Downstream fish passage at small-scale hydropower plants: Turbine or bypass?
- Aquatic Systems Biology, TUM School of Life Sciences, Technical University of Munich, Freising, Germany
Introduction: Hydropower plants are frequently equipped with physical and behavioral fish protection barriers to prevent downstream moving fish from harmful turbine passage and to guide them to alternative bypasses. As not only diadromous but also potamodromous fish species migrate and inevitably have to pass hydropower plants, knowledge on corridor usage for a wide range of species is important to identify potential deficits and to improve bypass efficiency.
Methods: In this study, the corridor usage of downstream moving fish (6,646 individuals from 42 species) was investigated at four small-scale hydropower plants with different concepts to prevent turbine entrainment and to bypass fish.
Results: Despite existing bypasses and fine screens with 15 mm and 20 mm bar spacing to prevent turbine entrainment, a large proportion of fish (35%–88%) still passed the turbines. The mainly poor efficiency of the investigated bypasses was probably due to low discharge and unfavorable bypass location or detectability. The various bypass types were used by a different range of fish species and sizes due to species-specific behavior and differing fish communities between sites. The effectiveness of the investigated downstream corridors was positively correlated with the share of discharge.
Discussion: To reduce the negative ecological impacts of hydropower plants on downstream moving fish, well-performing bypasses are required that consider not only current requirements regarding design, dimensioning and location, but also the site-specific fish community. Thus, bypasses should function for the widest possible range of species, which can be achieved through less selective bypass types such as full-depth bypasses, or a combination of different bypass systems. Moreover, less harmful turbine technologies and more effective fish protection systems need to be implemented, since fine screens with 15 mm and 20 mm bar spacing cannot prevent small-bodied fish species and juvenile fish <20 cm from turbine entrainment.
1 Introduction
Throughout the globe, weirs and dams, often combined with hydropower use, form barriers for migratory fish species (Gehrke et al., 2002; Dugan et al., 2010; Pelicice et al., 2015). The resulting negative effects of blocked migration routes, for example, on the accessibility of suitable spawning sites, annual recruitment success or genetic exchange, are well-known (e.g., Antonio et al., 2007; Fukushima et al., 2007; Liermann et al., 2012).
Generally, a distinction needs to me made between upstream and downstream migration of fish since both follow different principles (Geist, 2021). For decades, intensive efforts have been made to make migration barriers passable for upstream migrating fish species through provisioning alternative corridors, e.g., various types of fish ladders (Banks, 1969; Evans and Johnston, 1980). In contrast, the great importance of suitable bypasses that enable downstream moving fish to pass a barrier without being seriously injured was underestimated for a long time (Larinier and Travade, 2002).
Particularly the passage of conventional hydropower turbines (e.g., Francis, Kaplan turbines) poses a high injury and mortality risk for fish (Pracheil et al., 2016; Algera et al., 2020; Mueller et al., 2022). As fish primarily follow the main current during their downstream migration to save energy (Williams et al., 2012; Anderson et al., 2015) and the bulk of discharge typically flows through the turbines at most hydropower plants (HPPs), a large proportion of active or passive downstream moving fish also follows this route (Fjeldstad et al., 2018). To prevent fish from passing through the turbines and to direct them to alternative downstream corridors, so-called “bypasses”, various types of physical and behavioral barriers are used that are commonly installed upstream of the turbine inlet. Typical physical barriers are bar or mesh screens designed to block fish from swimming through whose body dimensions are larger than the clearance between the openings (Ebel, 2013; Schwevers and Adam, 2020). In contrast, behavioral barriers are intended to induce an avoidance or scaring reaction in fish through external stimuli such as electricity, light, sound or air bubbles (e.g., Schilt, 2007; Noatch and Suski, 2012). Recently, so-called “hybrid” barriers have also been developed, where physical barriers (e.g., bar screens) are combined with electric fields to provide an additional behavioral barrier (Tutzer et al., 2021, 2022; Haug et al., 2022).
Corridors specifically built for downstream migrating fish, such as surface, bottom or full-depth bypasses, should provide an alternative route to the turbine corridor and transport fish unharmed into the tailwater. In addition, structures to divert flotsam or floodwater to the tailwater such as flushing channels, spillways, periodically opened flap gates and sluices as well as fish passes that were actually built to enable upstream migration can also be used by downstream moving fish (e.g., Scruton et al., 2007; Travade et al., 2010; Egg et al., 2017). Recent empirical evidence suggests that larger fish than expected from modelling approaches can pass through physical screens at hydropower inlets (Knott et al., 2023), emphasizing the necessity of better understanding the roles of alternative corridors for their safe downstream passage.
However, there are also HPPs where fish protection measures and bypasses for downstream migrating fish are deliberately not in place [e.g., at innovative Archimedes screw turbines or very low-head (VLH) turbines], as it is assumed that the installed turbine technology should cause less mortality and injuries than conventional turbine types and therefore fish passage via the turbine corridor is intended (Anderson et al., 2015; Simmons and Lubitz, 2021).
In general, bypass efficiency depends not only on the available discharge, but also on bypass dimensioning, spatial proximity to fish guiding structures, location in the water column (top, middle, bottom) and prevailing hydraulic conditions (Larinier and Travade, 2002; Katopodis, 2005). Since these variables can differ considerably among sites, even the efficiency of state-of-the-art bypass systems can vary strongly depending on the local conditions.
For economically important fish species such as salmon and eel, there is already a large number of studies on the effectiveness of fish guiding structures and bypasses for downstream migration, mostly relying on telemetry or sonar detection (e.g., Gosset et al., 2005; Havn et al., 2017; Nyqvist et al., 2018). In contrast, research on corridor usage during downstream passage at hydropower facilities of fish species with little or no perceived economic value (particularly potamodromous species) is severely underrepresented. However, knowledge on the downstream movement behavior of the whole fish community is important, as many potamodromous fish species that are not known to be migratory species also move downstream and inevitably have to pass HPPs (Katopodis, 2005; Knott et al., 2020). Since there can be large differences between fish species in terms of morphology (e.g., body size, body shape) and behavior (e.g., habitat use, swimming behavior), it is often not known which corridors the various fish species preferentially use to pass barriers such as HPPs. Consequently, studies are needed that assess the corridor usage for a broad range of species in order to identify possible deficits and to improve bypass efficiency. This would help to better protect not only economically important species such as the European eel (Anguilla anguilla L.) from negative ecological effects of hydropower, but also other species such as the small-bodied bullhead (Cottus gobio L.), which is also threatened and protected by law (European Commission, 1992).
In this study, we investigated the corridor usage of downstream moving fish at four hydropower sites by a net-based monitoring during different seasons. For this purpose, downstream moving fish were captured and examined after turbine passage and the passage of alternative downstream corridors such as surface and bottom bypasses, flushing channels, flap gates and fish passes.
Specifically, we hypothesized that (i) the majority of downstream moving fish are guided towards alternative bypasses due to the physical and behavioral barrier function of the fine screens installed in front of the turbine inlets and that (ii) the sampled downstream corridors at a specific site differ in terms of number, size and species composition of downstream moving fish due to differing fish communities and species-specific behavior.
2 Materials and methods
2.1 Study sites
This study was performed at four small-scale (<1 MW) run-of-the-river HPPs in Bavaria, Germany (Figure 1). The investigated HPPs are all equipped with horizontal Kaplan bulb turbines, but differ in their concepts to protect fish from turbine passage and to bypass downstream moving fish (Figure 1; Table 1). At the HPP Lindesmuehle (N 50.1879, E 10.0744), a fine screen with horizontally aligned bars (bar spacing 15 mm) is installed in front of the turbine inlet at an angle of 30° to the flow direction to protect fish from turbine passage. Upstream and downstream fish passage is enabled by a nature-like fish pass. Downstream moving fish can also pass the HPP via a fish slide that has several bottom entrances in a zig-zag shaped tube placed in front of the screen and one near-surface entry (cf. Hassinger and Huebner, 2009; Egg et al., 2017) or via the periodically opening flap gate. The opening of the flap gate is linked to the screen cleaning process, which can vary between several times per hour and once a day during regular operation, depending on the amount of flotsam at the screen. During the investigations the flap gate was opened manually once per net emptying for standardization and personal safety reasons. At the HPP Baiersdorf-Wellerstadt (N 49.6706, E 11.0424), a vertical fine screen with a bar spacing of 15 mm, installed in front of the turbine inlet (inclination to streambed 27°), should prevent fish from turbine entrainment. A flushing channel at the upper end of the screen is intended to guide fish into the tailrace. Upstream and downstream fish passage is enabled by a vertical-slot fish pass. At the HPP Hoellthal (N 47.9780, E 12.5027), a vertical fine screen with a bar spacing of 20 mm is installed upstream of the turbine inlet at an angle of 45° towards the streambed as fish exclusion measure. Downstream moving fish can also get into the tailwater via another HPP, which is installed next to the Kaplan HPP in the same channel and equipped with two Archimedes screw turbines (no fine screen, horizontal trash rack with 150 mm bar spacing). Furthermore, fish can pass the Kaplan HPP during the screen cleaning process via a periodically opened flushing channel at the downstream end of the screen, which could not be investigated for technical reasons. At the assessed innovative “shaft” HPP Großweil (N 47.6819, E 11.3002), both Kaplan turbines are installed in a vertical shaft beneath the river bottom. A fine screen with a bar spacing of 20 mm installed horizontally on top of the shaft should act as a physical and behavioral barrier and guide fish towards the bypasses (one bottom and two surface bypasses in the sluice gates at the downstream end of the screen). Downstream fish passage is also possible through a vertical-slot fish pass on each bank constructed to facilitate upstream migration (Figure 1).
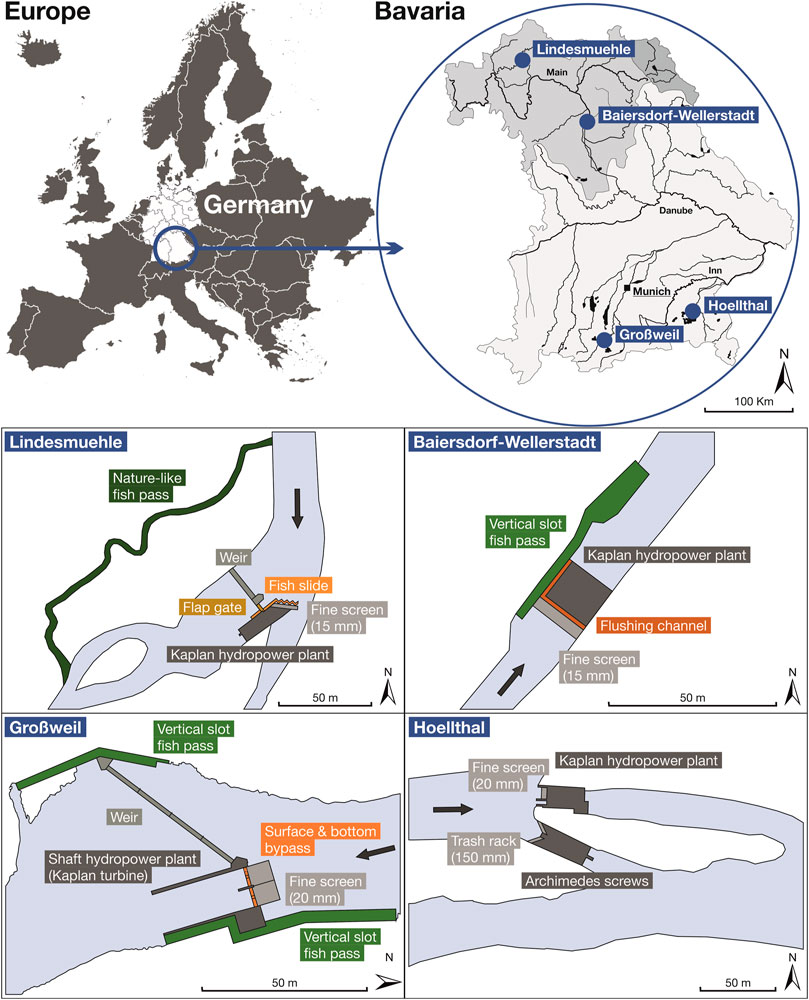
FIGURE 1. Location of the study sites in Bavaria, Germany (upper part of the figure) and sketch-map from a bird’s eye view of the assessed corridors at the different hydropower sites (lower part). Grey arrows indicate the main flow direction.
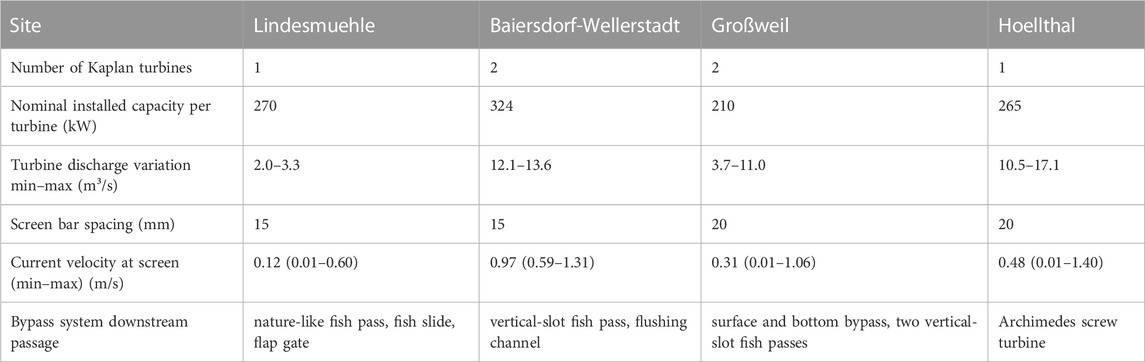
TABLE 1. Characteristics and operating conditions of the hydropower plants and assessed bypass systems for downstream fish passage.
2.2 Experimental design
In order to examine the corridor usage at the different HPPs, downstream moving fish passing the HPPs were captured with stow-nets. All possible corridors into the tailrace (i.e., turbine outlets, surface and bottom bypasses, flap gates, flushing channels, fish slides and fish passes) were sampled. Funnel-shaped stow-nets with decreasing mesh size (mesh sizes: 30 mm, 20 mm, 15 mm, 10 mm and 8 mm) and a fyke-net attached to the end were used. The openings of the stow-nets were fixed to rectangular metal frames, which were inserted into the u-profiles of the stop logs at the turbine outlet or at the downstream end of the bypasses to cover the entire discharge cross-section of the respective corridor (cf. Knott et al., 2020) (see Supplementary Table S1 for detailed dimensions). All stow- and fyke-nets were made of knotless polyamide netting material and were emptied regularly during day and night to minimize catch-related damage to fish (Pander et al., 2018). Captured fish were determined to species level and their total length (TL) was measured. The net sampling was carried out over a period of 1,538 h on a total of 140 study days between 2015 and 2021. Each study site was assessed for several weeks in spring and autumn, which is an ideal period for the assessment of migrating fish in Central Europe (Lucas and Baras, 2001). A relatively wide range of water temperatures was covered for the respective study rivers (min–max: 3.7°C–22.9°C; Supplementary Table S2), a factor known to have a strong influence on fish migrations (Jonsson, 1991; Wiesner et al., 2004). The current velocity (m/s) immediately upstream of the screen at the turbine inlet was measured three times a day at twelve different points each using an electromagnetic water flow meter (Ott MF Pro; Ott HydroMet, Kempten, Germany). In addition, turbidity (NTU), dissolved oxygen (mg/L), water temperature (°C), pH-value and electric conductivity (µS/cm; based on 25°C) were measured using hand-held probes (PhotoFlex Turb 3,430, Multi 3,430; WTW, Weilheim, Germany) three times a day in all study rivers. The river discharge (m³/s) at the nearest water gauge of the investigated hydropower sites was also recorded three times a day (see Supplementary Table S2 for abiotic characterization of study rivers).
2.3 Data analysis
To analyze the corridor usage of downstream moving fish at the four investigated hydropower sites, captured individuals per hour, TLs of captured individuals as well as the percentage of captured individuals by size classes ≤10 cm, >10 ≤ 20 cm, >20 ≤ 30 cm, >30 ≤ 40 cm and >40 cm were compared using the statistics software R (version 4.2.2; R Core Team, 2022). Wilcoxon tests (comparison of two groups) or non-parametric Kruskal–Wallis tests and Bonferroni-corrected post hoc pairwise Mann-Whitney U tests (comparison of more than two groups) were performed to determine statistical differences. Differences in the number of captured individuals per hour and TL between corridors were visualized with raincloud plots (Allen et al., 2021) from the R package ggplot2 (Wickham, 2016). The percentages of captured fish of a given size class that moved downstream via the turbines and the bypasses were compared using Two-proportions z-test with Yates continuity correction. Linear regressions were used to analyze relationships between captured fish and discharge, with “percentage of corridor discharge” as predictor variable and “percentage of captured fish” in the respective corridor as response variable. To identify the most frequent and steadily occurring species and to determine the contribution to the between-group dissimilarity between bypass and turbine passage, one-way Similarity Percentage analyses (SIMPER; Clarke et al., 2014) were carried out using the statistic software PRIMER v7 (PRIMER-e, Massey University, Auckland, NZ). Statistical test results were classified as significant at p ≤ 0.05.
3 Results
During the study period, 6,646 downstream moving fish from 42 species were caught after passing the different corridors of the investigated HPPs. The most frequently caught species were spirlin (Alburnoides bipunctatus L.), bleak (Alburnus alburnus L.) and roach (Rutilus rutilus L.), summing up to 42% of the total catch of individuals (Supplementary Table S3). TLs of all captured fish ranged from 2 cm (Rudd Scardinius erythrophthalmus L., Tench Tinca tinca L., Topmouth gudgeon Pseudorasbora parva Temminck and Schlegel) to 85 cm (European eel) (a.m.±s.d.: 10.2 ± 5.6 cm). Small-bodied species and juvenile fish <20 cm TL accounted for 96% of the total number of captured fish, 3% were between 20 cm and 40 cm TL and 1% were >40 cm (mostly European eels).
3.1 Comparison of catch numbers between corridors
At the study sites Lindesmuehle, Baiersdorf-Wellerstadt and Hoellthal, significantly more fish per hour moved downstream via the Kaplan turbine corridor than via the respective alternative corridors (Figure 2). At the Großweil site, there was no significant difference in downstream movement rates between turbine corridor, surface- and bottom-near bypasses and the two fish passes (Figure 2). The number of captured fish per hour in the fish passes at Lindesmuehle and Baiersdorf-Wellerstadt was significantly higher than in the bypasses fish slide, flap gate (Lindesmuehle) and flushing channel (Baiersdorf-Wellerstadt) (Figure 2).
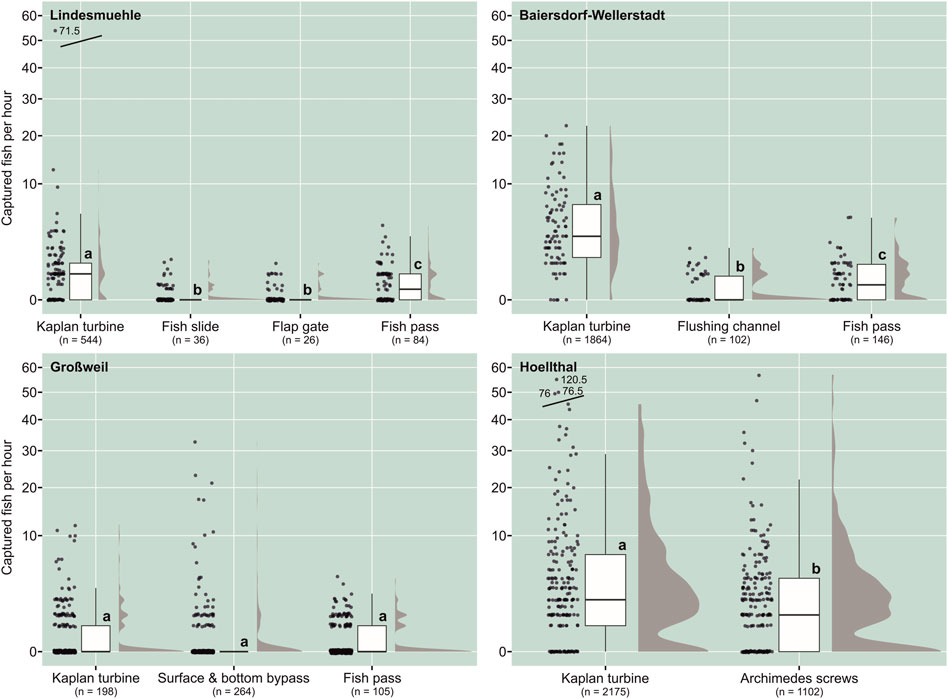
FIGURE 2. Comparison of captured fish per hour that passed the different corridors in downstream direction at the investigated hydropower sites. Different lowercase letters indicate significant differences between corridors (p ≤ 0.05) according to Bonferroni-corrected post hoc pairwise Mann-Whitney U test or Wilcoxon test. Box: 25% quantile, median, 75% quantile; whisker: minimum, maximum values; n = sum of captured fish.
The percentage of fish that passed the turbines was higher at the sites Lindesmuehle (79%) and Baiersdorf-Wellerstadt (88%) with a 15 mm fine screen than at the sites Großweil (35%) and Hoellthal (66%) with a 20 mm fine screen (Table 2). However, the proportion of turbine discharge was also higher in Lindesmuehle (94%) and Baiersdorf-Wellerstadt (98%) than in Großweil (85%) and Hoellthal (56%). The effectiveness of the fish guided away from the turbine corridor towards alternative corridors during their downstream passage was strongly dependent on the diverted water volume (Figure 3). Across all sites, a significant positive correlation between the percentage of corridor discharge and the percentage of captured fish in the respective corridor was found (linear regression: adjusted R2 = 0.71, p < 0.001) (Figure 3).
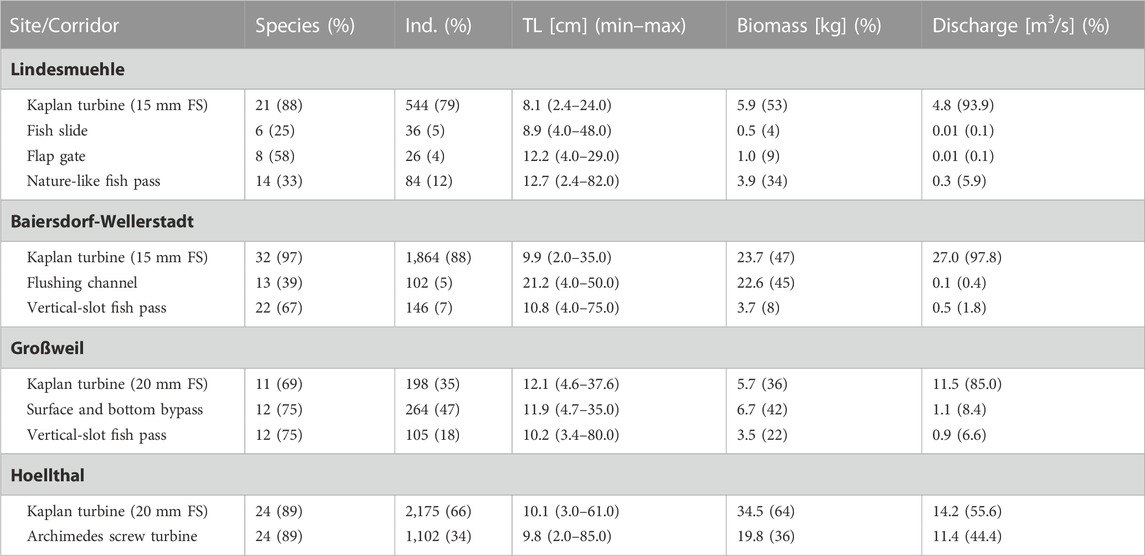
TABLE 2. Number and percentage (in parentheses) of captured fish species and individuals, average total length (TL) and range of TLs (min–max), total biomass and average discharge per corridor at the four investigated hydropower sites. For biomass and discharge, the percentage per corridor is given in parentheses. FS = fine screen.
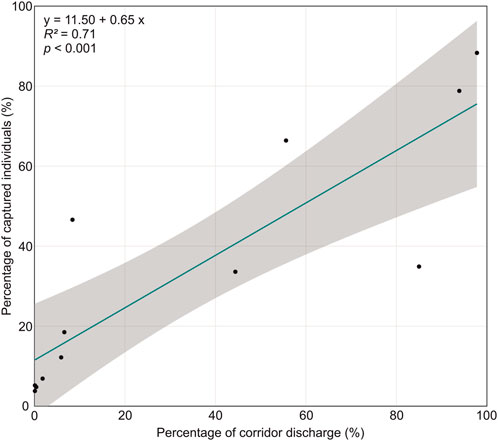
FIGURE 3. Percentage of captured fish per corridor as a function of the percentage of corridor discharge in relation to the total discharge. Function (y), adjusted coefficient of determination (R2) and significance level (p) of the linear regression are presented on the top left. The grey area indicates the 95% confidence interval.
3.2 Comparison of fish sizes between corridors
At Lindesmuehle, TLs of fish caught in the flap gate corridor (a.m.±s.d.: 12.2 ± 6.4 cm) were significantly higher than in the turbine corridor (8.2 ± 4.1 cm) and at the fish slide (8.9 ± 7.3 cm) (Figure 4). Significantly higher TLs of downstream moving fish were also found in the flushing channel corridor (21.2 ± 8.1 cm) at the Baiersdorf-Wellerstadt site compared to the turbine corridor (9.9 ± 4.1 cm) and the fish pass (10.8 ± 7.0 cm) (Figure 4). At Großweil, TLs of fish caught in the fish passes (10.2 ± 8.7 cm) were significantly lower than in the turbine corridor (12.1 ± 4.4 cm) and in the surface- and bottom-near bypasses (11.9 ± 3.7 cm) (Figure 4). A significant difference in TL of downstream moving fish was also found between the Kaplan turbine and the Archimedes screw corridors, with average TLs slightly higher in the Kaplan turbine corridor (10.1 ± 4.8 cm) than at the Archimedes screw turbines (9.8 ± 6.8 cm) (Figure 4; Table 2).
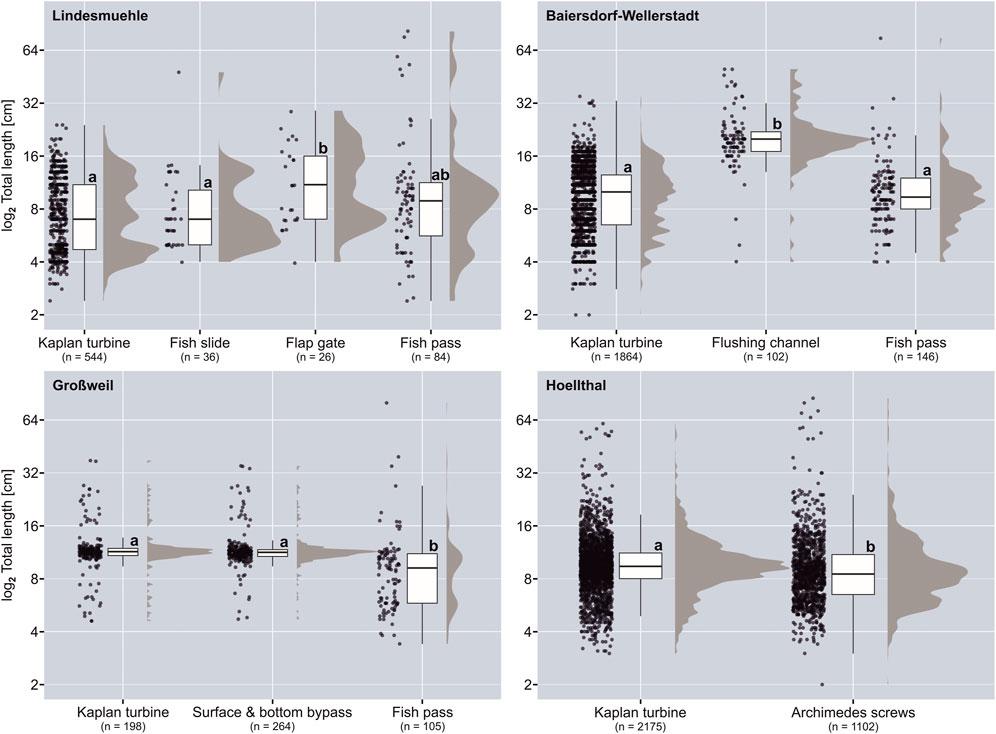
FIGURE 4. Comparison of the total lengths of captured fish that passed the different corridors in downstream direction at the investigated hydropower sites. Different lowercase letters indicate significant differences between corridors (p ≤ 0.05) according to Bonferroni-corrected post hoc pairwise Mann-Whitney U test or Wilcoxon test. Box: 25% quantile, median, 75% quantile; whisker: minimum, maximum values; n = sum of captured fish.
At the sites Lindesmuehle and Baiersdorf-Wellerstadt with 15 mm fine screens, the proportion of fish >20 cm TL using the bypasses for downstream passage was significantly higher than the proportion of fish >20 cm TL passing the turbines (proportion test: X2 = 326.8; p < 0.001). In contrast, no statistically significant difference between the proportion of fish >20 cm TL using the bypasses and the proportion of fish >20 cm TL passing the turbines was detectable for Großweil and Hoellthal with 20 mm fine screens (proportion test: X2 = 2.1; p = 0.15) (Figure 5).
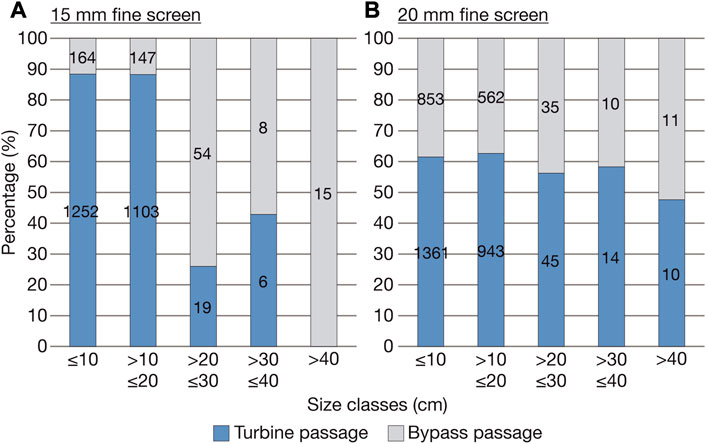
FIGURE 5. Comparison of the percentage of fish by size class that moved downstream via the turbines and the various bypasses. (A) Hydropower sites with 15 mm fine screens in front of the turbine inlet (Lindesmuehle and Baiersdorf-Wellerstadt), (B) hydropower sites with 20 mm fine screens (Großweil and Hoellthal). The sum of captured fish per size class and corridor is shown on the bars.
3.3 Species-specific corridor usage
The most frequently caught species at Lindesmuehle, roach, gudgeon (Gobio gobio L.), ruffe (Gymnocephalus cernua L.), common dace (Leuciscus leuciscus L.) and European perch (Perca fluviatilis L.), predominantly used the turbine corridor for downstream movement. In contrast, the bottom-oriented species bullhead was mainly caught in the fish slide corridor (80% of captured individuals, n = 20). With a share of more than 50% of the total number of individuals, European eel, spirlin and European minnow (Phoxinus phoxinus L.) were more frequently caught in the fish pass than in the corridors turbine, fish slide and flap gate (Figure 6). Similar to Lindesmuehle, the most abundant fish species in Baiersdorf-Wellerstadt—bleak, spirlin, topmouth gudgeon, roach, brown trout (Salmo trutta L.) and common dace—were more frequently caught in the turbine corridor than in the other corridors. It is noticeable that common carp (Cyprinus carpio L.) and Prussian carp (Carassius gibelio Bloch) more frequently moved downstream via the flushing channel than via the turbines and the fish pass (Figure 6). At Großweil, the most abundant species European grayling (Thymallus thymallus L.) was more frequently caught in the surface- and bottom-near bypasses than in the turbine corridor and the fish passes. In contrast, the second and third most abundant species, bullhead and European minnow, were more frequently caught in the fish passes than in the other corridors, accounting for 73% and 72% of the total catch, respectively. It is remarkable that barbel (Barbus barbus L.) was exclusively found in the turbine corridor, although only five barbels were caught (Figure 6). Of the ten most abundant species in Hoellthal, all except bleak, asp (Leuciscus aspius L.) and common dace more frequently moved downstream via the Kaplan turbine corridor than via the Archimedes screw turbines (Figure 6).
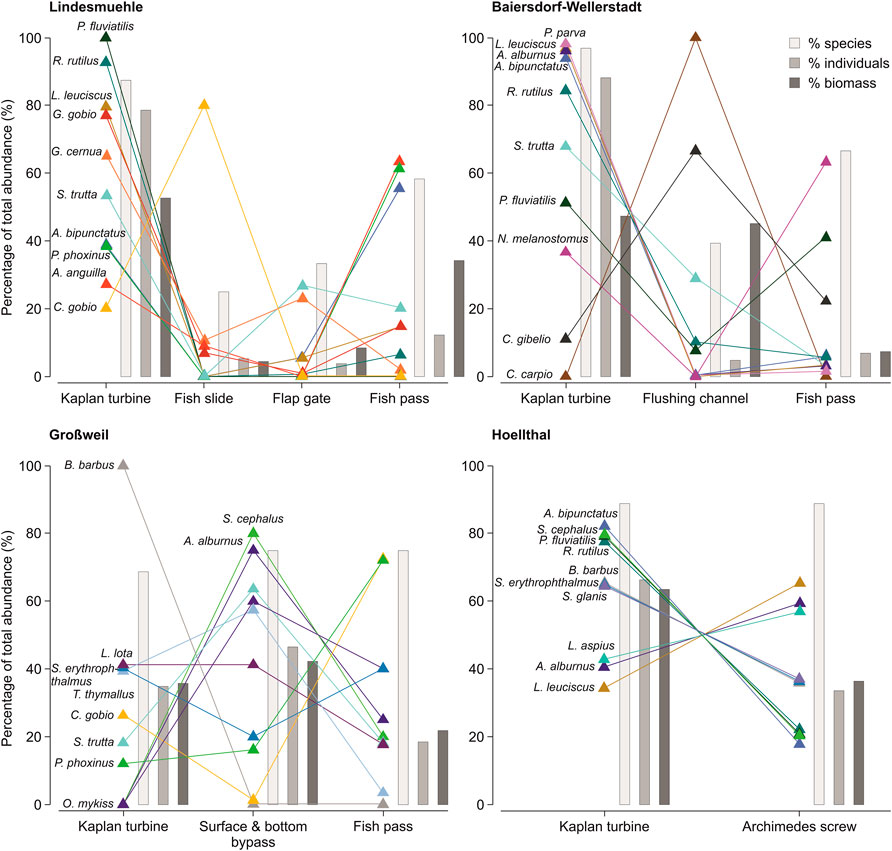
FIGURE 6. Species-specific percentage of total abundance of fish captured in the different downstream corridors of the assessed hydropower plants. For each site, the ten most important species with the highest contribution to the total catch in one or more of the corridors are shown. For each corridor, bars in shades of grey symbolize the percentage of captured species in the total number of species (% species), the percentage of captured individuals in the total number of individuals (% individuals) and the percentage of biomass in the total biomass (% biomass) per site.
Comparing the average abundance of captured fish between turbine corridor and other bypasses, considerably more individuals of the most important species (= species with the highest contribution to the total catch in one or more of the various corridors) passed the HPPs via the turbine corridor (min–max: 1.5–524.7 fish per week) than via the bypasses (2.1–118.6 fish per week), particularly at the sites Lindesmuehle, Baiersdorf-Wellerstadt and Hoellthal (Figure 7A). However, referring to a standardized discharge (= captured fish per 10 million m³ discharge), a contrary pattern was found: Primarily at the sites Lindesmuehle, Baiersdorf-Wellerstadt and Großweil, the discharge-related downstream movement rates via the bypasses (22.4–961.4 fish per 10 million m³ discharge) were mostly clearly higher than via the turbine corridor (2.2–610.8 fish per 10 million m³ discharge) (Figure 7B).
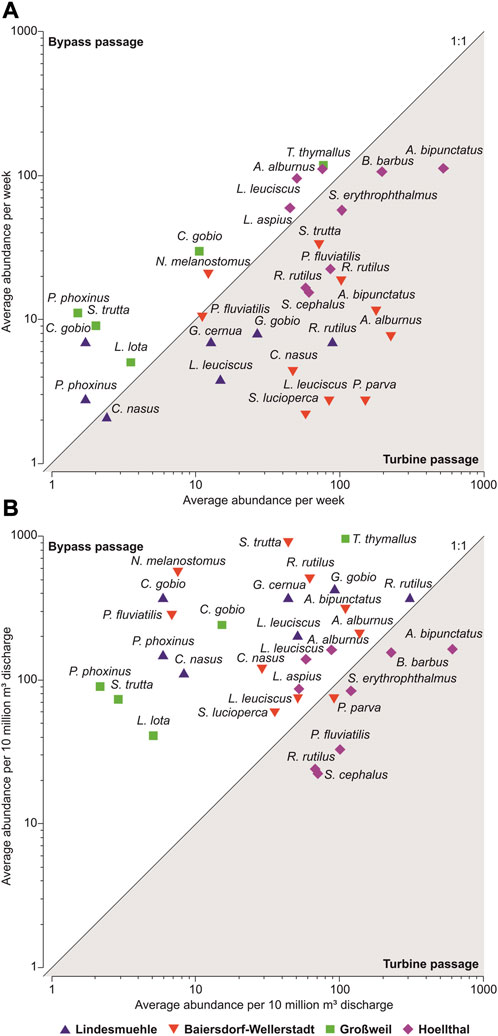
FIGURE 7. Scatterplot of the average abundance of captured downstream moving fish per week (A) and per 10 million m³ discharge (B) at the investigated hydropower sites for species, which contributed ≥3% to the dissimilarity between bypass and turbine passage according to one-way Similarity Percentage analyses (SIMPER).
4 Discussion
For an objective evaluation of the impacts of HPPs on fish welfare, not only fish species-specific mortalities and injury rates, but also the efficiency of fish guiding structures and the actual use of the different downstream migration corridors need to be considered. In the present study, the corridor usage of downstream moving fish was investigated at four small-scale HPPs with different concepts to prevent turbine entrainment and to bypass fish. The results indicate that despite existing bypass systems and fine screens with 15 mm and 20 mm bar spacing installed upstream of the turbine inlets, a large proportion of fish still passed the turbines, albeit at a slightly lower ratio than expected from the proportions of discharge through turbines versus alternative corridors. Physical exclusion effects of the bar screens and behavioral effects due to the arrangement of the fine screens (angled, inclined or horizontal at the river bottom), which are supposed to prevent passage and guide fish to bypasses, appear to be mostly low at the assessed HPPs. However, standardizing the number of downstream moving fish to a specific discharge revealed that the discharge-related downstream movement rates via the bypasses were mainly higher than via the turbine corridor. This suggests that increasing the bypass discharge, e.g., by enlarging the openings, could increase the actual bypass efficiency. Moreover, recent studies suggest that a combination of physical barriers such as bar screens with behavioral barriers (e.g., electric fields) could increase fish protection from turbine entrainment and the guiding effect towards bypasses (cf. Tutzer et al., 2021, 2022; Haug et al., 2022). However, since fish downstream movements can also take place at very low water temperatures in late autumn/winter and reduced metabolic rates potentially increase the proportion of fish drifting passively with the main current (Wiesner et al., 2004), it would be worthwhile for future studies to investigate whether the turbine entrainment risk is increased under these conditions.
It is widely assumed that fish can only pass bar-structured barriers if the body width or height is smaller than the bar spacing (Ebel, 2013; Schwevers and Adam, 2020). However, recent studies have shown that even much larger fish than expected by modelling or rules of thumb can pass through such bar screens (cf. Knott et al., 2023). This is probably due to the fact that fish can also squeeze through barriers and damage to the screen caused by debris or manufacturer-related deviations in bar spacing, which can be found in regular operation under field conditions, allow larger fish than expected to pass. In addition, the poor barrier function of the assessed fine screens may also be explained by the fact that the majority of the fish population in the study rivers consists of small-bodied species and juveniles (cf. Knott et al., 2020), for which the fine screens did not act as a physical barrier. This is also supported by the finding that 96% of the captured fish over all corridors in this study were <20 cm in TL.
In addition to the primary protective effect as a physical barrier, fine screens can also lead to an avoidance reaction or a guiding effect through optical or hydraulic stimuli, whereby physically permeable barriers can also be effective (Ebel, 2013; Harbicht et al., 2022). However, this behavior-influencing effect no longer works at high approach velocities at the screen (≥0.5 m/s according to DWA, 2005). High current velocities at the fine screen can be a reason for the high number of screen and turbine passages, especially in Baiersdorf-Wellerstadt, where the threshold value of 0.5 m/s was permanently exceeded. As a result, behavior-influencing effects that could also keep smaller individuals from passing the screen or guide them towards bypasses no longer apply. Moreover, fish can be injured or killed if the current velocities at the screen are too high, as they can no longer actively avoid the screen, but are pressed against or between the bars (Deng et al., 2010; Brown et al., 2014; Amaral et al., 2018).
The low proportion of fish that moved downstream via the bypasses located in the immediate vicinity of the fine screens in Lindesmuehle (fish slide and flap gate) and Baiersdorf-Wellerstadt (flushing channel) suggests that the guiding effect towards these corridors was low.
In general, bypass efficiency is, besides proper location and hydraulic conditions at the entrance, very much dependent on the available discharge. The higher the discharge, the more fish typically enter a bypass (Turnpenny et al., 1998) which is also supported by the findings of this study. Commonly, a minimum of 2% of turbine discharge is recommended for “good” bypass efficiency (Klopries et al., 2018: 2%–35%; Larinier and Travade, 2002: 2%–10%; Odeh and Orvis, 1998: 2%–5%). Bypass discharge via the fish slide and the flap gate in Lindesmuehle each amounted to 0.1% of the turbine discharge and via the flushing channel in Baiersdorf-Wellerstadt to 0.4% of the turbine discharge and was thus well below these recommendations. However, “good” bypass efficiency can only be achieved at low discharges if guiding structures, location and hydraulic conditions are ideal (Klopries et al., 2018). In addition to insufficient discharge, the fish slide and the flap gate in Lindesmuehle probably also have deficits in terms of detectability for fish. The fish slide was predominantly used by bottom-oriented species such as bullhead, ruffe and European eel, indicating that the bottom entrances were preferentially used over the near-surface opening. Results from Egg et al. (2017) also suggest that the functionality of the bottom entrances is reduced particularly in late autumn, as they can become clogged by leaf debris that is mobilized during increased flows. The low bypass efficiency of the flap gate in Lindesmuehle can further be explained by a potentially reduced detectability for fish due to the periodical operation compared to permanently opened corridors. In addition, the periodic bypass opening causes an immediate change in hydraulic conditions (e.g., during the screen cleaning and flushing process; cf. Williams et al., 2012), which can scare fish away (Schwevers and Adam, 2020). Moreover, an unfavorable bypass location may not only reduce detectability, but also lead to an increased predation or impingement risk (Turnpenny et al., 1998).
Deficits for fish to enter the flushing channel in Baiersdorf-Wellerstadt seemed to be the low overflow height of the downstream end of the vertically inclined fine screen (<5 cm) during the study period, which made it difficult for larger fish to get into the flushing channel. Nevertheless, considerably larger fish moved downstream via the flushing channel at this site than via the turbines and the fish pass. It was observed that larger individuals of common carp and Prussian carp were impinged at the 15 mm fine screen and transported into the flushing channel by the screen cleaner. These individuals were physically unable to pass the fine screen and probably could not escape the high approach velocities at the screen (0.97 m/s on average).
It is remarkable that in Lindesmuehle and Baiersdorf-Wellerstadt more fish moved downstream via the fish passes than via other bypasses. This can be explained by the considerably higher discharge through the fish passes compared to the other bypasses, whereby the upstream entrance of the fish pass in Lindesmuehle was about 70 m away from the HPP on the opposite bank (Figure 1) and its entrance was therefore expected to not be easily discovered by downstream moving fish. However, due to the applied fish catching method, it was not possible to differentiate whether the captured fish moved downstream from the headwater or used the fish passes as habitat before moving downstream. Particularly for small-bodied fish species such as spirlin, bullhead and European minnow and juvenile fish, fish passes not only serve as a migration corridor, but also as a suitable habitat (Pander et al., 2018).
Großweil was the only site where more fish moved downstream via the surface- and bottom-near bypasses than via the turbine corridor. In addition to a considerably higher share of bypass discharge of 9.9% of the turbine discharge compared to Lindesmuehle (0.2%) and Baiersdorf-Wellerstadt (0.4%), site-specific characteristics also seem to have affected the corridor usage of downstream moving fish. For example, this finding was influenced by a dominance of European grayling (68% of all captured fish at this site with TLs mostly ranging from 10 to 13 cm), which is an open water-oriented species that prefers surface-near corridors for downstream migration (Knott et al., 2019).
At Hoellthal, similar to Lindesmuehle and Baiersdorf-Wellerstadt, most of the captured fish moved downstream via the Kaplan turbine corridor. The lack of permanently open bypasses in spatial proximity to the HPP and approach velocities >0.5 m/s may have influenced the high number of screen passages. About one-third of the captured fish at this site passed the Archimedes screw turbines, where fish protection measures upstream of the turbine inlet are deliberately not in place. Although Archimedes screw turbines are often referred to as “fish-friendly” due to low runner speeds and pressure changes (Nuernbergk and Rorres, 2013; Simmons and Lubitz, 2021), recent studies have shown that this technique can indeed cause severe injuries and mortality, depending on the fish species in question and the site-specific conditions (e.g., Pauwels et al., 2020; Mueller et al., 2022).
5 Conclusion
Despite existing fish protection screens and bypasses, a large proportion of fish passed the turbine corridor during their downstream migration. Physical exclusion screens with 15 mm and 20 mm bar spacing, which are also supposed to have a behavior-influencing effect through various inclinations and guide fish to bypasses, could not prevent the majority of fish from passing the hazardous turbine corridor. The mainly poor effectiveness of the investigated bypasses was probably due to the fact that the discharge was too low and the location or detectability of the bypasses was unfavorable, reflecting the real operational conditions at these sites. The different bypass types were used by a different range of fish species and sizes which was also strongly site-dependent. This highlights the need to check existing bypasses not only for compliance with current requirements in terms of design, dimensioning and spatial location to the HPP, but also in relation to the site-specific fish community. Bypasses should ideally function for the widest possible range of fish species present in a system. This could be achieved through either less selective bypass types such as optimally positioned full-depth bypasses, or a combination of different bypass systems. Moreover, less harmful turbine technologies must be developed and identified in order to enhance fish protection from HPP-related injuries and mortality during downstream passage, since fine screens with 15 mm and 20 mm bar spacing cannot prevent small-bodied fish species and juvenile fish <20 cm from turbine entrainment.
Data availability statement
The raw data supporting the conclusion of this article will be made available by the authors, without undue reservation.
Ethics statement
The study was conducted within the framework of an approved animal experiment, with permission from the Bavarian Government (permit numbers ROB-55.2-2532.Vet_02-15-31 and ROB-55.2-2532.Vet_02-19-160). All required measures have been taken to prevent unnecessary stress of fish according to European laws (European Parliament, 2010) and national standards (Adam et al., 2013).
Author contributions
JK, MM, JP, and JG conceived the ideas and designed methodology; JK, MM, and JP collected the data; JK analyzed the data and led the writing of the manuscript. All authors contributed critically to the drafts and gave final approval for publication.
Funding
This work was supported by the Bavarian State Ministry of the Environment and Consumer Protection (grant numbers OelB-0270-45821/2014, OeIB-0270-88607/2018).
Acknowledgments
We are grateful to the Bavarian Environmental Agency, the HPP owners, the fisheries rights owners, the fisheries authorities and all field volunteers for their support.
Conflict of interest
The authors declare that the research was conducted in the absence of any commercial or financial relationships that could be construed as a potential conflict of interest.
Publisher’s note
All claims expressed in this article are solely those of the authors and do not necessarily represent those of their affiliated organizations, or those of the publisher, the editors and the reviewers. Any product that may be evaluated in this article, or claim that may be made by its manufacturer, is not guaranteed or endorsed by the publisher.
Supplementary material
The Supplementary Material for this article can be found online at: https://www.frontiersin.org/articles/10.3389/fenvs.2023.1168473/full#supplementary-material
References
Adam, B., Schürmann, M., and Schwevers, U. (2013). Zum Umgang mit aquatischen Organismen: Versuchstierkundliche Grundlagen. Wiesbaden: Springer Spektrum. doi:10.1007/978-3-658-01538-1
Algera, D. A., Rytwinski, T., Taylor, J. J., Bennett, J. R., Smokorowski, K. E., Harrison, P. M., et al. (2020). What are the relative risks of mortality and injury for fish during downstream passage at hydroelectric dams in temperate regions? A systematic review. Environ. Evid. 9, 3. doi:10.1186/s13750-020-0184-0
Allen, M., Poggiali, D., Whitaker, K., Marshall, T. R., van Langen, J., and Kievit, R. A. (2021). Raincloud plots: A multi-platform tool for robust data visualization. Wellcome Open Res. 4, 63. doi:10.12688/wellcomeopenres.15191.2
Amaral, S. V., Coleman, B. S., Rackovan, J. L., Withers, K., and Mater, B. (2018). Survival of fish passing downstream at a small hydropower facility. Mar. Freshw. Res. 69 (12), 1870–1881. doi:10.1071/MF18123
Anderson, D., Moggridge, H., Warren, P., and Shucksmith, J. (2015). The impacts of ‘run-of-river’ hydropower on the physical and ecological condition of rivers. Water Environ. J. 29 (2), 268–276. doi:10.1111/wej.12101
Antonio, R. R., Agostinho, A. A., Pelicice, F. M., Bailly, D., Okada, E. K., and Dias, J. H. P. (2007). Blockage of migration routes by dam construction: Can migratory fish find alternative routes? Neotrop. Ichthyol. 5 (2), 177–184. doi:10.1590/S1679-62252007000200012
Banks, J. W. (1969). A review of the literature on the upstream migration of adult salmonids. J. Fish. Biol. 1 (2), 85–136. doi:10.1111/j.1095-8649.1969.tb03847.x
Brown, R. S., Colotelo, A. H., Pflugrath, B. D., Boys, C. A., Baumgartner, L. J., Deng, Z. D., et al. (2014). Understanding barotrauma in fish passing hydro structures: A global strategy for sustainable development of water resources. Fisheries 39 (3), 108–122. doi:10.1080/03632415.2014.883570
Clarke, K. R., Gorley, R. N., Somerfield, P. J., and Warwick, R. M. (2014). Change in marine communities: An approach to statistical analysis and interpretation. 3rd ed. Plymouth, UK: PRIMER-E Ltd.
Deng, Z., Carlson, T. J., Duncan, J. P., Richmond, M. C., and Dauble, D. D. (2010). Use of an autonomous sensor to evaluate the biological performance of the advanced turbine at Wanapum Dam. J. Renew. Sustain. Energy 2 (5), 053104. doi:10.1063/1.3501336
Dugan, P. J., Barlow, C., Agostinho, A. A., Baran, E., Cada, G. F., Chen, D., et al. (2010). Fish migration, dams, and loss of ecosystem services in the mekong basin. AMBIO 39, 344–348. doi:10.1007/s13280-010-0036-1
DWA (Deutsche Vereinigung für Wasserwirtschaft, Abwasser und Abfall e.V.) (2005). Fischschutz- und Fischabstiegsanlagen: Bemessung, Gestaltung, Funktionskontrolle. Hennef: DWA Themen.
Ebel, G. (2013). Fischschutz und Fischabstieg an Wasserkraftanlagen – handbuch Rechen-und Bypasssysteme. Ingenieurbiologische Grundlagen, Modellierung und Prognose, Bemessung und Gestaltung (1. Auflage). Halle (Saale): Büro für Gewässerökologie und Fischereibiologie Dr. Ebel.
Egg, L., Mueller, M., Pander, J., Knott, J., and Geist, J. (2017). Improving European Silver Eel (Anguilla anguilla) downstream migration by undershot sluice gate management at a small-scale hydropower plant. Ecol. Eng. 106, 349–357. doi:10.1016/j.ecoleng.2017.05.054
European Commission (1992). Council Directive 92/43/EEC of 21 May 1992 on the conservation of natural habitats and of wild fauna and flora. Off. J. Eur. Union 206, 7–50.
European Parliament (2010). Directive 2010/63/EU of the European Parliament and of the Council of 22 September 2010 on the protection of animals used for scientific purposes. Off. J. Eur. Union 276, 33–77.
Evans, W. A., and Johnston, B. (1980). Fish migration and fish passage: A practical guide to solving fish passage problems. Washington D.C.: US Forest Service.
Fjeldstad, H. P., Pulg, U., and Forseth, T. (2018). Safe two-way migration for salmonids and eel past hydropower structures in Europe: A review and recommendations for best-practice solutions. Mar. Freshw. Res. 69 (12), 1834–1847. doi:10.1071/MF18120
Fukushima, M., Kameyama, S., Kaneko, M., Nakao, K., and Ashley Steel, E. (2007). Modelling the effects of dams on freshwater fish distributions in Hokkaido, Japan. Freshw. Biol. 52 (8), 1511–1524. doi:10.1111/j.1365-2427.2007.01783.x
Gehrke, P. C., Gilligan, D. M., and Barwick, M. (2002). Changes in fish communities of the shoalhaven river 20 years after construction of tallowa dam, Australia. River Res. Appl. 18, 265–286. doi:10.1002/rra.669
Geist, J. (2021). Editorial: Green or red: Challenges for fish and freshwater biodiversity conservation related to hydropower. Aquat. Conserv. 31 (7), 1551–1558. doi:10.1002/aqc.3597
Gosset, C., Travade, F., Durif, C., Rives, J., and Elie, P. (2005). Tests of two types of bypass for downstream migration of eels at a small hydroelectric power plant. River Res. Appl. 21, 1095–1105. doi:10.1002/rra.871
Harbicht, A. B., Watz, J., Nyqvist, D., Virmaja, T., Carlsson, N., Aldvén, D., et al. (2022). Guiding migrating salmonid smolts: Experimentally assessing the performance of angled and inclined screens with varying gap widths. Ecol. Eng. 174, 106438. doi:10.1016/j.ecoleng.2021.106438
Hassinger, R., and Huebner, D. (2009). Entwicklung eines neuartigen Aal-Abstiegsystems mithilfe von Laborversuchen. KW Korresp. Wasserwirtsch. 2, 276–281.
Haug, J., Auer, S., Frees, C., Brinkmeier, B., Tutzer, R., Hayes, D. S., et al. (2022). Retrofitting of existing bar racks with electrodes for fish protection—an experimental study assessing the effectiveness for a pilot site. Water 14 (6), 850. doi:10.3390/w14060850
Havn, T. B., Sæther, S. A., Thorstad, E. B., Teichert, M. A. K., Heermann, L., Diserud, O. H., et al. (2017). Downstream migration of Atlantic salmon smolts past a low head hydropower station equippped with Archimedes screw and Francis turbines. Ecol. Eng. 105, 262–275. doi:10.1016/j.ecoleng.2017.04.043
Jonsson, N. (1991). Influence of water flow, water temperature and light on fish migration in rivers. Nord. J. Freshw. Res. 66, 20–35.
Katopodis, C. (2005). Developing a toolkit for fish passage, ecological flow management and fish habitat works. J. Hydraul. Res. 43 (5), 451–467. doi:10.1080/00221680509500144
Klopries, E. M., Deng, Z. D., Lachmann, T. U., Schüttrumpf, H., and Trumbo, B. A. (2018). Surface bypass as a means of protecting downstream-migrating fish: Lack of standardised evaluation criteria complicates evaluation of efficacy. Mar. Freshw. Res. 69 (12), 1882–1893. doi:10.1071/MF18097
Knott, J., Mueller, M., Pander, J., and Geist, J. (2023). Bigger than expected: Species-and size-specific passage of fish through hydropower screens. Ecol. Eng. 188, 106883. doi:10.1016/j.ecoleng.2022.106883
Knott, J., Mueller, M., Pander, J., and Geist, J. (2019). Fish passage and injury risk at a surface bypass of a small-scale hydropower plant. Sustainability 11 (21), 6037. doi:10.3390/su11216037
Knott, J., Mueller, M., Pander, J., and Geist, J. (2020). Seasonal and diurnal variation of downstream fish movement at four small-scale hydropower plants. Ecol. Freshw. Fish. 29 (1), 74–88. doi:10.1111/eff.12489
Larinier, M., and Travade, F. (2002). Downstream migration: Problems and facilities. Bull. Fr. Pêche Piscic. 364, 181–207. doi:10.1051/kmae/2002102
Liermann, C. R., Nilsson, C., Robertson, J., and Ng, R. Y. (2012). Implications of dam obstruction for global freshwater fish diversity. BioScience 62 (6), 539–548. doi:10.1525/bio.2012.62.6.5
Lucas, M. C., and Baras, E. (2001). Migration of freshwater fishes. Oxford: Blackwell Science. doi:10.1002/9780470999653
Mueller, M., Knott, J., Pander, J., and Geist, J. (2022). Experimental comparison of fish mortality and injuries at innovative and conventional small hydropower plants. J. Appl. Ecol. 59 (9), 2360–2372. doi:10.1111/1365-2664.14236
Noatch, M. R., and Suski, C. D. (2012). Non-physical barriers to deter fish movements. Environ. Rev. 20 (1), 71–82. doi:10.1139/a2012-001
Nuernbergk, D. M., and Rorres, C. (2013). Analytical model for water inflow of an Archimedes screw used in hydropower generation. J. Hydraul. Eng. 139 (2), 213–220. doi:10.1061/(ASCE)HY.1943-7900.0000661
Nyqvist, D., Elghagen, J., Heiss, M., and Calles, O. (2018). An angled rack with a bypass and a nature-like fishway pass Atlantic salmon smolts downstream at a hydropower dam. Mar. Freshw. Res. 69 (12), 1894–1904. doi:10.1071/MF18065
Odeh, M., and Orvis, C. (1998). “Downstream fish passage considerations and developments at hydroelectric projects in North-east USA,” in Fish migration and fish bypasses. Editors M. Jungwirth, S. Schmutz, and S. Weiss (Oxford, Blackwell: Fishing News Books), 267–280.
Pander, J., Mueller, M., Knott, J., and Geist, J. (2018). Catch-related fish injury and catch efficiency of stow-net-based fish recovery installations for fish-monitoring at hydropower plants. Fish. Manag. Ecol. 25, 31–43. doi:10.1111/fme.12263
Pauwels, I. S., Baeyens, R., Toming, G., Schneider, M., Buysse, D., Coeck, J., et al. (2020). Multi-species assessment of injury, mortality, and physical conditions during downstream passage through a large archimedes hydrodynamic screw (Albert canal, Belgium). Sustainability 12 (20), 8722. doi:10.3390/su12208722
Pelicice, F. M., Pompeu, P. S., and Agostinho, A. A. (2015). Large reservoirs as ecological barriers to downstream movements of Neotropical migratory fish. Fish. Fish. 16 (4), 697–715. doi:10.1111/faf.12089
Pracheil, B. M., DeRolph, C. R., Schramm, M. P., and Bevelhimer, M. S. (2016). A fish-eye view of riverine hydropower systems: The current understanding of the biological response to turbine passage. Rev. Fish. Biol. Fish. 26 (2), 153–167. doi:10.1007/s11160-015-9416-8
R Core Team (2022). R: A language and environment for statistical computing. Vienna, Austria: R Foundation for Statistical Computing.
Schilt, C. R. (2007). Developing fish passage and protection at hydropower dams. Appl. Anim. Behav. Sci. 104, 295–325. doi:10.1016/j.applanim.2006.09.004
Schwevers, U., and Adam, B. (2020). Fish protection technologies and fish ways for downstream migration. Cham, Switzerland: Springer Nature. doi:10.1007/978-3-030-19242-6
Scruton, D. A., Pennell, C. J., Bourgeois, C. E., Goosney, R. F., Porter, T. R., and Clarke, K. D. (2007). Assessment of a retrofitted downstream fish bypass system for wild Atlantic salmon (Salmo salar) smolts and kelts at a hydroelectric facility on the Exploits River, Newfoundland, Canada. Hydrobiologia 582, 155–169. doi:10.1007/s10750-006-0557-6
Simmons, S. C., and Lubitz, W. D. (2021). Archimedes screw generators for sustainable micro-hydropower production. Int. J. Energy Res. 45 (12), 17480–17501. doi:10.1002/er.6893
Travade, F., Larinier, M., Subra, S., Gomes, P., and De-Oliveira, E. (2010). Behaviour and passage of European silver eels (Anguilla anguilla) at a small hydropower plant during their downstream migration. Knowl. Manag. Aquat. Ecosyst. 398, 01–19. doi:10.1051/kmae/2010022
Turnpenny, A. W., Struthers, G., and Hanson, P. (1998) A UK guide to intake fish-screening regulations, policy and best practice with particular reference to hydroelectric power schemes. Technical Report ETSU-H-06-00052/REP. Fawley Aquatic Research Laboratories Ltd. and Hydroplan.
Tutzer, R., Röck, S., Walde, J., Haug, J., Brinkmeier, B., Aufleger, M., et al. (2022). A physical and behavioral barrier for enhancing fish downstream migration at hydropower dams: The flexible FishProtector. Water 14 (3), 378. doi:10.3390/w14030378
Tutzer, R., Röck, S., Walde, J., Zeiringer, B., Unfer, G., Führer, S., et al. (2021). Ethohydraulic experiments on the fish protection potential of the hybrid system FishProtector at hydropower plants. Ecol. Eng. 171, 106370. doi:10.1016/j.ecoleng.2021.106370
Wickham, H. (2016). ggplot2: Elegant graphics for data analysis. New York: Springer Nature. doi:10.1007/978-3-319-24277-4
Wiesner, C., Unfer, G., Zitek, A., and Schmutz, S. (2004). “Drift of juvenile freshwater fish in late autumn in a Danube tributary,” in Proceedings of the Fifth International Conference on Ecohydraulics – Aquatic Habitats: Analysis and Restoration, Madrid, 12.–17.09.2004.
Keywords: downstream migration, fish behavior, fish bypass, fish guiding structure, fish passage, river fragmentation, sustainable hydropower, turbine entrainment
Citation: Knott J, Mueller M, Pander J and Geist J (2023) Downstream fish passage at small-scale hydropower plants: Turbine or bypass?. Front. Environ. Sci. 11:1168473. doi: 10.3389/fenvs.2023.1168473
Received: 17 February 2023; Accepted: 16 March 2023;
Published: 24 March 2023.
Edited by:
Thomas Hein, University of Natural Resources and Life Sciences Vienna, AustriaReviewed by:
Günther Unfer, University of Natural Resources and Life Sciences Vienna, AustriaSergio Makrakis, Western Parana State University, Brazil
Copyright © 2023 Knott, Mueller, Pander and Geist. This is an open-access article distributed under the terms of the Creative Commons Attribution License (CC BY). The use, distribution or reproduction in other forums is permitted, provided the original author(s) and the copyright owner(s) are credited and that the original publication in this journal is cited, in accordance with accepted academic practice. No use, distribution or reproduction is permitted which does not comply with these terms.
*Correspondence: Juergen Geist, geist@tum.de