MIF could be used as a potential early severity predictor in patients with AP and this needs further validation.
Previous studies described the ‘G’ to ‘C’ single nucleotide polymorphism (at −173 position) of MIF in patients with systemic-onset juvenile idiopathic arthritis (Donn et al., 2001) and sarcoidosis (Amoli et al., 2002), and a CATT repeat microsatellite (at -794 position) to be associated with lower disease severity in rheumatoid arthritis (Baugh et al., 2002). Accordingly, Makhija et al. (Makhija et al., 2007) compared the MIF gene polymorphism of a United Kingdom cohort of 164 AP patients with 197 healthy controls. It is shown that the distribution of MIF-173 genotype was significantly different between the two groups (p = 0.046), whilst there was no difference regarding the distribution of MIF-794 microsatellite genotypes and alleles. However, these findings warrant confirmation from a larger population.
MIF and Experimental Pancreatic Cancer
The in vitro and in vivo studies of MIF and pancreatic cancer are outlined in Tables 3, 4. The levels of MIF expression vary in pancreatic cancer cell lines with high over-expression in PT-45, CFPAC-1, PaCa2, and Capan-1 cells (Ct < 6.5) (Denz et al., 2010). MIF over-expression is associated with reduced E-cadherin expression and increased vimentin expression, indicative of epithelial-to-mesenchymal transition characteristics thus enhanced invasiveness in pancreatic cancer cell lines (Funamizu et al., 2013). Besides, MIF over-expression is also associated with increased proliferation and reduced sensitivity to gemcitabine (Funamizu et al., 2013; Yang et al., 2016). Activation of PI3K/Akt (Denz et al., 2010; Guo et al., 2016; Yang et al., 2016; Wang et al., 2018) and ERK (Guo et al., 2016; Wang et al., 2018) mediated signaling pathways were demonstrated to be involved in the process. MIF knockdown by siRNA attenuated proliferation and invasion along with increased apoptosis in pancreatic cancer cell lines via upregulation of p53 and downregulation of ERK1/2 and AKT phosphorylation (Denz et al., 2010; Guo et al., 2016; Wang et al., 2018). MIF inhibitor (4-iodo-6-phenylpyrimidine; 4-IPP) reduced proliferation and colony formation in PANC-1 cells (Guo et al., 2016).
A recent study (Suresh et al., 2019) found that recombinant MIF treatment significantly enhanced tumor growth via promoting angiogenesis in a hamster pancreatic cancer model induced by subcutaneous inoculation with HapT1 cells. Conversely, MIF knockdown or inhibition (ISO-1) reduced overall cell growth in HapT1 cells.
Intriguingly, MIF has been linked to exosomes in PDAC. Costa-Silva et al. (Costa-Silva et al., 2015) demonstrated that MIF levels in plasma exosomes isolated from a mouse pancreatic cancer model were markedly increased compared to non-cancerous controls, even at an early stage of pancreatic intraepithelial neoplasia. The process of MIF-expressing PAN02 exosome “education”, (whereby naive, wild-type mice were injected retro-orbitally every other day for three weeks with 5 μg of PAN02-derived exosomes), induced liver pre-metastatic niche formation in treated mice which was also inhibited by MIF knockdown.
Yang et al. (Yang et al., 2016) demonstrated the role of MIF–miR-301b–NR3C2 axis in the pathogenesis of PDAC. Over-expression of MIF induced a marked increase of miR-301b and reduction of NR3C2 levels, resulting in profound proliferation, migration, and invasion of pancreatic cancer cell lines (PANC-1 and Capan-2). While inhibition of miR-301b abolished MIF-induced suppression of NR3C2 in vitro, Mif−/− mice were associated with reduced metastasis and improved survival in a pancreatic cancer mouse model (LSL-KrasG12D, LSL-Trp53R172H/+, Pdx-1-Cre) in vivo.
MIF and Human Pancreatic Cancer
Clinical studies of MIF and pancreatic cancer are presented in Table 5. Collectively, these clinical studies have determined the circulating MIF levels (Winner et al., 2007; Fredriksson et al., 2008; Chen et al., 2010; Kondo et al., 2013; Tan et al., 2014; Schinagl et al., 2016) and MIF expression in dissected pancreatic tissue (Cui et al., 2009; Denz et al., 2010; Funamizu et al., 2013; Tan et al., 2014; Guo et al., 2016; Schinagl et al., 2016; Yang et al., 2016; Wang et al., 2018) of PDAC patients and healthy controls. All the studies reported that MIF levels in serum/plasma or pancreatic tissue were significantly higher in PDAC patients than healthy controls or paired non-cancerous tissue with preferable diagnostic utility, except for one study (Fredriksson et al., 2008) in which there was 30% increase in PDAC without significant difference compared with healthy controls (p = 0.15). Higher MIF expression in tumor tissue was associated with worse survival of PDAC patients (Funamizu et al., 2013; Wang et al., 2018).
In addition, Denz et al. (Denz et al., 2010) reported that MIF mRNA expression in pancreatic tissue was higher in PDAC than chronic pancreatitis, both were higher than normal controls. Chen et al. (Chen et al., 2010) compared the serum MIF in PDAC patients and controls including AP, chronic pancreatitis and healthy donors, showing that MIF had an area under the curve of receiver operating characteristic of 0.78 in discriminating pancreatic cancer from controls. Tan et al. (Tan et al., 2014) focused on diabetes mellitus-associated pancreatic cancer (DM-PC) and found that MIF expression in pancreatic tissues of DM-PC was markedly higher when comparing to chronic pancreatitis or pancreatic cancer without DM. Similarly, serum MIF levels were also higher in new-onset than long term DM-PC, pancreatic cancer without DM, or new-onset T2DM patients (all p < 0.001).
MIF Targeted Treatment Strategies
Different MIF antagonism strategies are depicted in Figure 2.
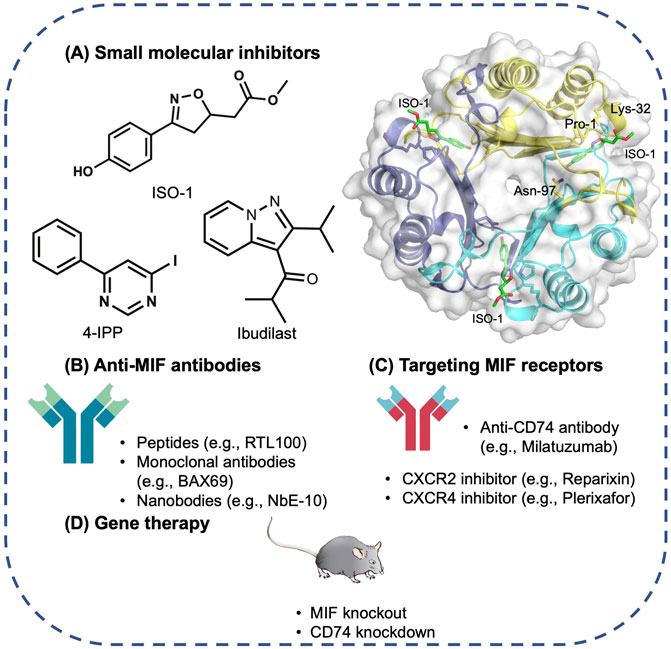
FIGURE 2. Therapeutic strategies targeting MIF. (A) Compounds bind to MIF’s tautomerase active site. For example, ISO-1 binds to the active-site residue Asn-97 of MIF (PDB 1LJT), leading to structural changes that block MIF–CD74 binding; 4-IPP covalently binds to Pro-1 of MIF or DDT, altering their structure and preventing function; and the non-competitive allosteric tautomerase inhibitor Ibudilast. (B) Anti-MIF antibodies. (C) Drugs targeting MIF receptors. (D) Gene therapy. Abbreviations: ISO-1, (S, R)3-(4-hydroxyphenyl)-4, 5-dihydro-5-isoxazole acetic acid methyl ester; CD74, Cluster of Differentiation 74; 4-IPP, 4-iodo-6-phenylpyrimidine; DDT, D-dopachrome tautomerase.
Small Molecular Inhibitors
Pharmacological inhibition of MIF with small molecule inhibitors have shown promise in the suppression of inflammation in various animals models such as severe sepsis (Calandra et al., 2000), rheumatoid arthritis (Mikulowska et al., 1997), allergic airway inflammation (Amano et al., 2007), colitis (Ohkawara et al., 2002), glomerulonephritis (Brown et al., 2002), and chronic obstructive pulmonary disease (Russell et al., 2016). Development of MIF inhibitors has been comprehensively and elegantly reviewed elsewhere (Xu et al., 2013; Kok et al., 2018; Trivedi-Parmar and Jorgensen, 2018). Small inhibitory agents of MIF have also been widely demonstrated in experimental cancer models, including lung cancer (Mawhinney et al., 2015), bladder cancer (Choudhary et al., 2013), adenoid cystic carcinoma (Liu et al., 2013), melanoma, and colon cancer (Ioanou et al., 2014). Briefly, small-molecule inhibitors of MIF primarily focus on rational structure-based design that target the MIF tautomerase activity and MIF-CD74 binding (Brown et al., 2009; Xu et al., 2013; Dickerhof et al., 2014; Spencer et al., 2015; Trivedi-Parmar and Jorgensen, 2018). The drought of a reliable, consensus-based in vitro assays for MIF biological activity has been a significant challenge to the development of small molecule MIF inhibitors. Further work should focus on developing a robust high-throughput clinically relevant MIF bioassay that can be applied for second-pass screening, glucocorticoid override, cellular proliferation, and cytokine release detection to expedite the discovery of efficient small molecular MIF inhibitors (Bloom et al., 2016b). In AP and PDAC, only ISO-1 and 4-IPP have been investigated in experimental animal models so far with limited data available, more endeavors are required to test small molecular MIF inhibitors in AP and PDAC in vitro and in vivo thus to consolidate the evidence for clinical translation.
Anti-MIF Antibodies
In AP, two studies (Sakai et al., 2003; Matsuda et al., 2006) have demonstrated the efficacy of anti-MIF antibody in CER/LPS and CDE diet AP models, respectively, revealing a promising therapeutic target. A newly established series of nanobodies (NbE5 and NbE10) have shown to attenuate lethality in in vivo septic shock model (Sparkes et al., 2018), is of great interest to be applied in other conditions of inflammatory end-organ damage, such as severe AP. A number of MIF monoclonal antibodies developed by researchers at Baxter have revealed significant anti-MIF activity in human PC3 prostate cancer cell lines and in vivo xenograft model (Hussain et al., 2013). In vitro, BaxG03, BaxB01, and BaxM159 reduced cell growth and viability by inhibiting ERK1/2 and AKT pathways. The antibodies also inhibited MIF-promoted migration and invasion. In vivo, treatment with anti-MIF antibodies reduced tumor growth in a dose-dependent manner. Recently, a phase 1 clinical trial using imalumab (Bax69) in solid tumors and metastatic colorectal adenocarcinoma demonstrated that imalumab has a maximum tolerated dose of 37.5 mg/kg every 2 weeks and a biologically active dose of 10 mg/kg weekly (Mahalingam et al., 2020). Further investigation is warranted to define the role of anti-MIF antibody as a treatment strategy for pancreatic cancer. Of note, the development of antibodies may be mitigated by their short half-life, high costs associated with production, and potential immunogenicity.
Targeting MIF Receptors
As MIF relies largely on CD74 to regulate the downstream cellular events, treatment targeting CD74 holds great potential to inhibit MIF signaling. A humanized anti-CD74 monoclonal antibody, milatuzumab has shown to significantly prolong the survival duration of multiple myeloma xenograft mice models (Stein et al., 2004; Stein et al., 2009). It has reached phase 1 clinical trial in multiple myeloma (Kaufman et al., 2013) and systemic lupus erythematosus (Wallace et al., 2016), indicating no severe adverse effects. Apart from CD74, inhibitors against CXCL2 (Reparixin) and CXCL4 (Plerixafor) are also of interest for further investigation (Steinberg and Silva, 2010; Goldstein et al., 2020). However, as MIF/CD74 pathway also plays an important role in wound repair by activating pro-survival and proliferative pathways that protects the host during injury (Farr et al., 2020), complete inhibition of CD74 could cause some unpredictable side effects which need precaution.
MIF-Related Gene Therapy
First of all, MIF knockout animals are long lived with no characteristic health issues, giving the opportunity for the initiation of MIF-related gene therapy (Harper et al., 2010). MIF related gene therapy such as Mif gene knockout/knockdown, DDT gene knockdown, or CD74 gene knockdown has exhibited great potential in pre-clinical studies of AP (Matsuda et al., 2006; Zhu et al., 2020) and PDAC (Winner et al., 2007; Denz et al., 2010; Funamizu et al., 2013; Tan et al., 2014; Costa-Silva et al., 2015; Guo et al., 2016; Yang et al., 2016; Wang et al., 2018; Suresh et al., 2019). Furthermore, it was demonstrated that a xenograft model of head and neck squamous cell carcinoma with MIF knockdown was more sensitive to cisplatin and 5-fluorouracil treatment than control (Kindt et al., 2013). On the other hand, overexpressing MIF in pancreatic cancer cells reduced the sensitivity to gemcitabine (Funamizu et al., 2013). Taken together, it supports that MIF downregulation may potentiate the effect of chemotherapy agents in cancer. There remain ongoing opportunities to develop additional MIF suppression therapies for clinical evaluation.
Conclusion
In summary, we have comprehensively reviewed the role of MIF in AP and PDAC. It is apparent from the review that investigations of MIF in AP are at a relatively early stage. Up to now, in vivo AP studies have measured circulating MIF levels, confirmed MIF expression in target organs, and commenced applying MIF inhibitory drugs for efficacy testing. Whether pancreatic acinar cells express MIF and how MIF contributes to the early acinar cell events in AP, (i.e. calcium overload, mitochondrial dysfunction, oxidative stress, endothelial reticulum stress, and trypsinogen activation) remains unclear. It is notable that anti-MIF antibodies and the MIF inhibitor ISO-1, have shown encouraging potential for improving pancreatic damage and associated organ injury in AP animal models. Clinical studies have identified circulating MIF as a potential biomarker for early prediction of AP severity which needs further validation. Future research is warranted to detail the underlying molecular mechanisms, (i.e. TLR4/NLRP3) of MIF in pancreatic acinar cells and AP. In chronic pancreatitis, the role of MIF remains elusive. In pancreatic cancer, MIF enhances the proliferation and invasion of tumor cells, resulting in increased tumor growth and metastasis in vivo. Early studies of MIF knockdown or use of specific inhibitors support MIF as a potential target for PDAC. Future research is required to bring forward a range of promising treatment approaches to clinical evaluation.
Author Contributions
TL, DD, and WH conceived the concept and designed the study. YW and WC collected literatures. YW, WC, JY, and LP analyzed literatures and summarized results. YW and WC drafted the manuscript. XF, JW, QX, AP, JT, and WH revised the manuscript. All authors contributed to the writing and final approval of the manuscript.
Funding
National Science Foundation of China (No. 81800575, TL; No. 81973632, WH; No. 81774120, QX); China-New Zealand Strategic Research Alliance Award (No. 2016YFE0101800, WH, QX); China-New Zealand International Cooperation Programme from Sichuan Provincial Department of Science and Technology (2019YFH0157, DD); China-New Zealand Scientist Exchange Programme (2018, WH; 2019, DD); The Ministry of Science and Technology of China (No. 2018ZX09201018-005, XF).
Conflict of Interest
The authors declare that the research was conducted in the absence of any commercial or financial relationships that could be construed as a potential conflict of interest.
References
Alampour-Rajabi, S., El Bounkari, O., Rot, A., Müller-Newen, G., Bachelerie, F., Gawaz, M., et al. (2015). MIF interacts with CXCR7 to promote receptor internalization, ERK1/2 and ZAP-70 signaling, and lymphocyte chemotaxis. Faseb j 29, 4497–4511. doi:10.1096/fj.15-273904 |
Amano, T., Nishihira, J., and Miki, I. (2007). Blockade of macrophage migration inhibitory factor (MIF) prevents the antigen-induced response in a murine model of allergic airway inflammation. Inflamm. Res. 56, 24–31. doi:10.1007/s00011-007-5184-9 |
Amoli, M. M., Donn, R. P., Thomson, W., Hajeer, A. H., Garcia-Porrua, C., Lueiro, M., et al. (2002). Macrophage migration inhibitory factor gene polymorphism is associated with sarcoidosis in biopsy proven erythema nodosum. J. Rheumatol. 29, 1671–1673.
Babic, A., Schnure, N., Neupane, N. P., Zaman, M. M., Rifai, N., Welch, M. W., et al. (2018). Plasma inflammatory cytokines and survival of pancreatic cancer patients. Clin. Transl Gastroenterol. 9, 145. doi:10.1038/s41424-018-0008-5 |
Bacher, M., Meinhardt, A., Lan, H. Y., Mu, W., Metz, C. N., Chesney, J. A., et al. (1997). Migration inhibitory factor expression in experimentally induced endotoxemia. Am. J. Pathol. 150, 235–246.
Baugh, J. A., Chitnis, S., Donnelly, S. C., Monteiro, J., Lin, X., Plant, B. J., et al. (2002). A functional promoter polymorphism in the macrophage migration inhibitory factor (MIF) gene associated with disease severity in rheumatoid arthritis. Genes Immun. 3, 170–176. doi:10.1038/sj.gene.6363867 |
Bernhagen, J., Calandra, T., Mitchell, R. A., Martin, S. B., Tracey, K. J., Voelter, W., et al. (1993). MIF is a pituitary-derived cytokine that potentiates lethal endotoxaemia. Nature 365, 756–759. doi:10.1038/365756a0 |
Bernhagen, J., Krohn, R., Lue, H., Gregory, J. L., Zernecke, A., Koenen, R. R., et al. (2007). MIF is a noncognate ligand of CXC chemokine receptors in inflammatory and atherogenic cell recruitment. Nat. Med. 13, 587–596. doi:10.1038/nm1567 |
Bernhagen, J., Mitchell, R. A., Calandra, T., Voelter, W., Cerami, A., and Bucala, R. (1994). Purification, bioactivity, and secondary structure analysis of mouse and human macrophage migration inhibitory factor (MIF). Biochemistry 33, 14144–14155. doi:10.1021/bi00251a025 |
Bloom, B. R., and Bennett, B. (1966). Mechanism of a reaction in vitro associated with delayed-type hypersensitivity. Science 153, 80–82. doi:10.1126/science.153.3731.80 |
Bloom, J., Metz, C., Nalawade, S., Casabar, J., Cheng, K. F., He, M., et al. (2016a). Identification of iguratimod as an inhibitor of macrophage migration inhibitory factor (MIF) with steroid-sparing potential. J. Biol. Chem. 291, 26502–26514. doi:10.1074/jbc.M116.743328 |
Bloom, J., Sun, S., and Al-Abed, Y. (2016b). MIF, a controversial cytokine: a review of structural features, challenges, and opportunities for drug development. Expert Opin. Ther. Targets 20, 1463–1475. doi:10.1080/14728222.2016.1251582 |
Brown, F. G., Nikolic-Paterson, D. J., Hill, P. A., Isbel, N. M., Dowling, J., Metz, C. M., et al. (2002). Urine macrophage migration inhibitory factor reflects the severity of renal injury in human glomerulonephritis. J. Am. Soc. Nephrol. 13 (Suppl. 1), S7–S13.
Brown, K. K., Blaikie, F. H., Smith, R. A., Tyndall, J. D., Lue, H., Bernhagen, J., et al. (2009). Direct modification of the proinflammatory cytokine macrophage migration inhibitory factor by dietary isothiocyanates. J. Biol. Chem. 284, 32425–32433. doi:10.1074/jbc.M109.047092 |
Calandra, T., Bernhagen, J., Metz, C. N., Spiegel, L. A., Bacher, M., Donnelly, T., et al. (1995). MIF as a glucocorticoid-induced modulator of cytokine production. Nature 377, 68–71. doi:10.1038/377068a0 |
Calandra, T., Bernhagen, J., Mitchell, R. A., and Bucala, R. (1994). The macrophage is an important and previously unrecognized source of macrophage migration inhibitory factor. J. Exp. Med. 179, 1895–1902. doi:10.1084/jem.179.6.1895 |
Calandra, T., Echtenacher, B., Roy, D. L., Pugin, J., Metz, C. N., Hültner, L., et al. (2000). Protection from septic shock by neutralization of macrophage migration inhibitory factor. Nat. Med. 6, 164–170. doi:10.1038/72262 |
Calandra, T., and Roger, T. (2003). Macrophage migration inhibitory factor: a regulator of innate immunity. Nat. Rev. Immunol. 3, 791–800. doi:10.1038/nri1200 |
Chen, R., Crispin, D. A., Pan, S., Hawley, S., Mcintosh, M. W., May, D., et al. (2010). Pilot study of blood biomarker candidates for detection of pancreatic cancer. Pancreas 39, 981–988. doi:10.1097/MPA.0b013e3181dac920 |
Choudhary, S., Hegde, P., Pruitt, J. R., Sielecki, T. M., Choudhary, D., Scarpato, K., et al. (2013). Macrophage migratory inhibitory factor promotes bladder cancer progression via increasing proliferation and angiogenesis. Carcinogenesis 34, 2891–2899. doi:10.1093/carcin/bgt239 |
Coleman, A. M., Rendon, B. E., Zhao, M., Qian, M. W., Bucala, R., Xin, D., et al. (2008). Cooperative regulation of non-small cell lung carcinoma angiogenic potential by macrophage migration inhibitory factor and its homolog, D-dopachrome tautomerase. J. Immunol. 181, 2330–2337. doi:10.4049/jimmunol.181.4.2330 |
Costa-Silva, B., Aiello, N. M., Ocean, A. J., Singh, S., Zhang, H., Thakur, B. K., et al. (2015). Pancreatic cancer exosomes initiate pre-metastatic niche formation in the liver. Nat. Cel Biol 17, 816–826. doi:10.1038/ncb3169
Cui, Y., Zhang, D., Jia, Q., Li, T., Zhang, W., and Han, J. (2009). Proteomic and tissue array profiling identifies elevated hypoxia-regulated proteins in pancreatic ductal adenocarcinoma. Cancer Invest. 27, 747–755. doi:10.1080/07357900802672746 |
Dambrauskas, Z., Giese, N., Gulbinas, A., Giese, T., Berberat, P. O., Pundzius, J., et al. (2010). Different profiles of cytokine expression during mild and severe acute pancreatitis. World J. Gastroenterol. 16, 1845–1853. doi:10.3748/wjg.v16.i15.1845 |
David, J. R. (1966). Delayed hypersensitivity in vitro: its mediation by cell-free substances formed by lymphoid cell-antigen interaction. Proc. Natl. Acad. Sci. USA 56, 72–77. doi:10.1073/pnas.56.1.72 |
Deng, L. H., Hu, C., Cai, W. H., Chen, W. W., Zhang, X. X., Shi, N., et al. (2017). Plasma cytokines can help to identify the development of severe acute pancreatitis on admission. Medicine 96, e7312. doi:10.1097/MD.0000000000007312 |
Denz, A., Pilarsky, C., Muth, D., Rückert, F., Saeger, H. D., and Grützmann, R. (2010). Inhibition of MIF leads to cell cycle arrest and apoptosis in pancreatic cancer cells. J. Surg. Res. 160, 29–34. doi:10.1016/j.jss.2009.03.048 |
Dickerhof, N., Magon, N. J., Tyndall, J. D., Kettle, A. J., and Hampton, M. B. (2014). Potent inhibition of macrophage migration inhibitory factor (MIF) by myeloperoxidase-dependent oxidation of epicatechins. Biochem. J. 462, 303–314. doi:10.1042/BJ20140612 |
Donn, R. P., Shelley, E., Ollier, W. E., and Thomson, W. (2001). A novel 5’-flanking region polymorphism of macrophage migration inhibitory factor is associated with systemic-onset juvenile idiopathic arthritis. Arthritis Rheum. 44, 1782–1785. doi:10.1002/1529-0131(200108)44:8<1782::AID-ART314>3.0.CO;2-# |
Esumi, N., Budarf, M., Ciccarelli, L., Sellinger, B., Kozak, C. A., and Wistow, G. (1998). Conserved gene structure and genomic linkage for D-dopachrome tautomerase (DDT) and MIF. Mamm. Genome 9, 753–757. doi:10.1007/s003359900858 |
Farr, L., Ghosh, S., and Moonah, S. (2020). Role of MIF cytokine/CD74 receptor pathway in protecting against injury and promoting repair. Front. Immunol. 11, 1273. doi:10.3389/fimmu.2020.01273 |
Fredriksson, S., Horecka, J., Brustugun, O. T., Schlingemann, J., Koong, A. C., Tibshirani, R., et al. (2008). Multiplexed proximity ligation assays to profile putative plasma biomarkers relevant to pancreatic and ovarian cancer. Clin. Chem. 54, 582–589. doi:10.1373/clinchem.2007.093195 |
Funamizu, N., Hu, C., Lacy, C., Schetter, A., Zhang, G., He, P., et al. (2013). Macrophage migration inhibitory factor induces epithelial to mesenchymal transition, enhances tumor aggressiveness and predicts clinical outcome in resected pancreatic ductal adenocarcinoma. Int. J. Cancer 132, 785–794. doi:10.1002/ijc.27736 |
Galvão, I., Dias, A. C., Tavares, L. D., Rodrigues, I. P., Queiroz-Junior, C. M., Costa, V. V., et al. (2016). Macrophage migration inhibitory factor drives neutrophil accumulation by facilitating IL-1β production in a murine model of acute gout. J. Leukoc. Biol. 99, 1035–1043. doi:10.1189/jlb.3MA0915-418R |
Goldstein, L. J., Perez, R. P., Yardley, D., Han, L. K., Reuben, J. M., Gao, H., et al. (2020). A window-of-opportunity trial of the CXCR1/2 inhibitor reparixin in operable HER-2-negative breast cancer. Breast Cancer Res. 22, 4. doi:10.1186/s13058-019-1243-8 |
Gore, Y., Starlets, D., Maharshak, N., Becker-Herman, S., Kaneyuki, U., Leng, L., et al. (2008). Macrophage migration inhibitory factor induces B cell survival by activation of a CD74-CD44 receptor complex. J. Biol. Chem. 283, 2784–2792. doi:10.1074/jbc.M703265200 |
Greven, D., Leng, L., and Bucala, R. (2010). Autoimmune diseases: MIF as a therapeutic target. Expert Opin. Ther. Targets 14, 253–264. doi:10.1517/14728220903551304 |
Gukovskaya, A. S., Gukovsky, I., Algül, H., and Habtezion, A. (2017). Autophagy, inflammation, and immune dysfunction in the pathogenesis of pancreatitis. Gastroenterology 153, 1212–1226. doi:10.1053/j.gastro.2017.08.071 |
Guo, D., Guo, J., Yao, J., Jiang, K., Hu, J., Wang, B., et al. (2016). D-dopachrome tautomerase is over-expressed in pancreatic ductal adenocarcinoma and acts cooperatively with macrophage migration inhibitory factor to promote cancer growth. Int. J. Cancer 139, 2056–2067. doi:10.1002/ijc.30278 |
Guo, Z. D., Zhao, L., Wang, P., Deng, W. H., Shi, Q., Zuo, T., et al. (2018). Fetal liver injury ameliorated by migration inhibitory factor inhibition in a rat model of acute pancreatitis in pregnancy. J. Obstet. Gynaecol. Res. 44, 374–383. doi:10.1111/jog.13538 |
Habtezion, A., Gukovskaya, A. S., and Pandol, S. J. (2019). Acute pancreatitis: a multifaceted set of organelle and cellular interactions. Gastroenterology 156, 1941–1950. doi:10.1053/j.gastro.2018.11.082 |
Harper, J. M., Wilkinson, J. E., and Miller, R. A. (2010). Macrophage migration inhibitory factor-knockout mice are long lived and respond to caloric restriction. FASEB J. 24, 2436–2442. doi:10.1096/fj.09-152223 |
Harris, J., Vanpatten, S., Deen, N. S., Al-Abed, Y., and Morand, E. F. (2019). Rediscovering MIF: new tricks for an old cytokine. Trends Immunol. 40, 447–462. doi:10.1016/j.it.2019.03.002 |
He, X. X., Chen, K., Yang, J., Li, X. Y., Gan, H. Y., Liu, C. Y., et al. (2009). Macrophage migration inhibitory factor promotes colorectal cancer. Mol. Med. 15, 1–10. doi:10.2119/molmed.2008.00107 |
Hertelendy, J., Reumuth, G., Simons, D., Stoppe, C., Kim, B. S., Stromps, J. P., et al. (2018). Macrophage migration inhibitory factor—a favorable marker in inflammatory diseases? Curr. Med. Chem. 25, 601–605. doi:10.2174/0929867324666170714114200 |
Hudson, J. D., Shoaibi, M. A., Maestro, R., Carnero, A., Hannon, G. J., and Beach, D. H. (1999). A proinflammatory cytokine inhibits p53 tumor suppressor activity. J. Exp. Med. 190, 1375–1382. doi:10.1084/jem.190.10.1375 |
Hussain, F., Freissmuth, M., Völkel, D., Thiele, M., Douillard, P., Antoine, G., et al. (2013). Human anti-macrophage migration inhibitory factor antibodies inhibit growth of human prostate cancer cells in vitro and in vivo. Mol. Cancer Ther. 12, 1223–1234. doi:10.1158/1535-7163.MCT-12-0988 |
Illescas, O., Pacheco-Fernández, T., Laclette, J. P., Rodriguez, T., and Rodriguez-Sosa, M. (2020). Immune modulation by the macrophage migration inhibitory factor (MIF) family: D-dopachrome tautomerase (DDT) is not (always) a backup system. Cytokine 133, 155121. doi:10.1016/j.cyto.2020.155121 |
Ioanou, K., Cheng, K. F., Crich Low, G. V., Birmpilis, A. I., Lo Lis, E. J., Tsitsilonis, O. E., et al. (2014). ISO-66, a novel inhibitor of macrophage migration inhibitory factor, shows efficacy in melanoma and colon cancer models. Int. J. Oncol. 45, 1457–1468. doi:10.3892/ijo.2014.2551 |
Jankauskas, S. S., Wong, D. W. L., Bucala, R., Djudjaj, S., and Boor, P. (2019). Evolving complexity of MIF signaling. Cell Signal. 57, 76–88. doi:10.1016/j.cellsig.2019.01.006 |
Johnson, D. E., O’keefe, R. A., and Grandis, J. R. (2018). Targeting the IL-6/JAK/STAT3 signalling axis in cancer. Nat. Rev. Clin. Oncol. 15, 234–248. doi:10.1038/nrclinonc.2018.8 |
Kaufman, J. L., Niesvizky, R., Stadtmauer, E. A., Chanan-Khan, A., Siegel, D., Horne, H., et al. (2013). Phase I, multicentre, dose-escalation trial of monotherapy with milatuzumab (humanized anti-CD74 monoclonal antibody) in relapsed or refractory multiple myeloma. Br. J. Haematol. 163, 478–486. doi:10.1111/bjh.12565 |
Kim, B. S., Pallua, N., Bernhagen, J., and Bucala, R. (2015). The macrophage migration inhibitory factor protein superfamily in obesity and wound repair. Exp. Mol. Med. 47, e161. doi:10.1038/emm.2015.26 |
Kindt, N., Preillon, J., Kaltner, H., Gabius, H. J., Chevalier, D., Rodriguez, A., et al. (2013). Macrophage migration inhibitory factor in head and neck squamous cell carcinoma: clinical and experimental studies. J. Cancer Res. Clin. Oncol. 139, 727–737. doi:10.1007/s00432-013-1375-7 |
Kleemann, R., Hausser, A., Geiger, G., Mischke, R., Burger-Kentischer, A., Flieger, O., et al. (2000). Intracellular action of the cytokine MIF to modulate AP-1 activity and the cell cycle through Jab1. Nature 408, 211–216. doi:10.1038/35041591 |
Kok, T., Wasiel, A. A., Cool, R. H., Melgert, B. N., Poelarends, G. J., and Dekker, F. J. (2018). Small-molecule inhibitors of macrophage migration inhibitory factor (MIF) as an emerging class of therapeutics for immune disorders. Drug Discov. Today 23, 1910–1918. doi:10.1016/j.drudis.2018.06.017 |
Kondo, S., Ueno, H., Hosoi, H., Hashimoto, J., Morizane, C., Koizumi, F., et al. (2013). Clinical impact of pentraxin family expression on prognosis of pancreatic carcinoma. Br. J. Cancer 109, 739–746. doi:10.1038/bjc.2013.348 |
Lang, T., Lee, J. P. W., Elgass, K., Pinar, A. A., Tate, M. D., Aitken, E. H., et al. (2018). Macrophage migration inhibitory factor is required for NLRP3 inflammasome activation. Nat. Commun. 9, 2223. doi:10.1038/s41467-018-04581-2 |
Leaver, S. K., Maccallum, N. S., Pingle, V., Hacking, M. B., Quinlan, G. J., Evans, T. W., et al. (2010). Increased plasma thioredoxin levels in patients with sepsis: positive association with macrophage migration inhibitory factor. Intensive Care Med. 36, 336–341. doi:10.1007/s00134-009-1640-z |
Lee, J. P., Foote, A., Fan, H., Peral De Castro, C., Lang, T., Jones, S. A., et al. (2016). Loss of autophagy enhances MIF/macrophage migration inhibitory factor release by macrophages. Autophagy 12, 907–916. doi:10.1080/15548627.2016.1164358 |
Lee, P. J., and Papachristou, G. I. (2019). New insights into acute pancreatitis. Nat. Rev. Gastroenterol. Hepatol. 16, 479–496. doi:10.1038/s41575-019-0158-2 |
Leng, L., Metz, C. N., Fang, Y., Xu, J., Donnelly, S., Baugh, J., et al. (2003). MIF signal transduction initiated by binding to CD74. J. Exp. Med. 197, 1467–1476. doi:10.1084/jem.20030286 |
Li, M., Yu, J., Zhao, L., Mei, F. C., Zhou, Y., Hong, Y. P., et al. (2019). Inhibition of macrophage migration inhibitory factor attenuates inflammation and fetal kidney injury in a rat model of acute pancreatitis in pregnancy. Int. Immunopharmacol 68, 106–114. doi:10.1016/j.intimp.2018.12.068 |
Linkermann, A., Stockwell, B. R., Krautwald, S., and Anders, H. J. (2014). Regulated cell death and inflammation: an auto-amplification loop causes organ failure. Nat. Rev. Immunol. 14, 759–767. doi:10.1038/nri3743 |
Lippitz, B. E. (2013). Cytokine patterns in patients with cancer: a systematic review. Lancet Oncol. 14, e218–28. doi:10.1016/S1470-2045(12)70582-X |
Liu, H., Chen, G., Zhang, W., Zhu, J. Y., Lin, Z. Q., Gong, Z. C., et al. (2013). Overexpression of macrophage migration inhibitory factor in adenoid cystic carcinoma: correlation with enhanced metastatic potential. J. Cancer Res. Clin. Oncol. 139, 287–295. doi:10.1007/s00432-012-1330-z |
Lue, H., Thiele, M., Franz, J., Dahl, E., Speckgens, S., Leng, L., et al. (2007). Macrophage migration inhibitory factor (MIF) promotes cell survival by activation of the Akt pathway and role for CSN5/JAB1 in the control of autocrine MIF activity. Oncogene 26, 5046–5059. doi:10.1038/sj.onc.1210318 |
Lugea, A., Waldron, R. T., Mareninova, O. A., Shalbueva, N., Deng, N., Su, H. Y., et al. (2017). Human pancreatic acinar cells: proteomic characterization, physiologic responses, and organellar disorders in ex Vivo pancreatitis. Am. J. Pathol. 187, 2726–2743. doi:10.1016/j.ajpath.2017.08.017 |
Mahalingam, D., Patel, M. R., Sachdev, J. C., Hart, L. L., Halama, N., Ramanathan, R. K., et al. (2020). Phase I study of imalumab (BAX69), a fully human recombinant antioxidized macrophage migration inhibitory factor antibody in advanced solid tumours. Br. J. Clin. Pharmacol. 86, 1836–1848. doi:10.1111/bcp.14289 |
Makhija, R., Kingsnorth, A., and Demaine, A. (2007). Gene polymorphisms of the macrophage migration inhibitory factor and acute pancreatitis. Jop 8, 289–295.
Matsuda, N., Nishihira, J., Takahashi, Y., Kemmotsu, O., and Hattori, Y. (2006). Role of macrophage migration inhibitory factor in acute lung injury in mice with acute pancreatitis complicated by endotoxemia. Am. J. Respir. Cell Mol. Biol. 35, 198–205. doi:10.1165/rcmb.2005-0272OC |
Mawhinney, L., Armstrong, M. E., O’ Reilly, C., Bucala, R., Leng, L., Fingerle-Rowson, G., et al. (2015). Macrophage migration inhibitory factor (MIF) enzymatic activity and lung cancer. Mol. Med. 20, 729–735. doi:10.2119/molmed.2014.00136 |
Merk, M., Zierow, S., Leng, L., Das, R., Du, X., Schulte, W., et al. (2011). The D-dopachrome tautomerase (DDT) gene product is a cytokine and functional homolog of macrophage migration inhibitory factor (MIF). Proc. Natl. Acad. Sci. USA 108, E577–E585. doi:10.1073/pnas.1102941108 |
Meyer-Siegler, K. L., Bellino, M. A., and Tannenbaum, M. (2002). Macrophage migration inhibitory factor evaluation compared with prostate specific antigen as a biomarker in patients with prostate carcinoma. Cancer 94, 1449–1456. doi:10.1002/cncr.10354 |
Mikulowska, A., Metz, C. N., Bucala, R., and Holmdahl, R. (1997). Macrophage migration inhibitory factor is involved in the pathogenesis of collagen type II-induced arthritis in mice. J. Immunol. 158, 5514–5517.
Mitchell, R. A., Metz, C. N., Peng, T., and Bucala, R. (1999). Sustained mitogen-activated protein kinase (MAPK) and cytoplasmic phospholipase A2 activation by macrophage migration inhibitory factor (MIF). Regulatory role in cell proliferation and glucocorticoid action. J. Biol. Chem. 274, 18100–18106. doi:10.1074/jbc.274.25.18100 |
Mitchell, R. A., Liao, H., Chesney, J., Fingerle-Rowson, G., Baugh, J., David, J., et al. (2002). Macrophage migration inhibitory factor (MIF) sustains macrophage proinflammatory function by inhibiting p53: regulatory role in the innate immune response. Proc. Natl. Acad. Sci. USA 99, 345–350. doi:10.1073/pnas.012511599
Moggia, E., Koti, R., Belgaumkar, A. P., Fazio, F., Pereira, S. P., Davidson, B. R., et al. (2017). Pharmacological interventions for acute pancreatitis. Cochrane Database Syst. Rev. 4, CD011384. doi:10.1002/14651858.CD011384.pub2 |
Morrison, M. C., and Kleemann, R. (2015). Role of macrophage migration inhibitory factor in obesity, insulin resistance, type 2 diabetes, and associated hepatic Co-morbidities: a comprehensive review of human and rodent studies. Front. Immunol. 6, 308. doi:10.3389/fimmu.2015.00308 |
Munaut, C., Boniver, J., Foidart, J. M., and Deprez, M. (2002). Macrophage migration inhibitory factor (MIF) expression in human glioblastomas correlates with vascular endothelial growth factor (VEGF) expression. Neuropathol. Appl. Neurobiol. 28, 452–460. doi:10.1046/j.1365-2990.2002.00416.x |
Nishibori, M., Nakaya, N., Tahara, A., Kawabata, M., Mori, S., and Saeki, K. (1996). Presence of macrophage migration inhibitory factor (MIF) in ependyma, astrocytes and neurons in the bovine brain. Neurosci. Lett. 213, 193–196. doi:10.1016/0304-3940(96)12864-0 |
Nishihira, J., Fujinaga, M., Kuriyama, T., Suzuki, M., Sugimoto, H., Nakagawa, A., et al. (1998). Molecular cloning of human D-dopachrome tautomerase cDNA: N-terminal proline is essential for enzyme activation. Biochem. Biophys. Res. Commun. 243, 538–544. doi:10.1006/bbrc.1998.8123 |
O’reilly, C., Doroudian, M., Mawhinney, L., and Donnelly, S. C. (2016). Targeting MIF in cancer: therapeutic strategies, current developments, and future opportunities. Med. Res. Rev. 36, 440–460. doi:10.1002/med.21385 |
Oda, S., Oda, T., Nishi, K., Takabuchi, S., Wakamatsu, T., Tanaka, T., et al. (2008). Macrophage migration inhibitory factor activates hypoxia-inducible factor in a p53-dependent manner. PLoS One 3, e2215. doi:10.1371/journal.pone.0002215 |
Ohkawara, T., Nishihira, J., Takeda, H., Hige, S., Kato, M., Sugiyama, T., et al. (2002). Amelioration of dextran sulfate sodium-induced colitis by anti-macrophage migration inhibitory factor antibody in mice. Gastroenterology 123, 256–270. doi:10.1053/gast.2002.34236 |
Ohkawara, T., Takeda, H., and Nishihira, J. (2017). Protective effect of chlorogenic acid on the inflammatory damage of pancreas and lung in mice with l-arginine-induced pancreatitis. Life Sci. 190, 91–96. doi:10.1016/j.lfs.2017.09.015 |
Oliveira, C. S., De Bock, C. E., Molloy, T. J., Sadeqzadeh, E., Geng, X. Y., Hersey, P., et al. (2014). Macrophage migration inhibitory factor engages PI3K/Akt signalling and is a prognostic factor in metastatic melanoma. BMC Cancer 14, 630. doi:10.1186/1471-2407-14-630 |
Paszt, A., Eder, K., Szabolcs, A., Tiszlavicz, L., Lázár, G., Duda, E., et al. (2008). Effects of glucocorticoid agonist and antagonist on the pathogenesis of L-arginine-induced acute pancreatitis in rat. Pancreas 36, 369–376. doi:10.1097/MPA.0b013e31815bd26a |
Peery, A. F., Crockett, S. D., Murphy, C. C., Lund, J. L., Dellon, E. S., Williams, J. L., et al. (2019). Burden and cost of gastrointestinal, liver, and pancreatic diseases in the United States: update 2018. Gastroenterology 156, 254–e11. doi:10.1053/j.gastro.2018.08.063 |
Petrov, M. S., and Yadav, D. (2019). Global epidemiology and holistic prevention of pancreatitis. Nat. Rev. Gastroenterol. Hepatol. 16, 175–184. doi:10.1038/s41575-018-0087-5 |
Petrovsky, N., Socha, L., Silva, D., Grossman, A. B., Metz, C., and Bucala, R. (2003). Macrophage migration inhibitory factor exhibits a pronounced circadian rhythm relevant to its role as a glucocorticoid counter-regulator. Immunol. Cel Biol 81, 137–143. doi:10.1046/j.0818-9641.2002.01148.x
Rahman, S. H., Menon, K. V., Holmfield, J. H., Mcmahon, M. J., and Guillou, J. P. (2007). Serum macrophage migration inhibitory factor is an early marker of pancreatic necrosis in acute pancreatitis. Ann. Surg. 245, 282–289. doi:10.1097/01.sla.0000245471.33987.4b |
Roger, T., David, J., Glauser, M. P., and Calandra, T. (2001). MIF regulates innate immune responses through modulation of Toll-like receptor 4. Nature 414, 920–924. doi:10.1038/414920a |
Rosengren, E., Bucala, R., Åman, P., Jacobsson, L., Odh, G., Metz, C. N., et al. (1996). The immunoregulatory mediator macrophage migration inhibitory factor (MIF) catalyzes a tautomerization reaction. Mol. Med. 2, 143–149. doi:10.1007/BF03402210 |
Rosengren, E., Åman, P., Thelin, S., Hansson, C., Ahlfors, S., Björk, P., et al. (1997). The macrophage migration inhibitory factor MIF is a phenylpyruvate tautomerase. FEBS Lett. 417, 85–88. doi:10.1016/s0014-5793(97)01261-1 |
Russell, K. E., Chung, K. F., Clarke, C. J., Durham, A. L., Mallia, P., Footitt, J., et al. (2016). The MIF antagonist ISO-1 attenuates corticosteroid-insensitive inflammation and airways hyperresponsiveness in an ozone-induced model of COPD. PLoS One 11, e0146102. doi:10.1371/journal.pone.0146102 |
Ryan, D. P., Hong, T. S., and Bardeesy, N. (2014). Pancreatic adenocarcinoma. N. Engl. J. Med. 371, 1039–1049. doi:10.1056/NEJMra1404198 |
Sakai, Y., Masamune, A., Satoh, A., Nishihira, J., Yamagiwa, T., and Shimosegawa, T. (2003). Macrophage migration inhibitory factor is a critical mediator of severe acute pancreatitis. Gastroenterology 124, 725–736. doi:10.1053/gast.2003.50099 |
Saluja, A., Dudeja, V., Dawra, R., and Sah, R. P. (2019). Early intra-acinar events in pathogenesis of pancreatitis. Gastroenterology 156, 1979–1993. doi:10.1053/j.gastro.2019.01.268 |
Sankaran, S. J., Xiao, A. Y., Wu, L. M., Windsor, J. A., Forsmark, C. E., and Petrov, M. S. (2015). Frequency of progression from acute to chronic pancreatitis and risk factors: a meta-analysis. Gastroenterology 149, 1490–e1491. doi:10.1053/j.gastro.2015.07.066 |
Schinagl, A., Thiele, M., Douillard, P., Völkel, D., Kenner, L., Kazemi, Z., et al. (2016). Oxidized macrophage migration inhibitory factor is a potential new tissue marker and drug target in cancer. Oncotarget 7, 73486–73496. doi:10.18632/oncotarget.11970 |
Schwartz, V., Lue, H., Kraemer, S., Korbiel, J., Krohn, R., Ohl, K., et al. (2009). A functional heteromeric MIF receptor formed by CD74 and CXCR4. FEBS Lett. 583, 2749–2757. doi:10.1016/j.febslet.2009.07.058 |
Sendler, M., Van Den Brandt, C., Glaubitz, J., Wilden, A., Golchert, J., Weiss, F. U., et al. (2020). NLRP3 inflammasome regulates development of systemic inflammatory response and compensatory anti-inflammatory response syndromes in mice with acute pancreatitis. Gastroenterology 158, 253–e214. doi:10.1053/j.gastro.2019.09.040 |
Sharif, R., Dawra, R., Wasiluk, K., Phillips, P., Dudeja, V., Kurt-Jones, E., et al. (2009). Impact of toll-like receptor 4 on the severity of acute pancreatitis and pancreatitis-associated lung injury in mice. Gut 58, 813–819. doi:10.1136/gut.2008.170423 |
Shen, D., Tang, C., Zhu, S., and Huang, G. (2021). Macrophage migration inhibitory factor is an early marker of severe acute pancreatitis based on the revised Atlanta classification. BMC Gastroenterol. 21, 34. doi:10.1186/s12876-020-01598-0 |
Shi, X., Leng, L., Wang, T., Wang, W., Du, X., Li, J., et al. (2006). CD44 is the signaling component of the macrophage migration inhibitory factor-CD74 receptor complex. Immunity 25, 595–606. doi:10.1016/j.immuni.2006.08.020 |
Shin, M. S., Kang, Y., Lee, N., Kim, S. H., Kang, K. S., Lazova, R., et al. (2012). U1-Small nuclear ribonucleoprotein activates the NLRP3 inflammasome in human monocytes. J. Immunol. 188, 4769–4775. doi:10.4049/jimmunol.1103355 |
Shin, M. S., Kang, Y., Wahl, E. R., Park, H. J., Lazova, R., Leng, L., et al. (2019). Macrophage migration inhibitory factor regulates U1 small nuclear RNP immune complex-mediated activation of the NLRP3 inflammasome. Arthritis Rheumatol. 71, 109–120. doi:10.1002/art.40672 |
Siegel, R. L., Miller, K. D., and Jemal, A. (2019). Cancer statistics, 2019. CA Cancer J. Clin. 69, 7–34. doi:10.3322/caac.21551 |
Sparkes, A., De Baetselier, P., Brys, L., Cabrito, I., Sterckx, Y. G., Schoonooghe, S., et al. (2018). Novel half-life extended anti-MIF nanobodies protect against endotoxic shock. FASEB J. 32, 3411–3422. doi:10.1096/fj.201701189R |
Sparkes, A., De Baetselier, P., Roelants, K., De Trez, C., Magez, S., Van Ginderachter, J. A., et al. (2017). Reprint of: the non-mammalian MIF superfamily. Immunobiology 222, 858–867. doi:10.1016/j.imbio.2017.05.004 |
Spencer, E. S., Dale, E. J., Gommans, A. L., Rutledge, M. T., Vo, C. T., Nakatani, Y., et al. (2015). Multiple binding modes of isothiocyanates that inhibit macrophage migration inhibitory factor. Eur. J. Med. Chem. 93, 501–510. doi:10.1016/j.ejmech.2015.02.012 |
Stein, R., Qu, Z., Cardillo, T. M., Chen, S., Rosario, A., Horak, I. D., et al. (2004). Antiproliferative activity of a humanized anti-CD74 monoclonal antibody, hLL1, on B-cell malignancies. Blood 104, 3705–3711. doi:10.1182/blood-2004-03-0890 |
Stein, R., Smith, M. R., Chen, S., Zalath, M., and Goldenberg, D. M. (2009). Combining milatuzumab with bortezomib, doxorubicin, or dexamethasone improves responses in multiple myeloma cell lines. Clin. Cancer Res. 15, 2808–2817. doi:10.1158/1078-0432.CCR-08-1953 |
Steinberg, M., and Silva, M. (2010). Plerixafor: a chemokine receptor-4 antagonist for mobilization of hematopoietic stem cells for transplantation after high-dose chemotherapy for non-Hodgkin’s lymphoma or multiple myeloma. Clin. Ther. 32, 821–843. doi:10.1016/j.clinthera.2010.05.007 |
Sugimoto, H., Taniguchi, M., Nakagawa, A., Tanaka, I., Suzuki, M., and Nishihira, J. (1999). Crystal structure of human D-dopachrome tautomerase, a homologue of macrophage migration inhibitory factor, at 1.54 A resolution. Biochemistry 38, 3268–3279. doi:10.1021/bi982184o |
Sun, H. W., Bernhagen, J., Bucala, R., and Lolis, E. (1996). Crystal structure at 2.6-A resolution of human macrophage migration inhibitory factor. Proc. Natl. Acad. Sci. USA 93, 5191–5196. doi:10.1073/pnas.93.11.5191 |
Suresh, V., Sundaram, R., Dash, P., Sabat, S. C., Mohapatra, D., Mohanty, S., et al. (2019). Macrophage migration inhibitory factor of Syrian golden hamster shares structural and functional similarity with human counterpart and promotes pancreatic cancer. Sci. Rep. 9, 15507. doi:10.1038/s41598-019-51947-7 |
Suzuki, M., Sugimoto, H., Nakagawa, A., Tanaka, I., Nishihira, J., and Sakai, M. (1996). Crystal structure of the macrophage migration inhibitory factor from rat liver. Nat. Struct. Biol. 3, 259–266. doi:10.1038/nsb0396-259 |
Tan, L., Ye, X., Zhou, Y., Yu, M., Fu, Z., Chen, R., et al. (2014). Macrophage migration inhibitory factor is overexpressed in pancreatic cancer tissues and impairs insulin secretion function of β-cell. J. Transl Med. 12, 92. doi:10.1186/1479-5876-12-92 |
Taniguchi, K., and Karin, M. (2014). IL-6 and related cytokines as the critical lynchpins between inflammation and cancer. Semin. Immunol. 26, 54–74. doi:10.1016/j.smim.2014.01.001 |
Tomiyasu, M., Yoshino, I., Suemitsu, R., Okamoto, T., and Sugimachi, K. (2002). Quantification of macrophage migration inhibitory factor mRNA expression in non-small cell lung cancer tissues and its clinical significance. Clin. Cancer Res. 8, 3755–3760.
Trivedi-Parmar, V., and Jorgensen, W. L. (2018). Advances and insights for small molecule inhibition of macrophage migration inhibitory factor. J. Med. Chem. 61, 8104–8119. doi:10.1021/acs.jmedchem.8b00589 |
Vege, S. S., Dimagno, M. J., Forsmark, C. E., Martel, M., and Barkun, A. N. (2018). Initial medical treatment of acute pancreatitis: American gastroenterological association institute technical review. Gastroenterology 154, 1103–1139. doi:10.1053/j.gastro.2018.01.031 |
Waeber, G., Calandra, T., Roduit, R., Haefliger, J. A., Bonny, C., Thompson, N., et al. (1997). Insulin secretion is regulated by the glucose-dependent production of islet beta cell macrophage migration inhibitory factor. Proc. Natl. Acad. Sci. USA 94, 4782–4787. doi:10.1073/pnas.94.9.4782 |
Wallace, D. J., Wegener, W. A., Horne, H., and Goldenberg, D. M. (2016). CT-01 Phase IB study of IMMU-115 (humanised ANTI-CD74 antibody) targeting antigen presenting cells in patients with systemic lupus erythematosus (SLE). Lupus Sci. Med. 3, A37. doi:10.1136/lupus-2016-000179.73
Wang, B., Zhao, K. L., Hu, W. J., Zuo, T., Ding, Y. M., and Wang, W. X. (2019). Macrophage migration inhibitor promoted the intrahepatic bile duct injury in rats with severe acute pancreatitis. Dig. Dis. Sci. 64, 759–772. doi:10.1007/s10620-018-5379-7 |
Wang, D., Wang, R., Huang, A., Fang, Z., Wang, K., He, M., et al. (2018). Upregulation of macrophage migration inhibitory factor promotes tumor metastasis and correlates with poor prognosis of pancreatic ductal adenocarcinoma. Oncol. Rep. 40, 2628–2636. doi:10.3892/or.2018.6703 |
Winner, M., Koong, A. C., Rendon, B. E., Zundel, W., and Mitchell, R. A. (2007). Amplification of tumor hypoxic responses by macrophage migration inhibitory factor-dependent hypoxia-inducible factor stabilization. Cancer Res. 67, 186–193. doi:10.1158/0008-5472.CAN-06-3292 |
Xiao, A. Y., Tan, M. L., Wu, L. M., Asrani, V. M., Windsor, J. A., Yadav, D., et al. (2016). Global incidence and mortality of pancreatic diseases: a systematic review, meta-analysis, and meta-regression of population-based cohort studies. Lancet Gastroenterol. Hepatol. 1, 45–55. doi:10.1016/S2468-1253(16)30004-8 |
Xu, X., Wang, B., Ye, C., Yao, C., Lin, Y., Huang, X., et al. (2008). Overexpression of macrophage migration inhibitory factor induces angiogenesis in human breast cancer. Cancer Lett. 261, 147–157. doi:10.1016/j.canlet.2007.11.028 |
Xu, L., Li, Y., Sun, H., Zhen, X., Qiao, C., Tian, S., et al. (2013). Current developments of macrophage migration inhibitory factor (MIF) inhibitors. Drug Discov. Today 18, 592–600. doi:10.1016/j.drudis.2012.12.013 |
Xu, X. W., Yang, X. M., Bai, Y. H., Zhao, Y. R., Shi, G. S., Zhang, J. G., et al. (2014). Treatment with ginkgo biloba extract protects rats against acute pancreatitis-associated lung injury by modulating alveolar macrophage. Prz Gastroenterol. 9, 43–48. doi:10.5114/pg.2014.40850 |
Yang, S., He, P., Wang, J., Schetter, A., Tang, W., Funamizu, N., et al. (2016). A novel MIF signaling pathway drives the malignant character of pancreatic cancer by targeting NR3C2. Cancer Res. 76, 3838–3850. doi:10.1158/0008-5472.CAN-15-2841 |
Zhang, M., Aman, P., Grubb, A., Panagopoulos, I., Hindemith, A., Rosengren, E., et al. (1995). Cloning and sequencing of a cDNA encoding rat D-dopachrome tautomerase. FEBS Lett. 373, 203–206. doi:10.1016/0014-5793(95)01041-c |
Zhou, Y., Zhao, L., Mei, F., Hong, Y., Xia, H., Zuo, T., et al. (2018). Macrophage migration inhibitory factor antagonist (S,R)3(4hydroxyphenyl)4,5dihydro5isoxazole acetic acid methyl ester attenuates inflammation and lung injury in rats with acute pancreatitis in pregnancy. Mol. Med. Rep. 17, 6576–6584. doi:10.3892/mmr.2018.8672 |
Keywords: macrophage migration inhibitory factor, acute inflammatory response, toll-like receptor, inflammasome, acute pancreatitis, pancreatic cancer
Citation: Wen Y, Cai W, Yang J, Fu X, Putha L, Xia Q, Windsor JA, Phillips AR, Tyndall JDA, Du D, Liu T and Huang W (2021) Targeting Macrophage Migration Inhibitory Factor in Acute Pancreatitis and Pancreatic Cancer. Front. Pharmacol. 12:638950. doi: 10.3389/fphar.2021.638950
Received: 07 December 2020; Accepted: 29 January 2021;
Published: 11 March 2021.
Edited by:
Gerard Bannenberg, Global Organization for EPA and DHA Omega-3s (GOED), United StatesReviewed by:
Paolo Fagone, University of Catania, ItalyShannon Moonah, University of Virginia, United States
Ana Newton, Yale University, United States
Copyright © 2021 Wen, Cai, Yang, Fu, Putha, Xia, Windsor, Phillips, Tyndall, Du, Liu and Huang. This is an open-access article distributed under the terms of the Creative Commons Attribution License (CC BY). The use, distribution or reproduction in other forums is permitted, provided the original author(s) and the copyright owner(s) are credited and that the original publication in this journal is cited, in accordance with accepted academic practice. No use, distribution or reproduction is permitted which does not comply with these terms.
*Correspondence: Dan Du, dudan1520@163.com; Tingting Liu, tingting_liu66@163.com
†These authors have contributed equally to this work and share first authorship