- Rynda-Apple Laboratory, Department of Microbiology and Immunology, Montana State University, Bozeman, MT, United States
Influenza virus infections particularly when followed by bacterial superinfections (BSI) result in significant morbidities and mortalities especially during influenza pandemics. Type I interferons (IFNs) regulate both anti-influenza immunity and host susceptibility to subsequent BSIs. These type I IFNs consisting of, among others, 14 IFN-α's and a single IFN-β, are recognized by and signal through the heterodimeric type I IFN receptor (IFNAR) comprised of IFNAR1 and IFNAR2. However, the individual receptor subunits can bind IFN-β or IFN-α's independently of each other and induce distinct signaling. The role of type I IFN signaling in regulating host susceptibility to both viral infections and BSI has been only examined with respect to IFNAR1 deficiency. Here, we demonstrate that despite some redundancies, IFNAR1 and IFNAR2 have distinct roles in regulating both anti-influenza A virus (IAV) immunity and in shaping host susceptibility to subsequent BSI caused by S. aureus. We found IFNAR2 to be critical for anti-viral immunity. In contrast to Ifnar1−/− mice, IAV-infected Ifnar2−/− mice displayed both increased and accelerated morbidity and mortality compared to WT mice. Furthermore, unlike IFNAR1, IFNAR2 was sufficient to generate protection from lethal IAV infection when stimulated with IFN-β. With regards to BSI, unlike what we found previously in Ifnar1−/− mice, Ifnar2−/− mice were not susceptible to BSI induced on day 3 post-IAV, even though absence of IFNAR2 resulted in increased viral burden and an increased inflammatory environment. The Ifnar2−/− mice similar to what we previously found in Ifnar1−/− mice were less susceptible than WT mice to BSI induced on day 7 post-IAV, indicating that signaling through a complete receptor increases BSI susceptibility late during clinical IAV infection. Thus, our results support a role for IFNAR2 in induction of anti-IAV immune responses that are involved in altering host susceptibility to BSI and are essential for decreasing the morbidity and mortality associated with IAV infection. These results begin to elucidate some of the mechanisms involved in how the individual IFNAR subunits shape the anti-viral immune response. Moreover, our results highlight the importance of examining the contributions of entire receptors, as individual subunits can induce distinct outcomes as shown here.
Introduction
Influenza A virus (IAV) causes one of the most common respiratory infections worldwide. While infection from IAV can be detrimental on its own, more typically, anti-viral immune responses induced during IAV infection alter host susceptibility to bacterial superinfections (BSI). Over the past decades, Staphylococcus aureus has become one of the predominant bacteria involved in BSIs leading to increased morbidity and mortality, especially during IAV pandemics (1–3). A better understanding of the anti-viral immune factors that are involved in altering the host susceptibility to BSI is essential for decreasing the morbidity and mortality associated with post-IAV BSI.
It is well-known that type I IFNs, including a single IFN-β and 14 IFN-α's, have an established role in the anti-viral immunity to influenza and are known to regulate host susceptibility to the subsequent BSIs. Because type I IFNs are thought to require recognition by the heterodimeric IFNAR1/IFNAR2 receptor, depletion of the IFNAR1 subunit (Ifnar1−/−) is assumed to render mice unresponsive to type I IFN signaling. However, previous studies on the role of IFNAR1 in influenza infection and post-influenza BSI models showed conflicting results. Absence of IFNAR1 either in knockout mice or by antibody blockage resulted in a range of responses. These responses varied from increased mouse susceptibility to infection (4–7), no significant antiviral effect (8), no effect on survival from influenza disease (9), or reduced susceptibility by the means of better controlled inflammation (10). Recent reports have also demonstrated that IFN-β can ligate to either IFNAR1 or IFNAR2 individually, while IFN-α appeared to only ligate to IFNAR2, and that the two different IFN-β/IFNAR subunit complexes can transduce expression of distinct sets of genes (11). Additional evidence for the existence of differential signaling by IFNAR1 and IFNAR2 is supported by an absence of sepsis morbidity in Ifnar1−/− mice compared to high morbidity in Ifnar2−/− and WT mice. These conflicting results prompted us to investigate whether the individual IFNAR subunits are sufficient for interferon signaling, and whether they have non-redundant roles in mediating host immunity to IAV infections and subsequent BSIs. Here, we report that despite some redundancies, IFNAR1 and IFNAR2 have distinct roles in regulating both anti-IAV immunity and in shaping host susceptibility to a subsequent S. aureus BSI. Importantly, these results highlight the need to understand the contribution of individual IFNAR subunits to the infections mediated by either virus or bacteria alone, or together in the context of BSI.
Materials and Methods
Mice
Male and female wild type (WT) C57BL/6 (CD45.2), Ifnar1−/−, and Ifnar2−/− (Ifnar2tm1(KOMP)Vlcg)mice (originally purchased from Jackson Laboratories or UC Davis KOMP Repository) were bred and maintained at Montana State University (Bozeman, MT) Animal Resources Center under pathogen-free conditions. All mice used in this study were between 6 and 8 weeks of age at the initiation of experiment. Mice were weighed and monitored for signs of morbidity and mortality. All care and procedures were in accordance with the recommendations of the NIH, the USDA, and the Guide for the Care and Use of Laboratory Animals (8th ed.) (12). Animal protocols were reviewed and approved by the MSU Institutional Animal Care and Use Committee (IACUC). MSU is accredited by the Association for Assessment and Accreditation of Laboratory Animal Care (AAALAC; number 713).
Inoculations and Challenge
Nonsurgical intratracheal (i.t.) inoculations were performed as described previously for all inoculations and challenges (13). Virus inoculation: mice were inoculated with 100 μL of PBS or 0.1LD50 (1,500 plaque forming units [PFU]) of IAV strain A/PR8/8/34 (PR8; H1N1). Bacterial challenge: mice were inoculated with 100 μL of PBS or 108 CFU of LAC strain of S. aureus (MRSA pulsed-field type USA300; was a kind gift from Jovanka Voyich at MSU). Our previously described procedure for determining CFUs (13) and PFUs (14) was followed on lung homogenate samples. Phosphorylation of STAT3 (P-STAT3) inhibition: mice were inoculated 6 h after IAV infection with 100 μL containing 100 μg of FLLL32 [Cayman Chemical, (15)] in 10% DMSO. Control mice were inoculated with equal volume of PBS with 10% DMSO. Mouse recombinant IFN inoculation (mrIFN): mice were inoculated with either 104 IU mrIFN-β (PBLassay bioscience) or with 2.5 × 105 IU mrIFN-αA in 100 μL PBS (BioLegend) at 0, 3, and 6 h post-IAV infection.
Preparation of BALF Samples and Cytokine Analyses
Mice were sacrificed by intraperitoneal (i.p.) administration of 90 mg/kg of body weight sodium pentobarbital. Bronchoalveolar lavage (BAL) was performed by washing the lungs with 3 mM EDTA in PBS (16) and cellular composition was determined by hemocytometer cell counts and differential counts of cytospins after staining with Quick-Diff solution (Siemens; Medical Solutions Diagnostics, Tarrytown, NY). Cell-free BAL fluid (BALF) was used to determine levels of IL-13 (4–500 pg/ml) and IFN-α (31.3–20.00 pg/ml) using ELISA kits (Ready-SET-Go; eBioscience, San Diego, CA), and levels of IL-1α (Minimal Detectable Concentration (MDC); 1.3 pg/mL), IFNγ (MDC; 0.8 pg/mL), TNFα (MDC; 1.9 pg/mL), IL-1β (MDC; 2.8 pg/mL), IL-10 (MDC; 2.1 pg/mL), IL-6 (MDC; 0.9 pg/mL), IFN-β (MDC; 4 pg/mL) using the LEGENDplex mouse inflammation panel (BioLegend, San Diego, CA). LEGENDplex panel was acquired on LSRII running FACS-Diva software (both obtained from BD Bioscience) and analyzed using BioLegend data analysis software. Results are from ≥4 mice per group (biological replicates) and 2 technical replicates per mouse.
qRT-PCR
Mice were inoculated with IAV or PBS as described above and euthanized 24 h after inoculation. Lungs were homogenized and RNA was extracted immediately using Trizol reagent and chloroform method per manufacturer's protocol. RNA was reverse transcribed with QuantiTect reverse transcription kit (Qiagen, USA). Primers for all murine genes of interest were designed, unless denoted, with PrimerQuest (IDT) and all were manufactured by IDT, USA. Sequences are:
STAT3 (17) Fwd: GGATCGCTGAGGTACAACCC
STAT3 Rev: GTCAGGGGTCTCGACTGTCT
STAT6 (18) Fwd: TCTCCACGAGCTTCACATTG
STAT6 Rev: GACCACCAAGGGCAGAGAC
STAT1 Fwd: GACCCTAAGCGAACTGGATAC
STAT1 Rev: TGTCGCCAGAGAGAAATTCGTGT
STAT2 Fwd: CGGCCAACAGGTGAAATTAAG
STAT2 Rev: GGGACTTACAAAGGAGCAGAA
IRF3 Fwd: CCCACAGTGCTACTGATACC
IRF3 Rev: GTCACACCAGACTTAGGAATGT
IRF7 Fwd: TATGCAAGGCATACCTGGAG
IRF7 Rev: CGATGTCTTCGTAGAGACTGTT
rpl13a fwd: CTCTGGAGGAGAAACGGAAGGAAA, rev: GGTCTTGAGGACCTCTGTGAACTT. All reactions were performed on Roche LightCycler 96 real-time PCR detection system with iTaq universal SYBR green supermix (Bio-Rad, Hercules, CA). The ΔΔCt method was used to assess changes in mRNA abundance, using rpl13a as the housekeeping gene. Results presented are the mean and standard deviation from three biological and three experimental replicates.
Survival, Morbidity, LDH, and Albumin
Mice were weighed on a daily basis and assessed for signs of morbidity and mortality. Morbidity measures were as follow: 0, normal; 1, hunched back or ruffled fur; 2, both hunched back and ruffled fur; 3, not moving over a 5 min period. Lactate dehydrogenase (LDH) in the cell-free BALF was measured using the CytoTox 96® Non-Radioactive Cytotoxicity Assay (Promega) and albumin in the cell-free BALF was measured using QuantiChrom BCG Albumin Assay Kit (BioAssay Systems) following the manufacturers protocols.
Primary Alveolar Epithelial Cell Harvest
After mice were euthanized, their lungs were perfused with PBS containing gentamycin (10 μg/mL) injected into the heart. Lungs were then lavaged with PBS containing 3 mM EDTA, filled with 1 mL PBS containing Dispase (50 units/mL) and Elastase (5 units/mL) to degrade extracellular matrix proteins and tied off. Lungs were incubated at 37°C while shaking for 1 h and following incubation elastase activity was terminated by addition of fetal bovine serum (FBS) and Dnase (4 units/mL) was also added to the lung homogenate samples to ensure degradation of extracellular DNA. The lungs were then dissected and aspirated through syringes to create a single cell suspension. The cell suspension was filtered (100 and 50 μm) and separated by a discontinuous Percoll gradient consisting of a heavy layer (5.2 mL Percoll, 50 μL FBS, 3.8 mL water, 1 mL 10 × PBS/100 mM HEPES/55 mM Glucose/pH 7.4) and a light layer (3.6 mL Percoll, 50 μL FBS, 6 mL HBSS, 0.4 10 × PBS/100 mM HEPES/55 mM Glucose/pH 7.4) by centrifuging at 2,000 rpm for 20 min. Cells were collected, washed and non-epithelial cells were removed by incubating collected cells in a plate pre-coated with IgG (5 ug/mL) for 30 min. Epithelial cells were collected, washed and transferred to plates pre-coated with fibronectin (Calbiochem, 50 μg/mL) in DMEM/F-12 media (Hyclone) containing 1.5 g/L NAHCO3, 5 mL insulin/transferrin/sodium selenite (Gibco), 1 μg/mL hydrocortisone (Sigma), 1 mM L-glutamine, 10% FBS, and Pen-Strep. Upon confluency, cells were passaged and expanded for use.
Western Blot
For whole lung protein fractions, mice were euthanized 24 h after inoculation as described above, lungs were homogenized, and protein was extracted using RIPA buffer (50 mM Tris-HCl pH 7.5, 2 mM EDTA, 150 mM NaCl, 1% NP-40, 0.1% SDS, 0.5% Na-deoxycholate, 50 mM NaF). Primary alveolar epithelial cells were grown to ~80–90% confluence on T-75 flasks and were treated with IAV (MOI 1) or PBS. At 24 h after treatment cells were washed with ice-cold PBS and cytoplasmic protein fraction was extracted with Buffer A (10 mM HEPES pH 8.0, 0.5% NP-40, 1.5 mM MgCl2, 10 mM KCl, 0.5 mM DTT, and 200 mM sucrose). The tubes were incubated for 30 min on ice, and then centrifuged at 14,000 rpm at 4°C and the supernatant was collected. The pellets were resuspended in 30 μl of RIPA buffer and incubated on ice for 30 min. Then they were centrifuged at 14,000 rpm at 4°C and the supernatant was collected as the nuclear protein fraction. Proteins were quantified using BCA (Pierce). Proteins were run on a 10% SDS-Page gel, transferred to nitrocellulose, and stained for either STAT3 (Cell Signaling; D1B2), P-STAT3 (Cell Signaling; Tyr705, D3A7), or beta-actin (BioLegend; Poly6221). Protein levels were normalized to beta-actin and fold change was calculated over WT PBS.
Statistical Analyses
Reported results are means ± SD of ≥4 mice/group from a single experiment. Each experiment for which results are presented in the manuscript was independently performed at least twice with similar results. The differences between treatment groups were analyzed by analysis of variance (ANOVA) or Student's t-test (two-tailed) using GraphPad Prism software V.7.0d. For the differences in survival Kaplan-Meier curves were plotted and analyzed using GraphPad Prism software (Version 4.0; La Jolla, CA) using Gehan-Breslow-Wilcoxon (Logrank) test. Statistical differences with P < 0.05 were considered significant.
Results
Absence of IFNAR2 Causes Increased Morbidity and Mortality in Response to IAV Infection
It is well-known that type I IFNs are involved in anti-viral immunity to influenza and involved in BSI outcome. As type I IFNs are recognized by and signal through the heterodimeric IFNAR1/IFNAR2 receptor, depletion of the IFNAR1 subunit (Ifnar1−/−) has been assumed to eliminate type I IFN signaling in mice. However, IFN-β has recently been shown to ligate the IFNAR subunits independently resulting in distinct gene induction (11), suggesting that the individual receptors may have distinct functions following IFN recognition. Since the Ifnar2−/− mice have not been characterized in terms of susceptibility to IAV or BSI, we first sought to determine the role of IFNAR2 throughout the course of IAV infection. We found that Ifnar2−/− mice were more susceptible to IAV infection than WT mice indicated by earlier weight loss (Figure 1A) and significantly reduced survival (Figure 1B). Unlike Ifnar1−/− mice, that in our hands had similar viral load to WT mice throughout influenza disease (9), Ifnar2−/− mice had increased viral burden on both days 3 and 7 of IAV infection compared to WT mice (Figure 1C). These and our previously published results imply that the presence of IFNAR2 is sufficient for controlling viral infection in Ifnar1−/− mice (9), but the presence of IFNAR1 in Ifnar2−/− mice is not. Both weight loss and morbidity can be due to increased cellular recruitment into the lung, but also inflammation resulting from cellular and/or tissue damage. At day 3 post-IAV, when the virus titer is typically at its peak (19), neither the pattern of cellular recruitment into the lung (Figure 1D) nor the extent of lung damage as measured by LDH (Figure 1E) and albumin (Figure 1F) were different between Ifnar2−/− and WT mice. This suggests that IFNAR2 deficiency does not affect cell recruitment or lung integrity early during IAV infection. At day 7 post-IAV, when mice experience the peak of body weight loss, Ifnar2−/− mice had increased overall cell numbers with significantly more neutrophils recruited to the lung compared to WT mice (Figure 1D). The increased number of neutrophils in Ifnar2−/− mice corresponded to an increase in LDH (Figure 1E), but not in albumin (Figure 1F) when compared to WT mice.
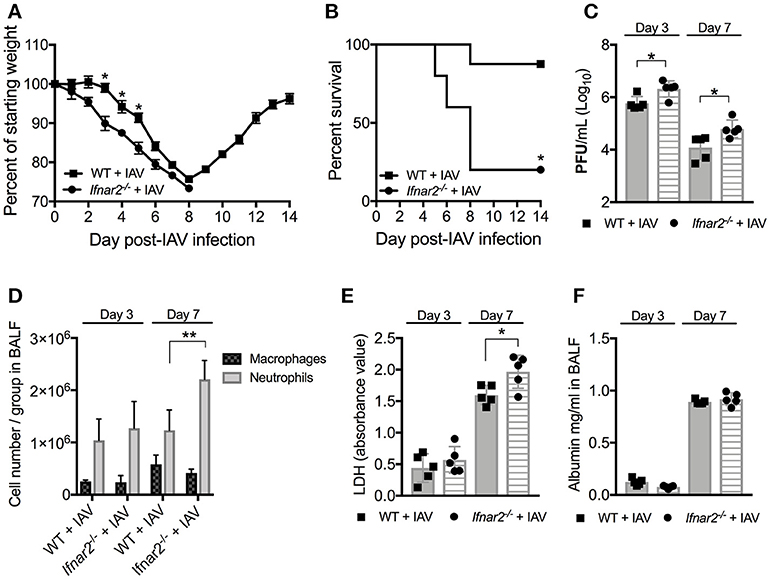
Figure 1. Ifnar2−/− mice are more susceptible to IAV. WT and Ifnar2−/− mice were infected with IAV or PBS on day 0. (A) Weights were monitored daily and are represented as percent of starting body weight depicted as average/group (Significance represents WT+IAV compared to Ifnar2−/−+IAV). (B) Survival is represented as percent survival/group. PFUs (C) were determined on day 3 or day 7 post-IAV. (D) Differential counts were determined from cell-free BALF on day 3 or day 7 post-IAV. LDH (E) and Albumin (F) were analyzed from cell-free BALF on day 3 or day 7 post-IAV. Data shown are mean ± SD results of ≥4 animals per group from one representative experiment. **P >0.01; *P > 0.05.
Absence of IFNAR2 Alters the Inflammatory Environment During IAV
Due to the impaired anti-viral immune response in Ifnar2−/− mice and enhanced neutrophil recruitment later during IAV infection, we sought to determine whether these mice exhibit reduced anti-viral cytokines in the lung throughout IAV infection. At day 3 post-IAV we found a decrease in IFNγ and IL-6 levels in the Ifnar2−/− mice compared to WT mice (Figure 2A). The Ifnar2−/− mice at day 3 post-IAV also had about 1 log less of each IFN-β and IFN-α in their lungs compared to WT mice. At day 7 post-IAV there was an increase in the inflammatory cytokines IL-1α, IFNγ, and IL-6 and a decrease in the anti-inflammatory cytokine IL-10 compared to WT mice (Figure 2B). The increase in inflammatory cytokines at day 7 post-IAV in Ifnar2−/− mice compared to WT mice correlated with the increased neutrophil recruitment and cellular damage (LDH) found in these mice at that time (Figures 1D,E). These results imply that induction of a proinflammatory response early after IAV infection is reduced in the absence of IFNAR2, whereas later after IAV, lack of IFNAR2 resulted in exacerbated lung inflammation.
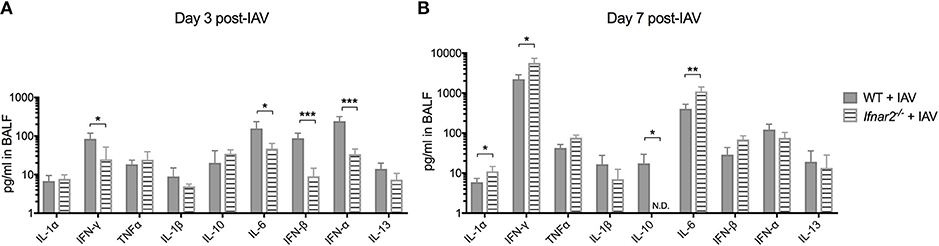
Figure 2. Absence of IFNAR2 initially reduces the inflammatory environment during IAV. WT and Ifnar2−/− mice were infected with IAV or PBS on day 0. Indicated cytokines were measured in cell-free BALF collected on (A) day 3 or (B) day 7 post-IAV. Data shown are mean ± SD results of ≥4 animals per group from one representative experiment. N.D. is not detectable. **P >0.01; *P > 0.05.
Absence of IFNAR2 Signaling Limits IAV Burden, but Does Not Alter Susceptibility to BSI at Day 3 Post-IAV
Previously we found that while IFNAR1 was not required for anti-IAV immunity (9), it was important for controlling host susceptibility to S. aureus BSI. Specifically, we demonstrated that Ifnar1−/− mice were more susceptible to BSI than WT mice on day 3 post-IAV, but less susceptible on day 7 post-IAV (9). Because our results thus far indicate that IFNAR2 is important for anti-viral immunity to IAV, we next sought to determine whether the increased IAV-susceptibility of Ifnar2−/− mice affected their BSI susceptibility, and whether Ifnar2−/− mice exhibit a similar pattern of BSI susceptibility as Ifnar1−/− mice. To this end we found that Ifnar2−/− mice superinfected with S. aureus on day 3 post-IAV had comparable lung bacterial burden to Ifnar2−/− mice infected with S. aureus alone (Figure 3A). Bacterial burden of either the BSI-Ifnar2−/− mice or the Ifnar2−/− mice infected with S. aureus alone did not significantly differ from that of S. aureus-only infected WT control mice. This suggested that IFNAR2 deficiency has no effect on host susceptibility to respiratory infection with S. aureus, whether introduced alone or as BSI on day 3 post-IAV. Because we previously found that IAV-infected Ifnar1−/− mice were more susceptible to day 3 BSI than either their mock-inoculated littermates or IAV-infected WT mice (9), collectively our results indicate that presence of IFNAR1 is sufficient to protect mice from altered BSI susceptibility at day 3 post-IAV. Previously we reported that IFNAR1 deficiency had no effect on either lung viral burden or influenza disease progression both prior to and following BSI (9). In contrast to these findings in Ifnar1−/− mice, here we found that lung virus burden of Ifnar2−/− mice was significantly higher than that of WT mice both prior and 24 h after BSI induced on day 3 post-IAV (Figures 1C, 3B). This suggested that despite increased IAV susceptibility of mice in the absence of IFNAR2, BSI early after IAV infection did not exacerbate IAV disease in Ifnar2−/− mice. Along these lines, BSI at day 3 post-IAV did not further exacerbate body weight loss and morbidity of IAV-infected Ifnar2−/− mice (Figures 3C,F), further indicating that IFNAR2 may be more important for viral immunity than bacterial immunity. When compared to BSI-WT mice the BSI-Ifnar2−/− mice had similar levels (Figure 3D) and types (Figure 3E) of cells recruited to the lung during BSI, indicating that the Ifnar2−/− mice do not have a defect in cellular responses during a BSI. The superinfected Ifnar2−/− mice also did not have significantly altered LDH (Figure 3G) or albumin (Figure 3H) levels in the BALF compared to WT mice. This suggests that at day 3 BSI with S. aureus the absence of IFNAR2 did not have a substantial effect on damage, similar to what we found early during IAV infection alone (Figure 1). Importantly, although IFNAR2 is not required for protection from day 3 BSI post-IAV, our results thus far demonstrate that when examined individually, the IFNAR subunits can lead to distinct outcomes.
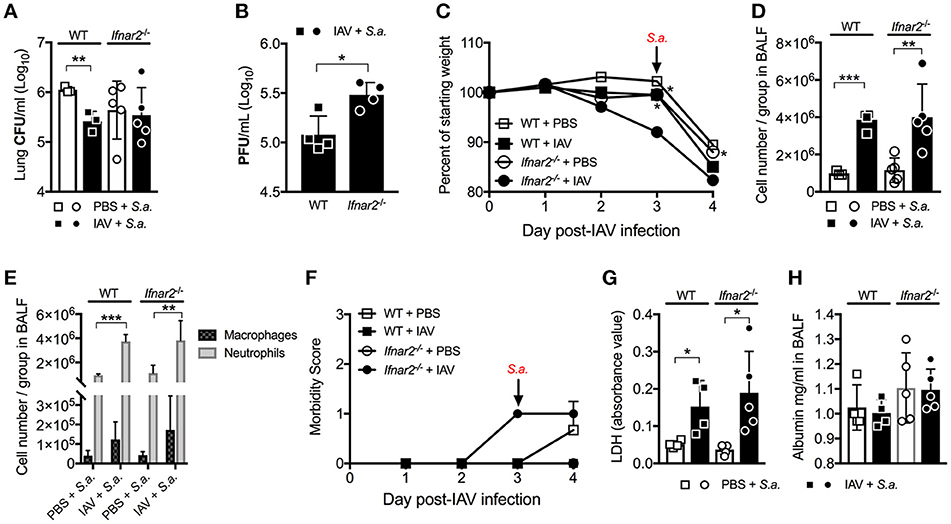
Figure 3. Absence of IFNAR2 does not alter susceptibility to day 3 BSI post-IAV. WT and Ifnar2−/− mice were infected with IAV or PBS on day 0 and challenged with S. aureus on day 3. Lung CFUs (A) and PFUs (B) were determined 24 h post-S.a.-challenge. (C) Weights were monitored daily and are represented as percent of initial body weight depicted as average/group (Significance represents WT+IAV or Ifnar2−/− compared to Ifnar2−/−+IAV). Cell number (D) and differential counts (E) were determined from cell-free BALF 24 h post- S.a.-challenge. (F) Mice were monitored and scored daily for signs of morbidity as described in methods section. Average daily score for each group is depicted. LDH (G) and Albumin (H) were analyzed in the cell-free BALF collected at 24 h post- S.a.-challenge. Data shown are mean ± SD results of ≥4 animals per group from one representative experiment. ***P >0.001; **P >0.01; *P > 0.05.
Ifnar2−/− Mice Have Reduced Susceptibility to Day 7 BSI Post-IAV
Unlike BSI at day 3 post-IAV, mice and humans are known to be more susceptible to BSI around day 7 post-IAV infection. Our laboratory and others have shown this susceptibility to depend on IFNAR1 with Ifnar1−/− mice being less susceptible to BSI at day 7 post-IAV compared to WT mice (7, 9, 20, 21). Thus, we next sought to determine whether IFNAR2 plays a similar role as IFNAR1 in the susceptibility to day 7 post-IAV BSI. Ifnar2−/− mice, similar to what we found for Ifnar1−/− mice (9), were protected from BSI at day 7 post-IAV as compared to S. aureus-Ifnar2−/− mice (Figure 4A). Like mice deficient in IFNAR1 signaling (9), when compared to WT mice Ifnar2−/− mice had similar levels of virus following BSI at day 7 post-IAV (Figure 4B). While Ifnar2−/− and WT mice had similar virus burden at 24 h post-BSI (Figure 4B), singly infected Ifnar2−/− mice at day 7 post-IAV infection showed 1 log increase in the lung viral burden when compared to IAV-only infected WT mice (Figure 1C). This suggests that in the absence of IFNAR2, superinfection on day 7 prevents further increases in viral burden. Although the IAV infected Ifnar2−/− mice had increased early weight loss compared to WT mice prior to BSI on day 7 (Figure 4C), these mice had a similar percentage of weight loss in response to S. aureus challenge (Figure 4C). However, IAV infected Ifnar2−/− mice had increased morbidity compared to IAV infected WT mice both before and after challenge with S. aureus (Figure 4F), further suggesting that IFNAR2 signaling may be involved in regulating anti-viral immunity. At 24 h after day 7 BSI, WT, and Ifnar2−/− mice had similar numbers (Figure 4D) and types (Figure 4E) of cells in the lung. This again was interesting because at day 7 post-IAV infection, Ifnar2−/− mice showed an increase in number of neutrophils when compared to IAV-infected WT mice at that time (prior to S. aureus challenge; Figure 1D). This suggests that the increased number of neutrophils recruited during IAV and prior to BSI, may contribute to the decreased susceptibility of the Ifnar2−/− mice to BSI at day 7, as neutrophils are known to be involved in anti-S. aureus immunity (22, 23). Similar to BSI at day 3 post-IAV, BSI at day 7 post-IAV did not significantly increase levels of LDH (Figure 4G) or albumin (Figure 4H) in the lung. These results indicate that like IFNAR1, presence of IFNAR2 is detrimental to BSI at day 7 post-IAV. These results also suggest that response to bacterial challenge may be beneficial for the late anti-viral response in the Ifnar2−/− mice.
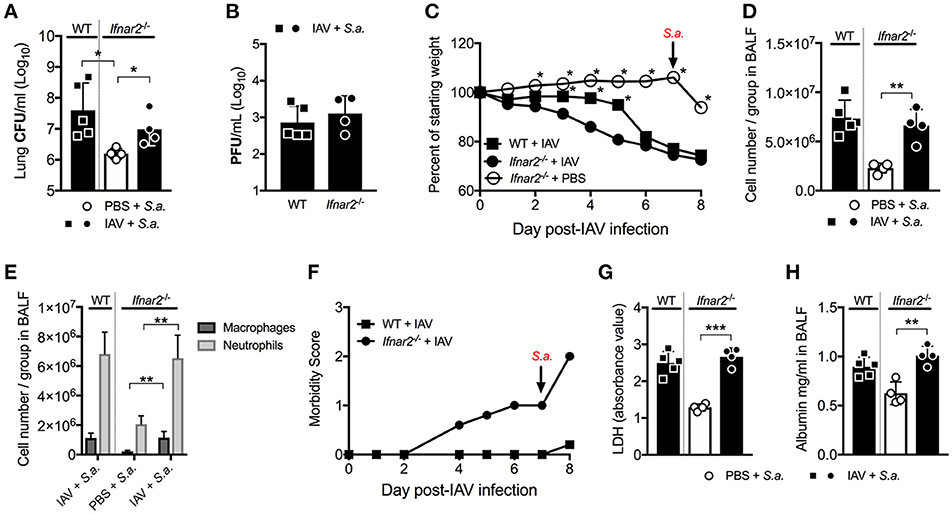
Figure 4. Ifnar2−/− mice have reduced susceptibility to day 7 BSI post-IAV. WT and Ifnar2−/− mice were infected with IAV or PBS on day 0 and challenged with S. aureus on day 7. Lung CFUs (A) and PFUs (B) were determined 24 h post-S.a.-challenge. (C) Weights were monitored daily and are represented as percent of starting body weight depicted as average/group (Significance represents WT+IAV or Ifnar2−/− compared to Ifnar2−/−+IAV). Cell number (D) and differential counts (E) were determined from cell-free BALF 24 h post- S.a.-challenge. (F) Morbidity was assessed and monitored daily. Average daily score for each group is depicted. LDH (G) and Albumin (H) were analyzed in the cell-free BALF collected at 24 h post- S.a.-challenge. Data shown are mean ± SD results of ≥4 animals per group from one representative experiment. ***P >0.001; **P >0.01; *P > 0.05.
Ifnar2−/− Mice Have Decreased Inflammatory Cytokines at Day 3 and Increased IFNγ at Day 7 Post-IAV BSI
Since IFNAR2 deficiency had no effect on numbers and types of recruited cells in response to BSI either at day 3 or day 7 post-IAV, we next sought to determine whether cytokines produced by WT and Ifnar2−/− mice after BSI contribute to differences in BSI severity. In response to day 3 BSI, Ifnar2−/− mice had less IFNγ, TNFα, and IL-6 compared to BSI-WT mice, indicating that the Ifnar2−/− mice develop a less inflammatory lung environment (Figure 5A). The reduction in inflammatory cytokines in response to BSI at day 3 in Ifnar2−/− mice did not correspond to less cells or less damage (Figures 3D,G), suggesting there is another mechanism involved. Moreover, the reduction in inflammatory cytokines we found in day 3 BSI-Ifnar2−/− mice (Figure 5A) did not occur in Ifnar2−/− mice in response to single infection with S. aureus alone (Supplemental Figure 1). As it relates to interferon responses, the Ifnar2−/− mice also had increased IFN-α on day 3 BSI compared to WT mice. In response to day 7 BSI, Ifnar2−/− mice only had an increase in IFNγ compared to BSI-WT mice (Figure 5B). These results suggest that the decreased susceptibility of Ifnar2−/− mice to day 7 BSI is not due to an altered lung environment as there were no major changes in cytokine levels associated with anti-viral or anti-bacterial immunity. Together, these results suggest that the inflammatory environment on day 3 and day 7 may be more related to IAV outcome than the outcome of BSI or single S. aureus infection.
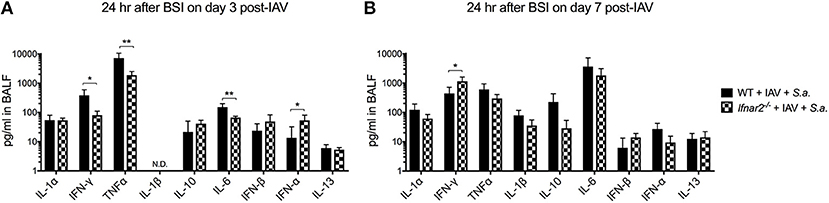
Figure 5. Ifnar2−/− mice have decreased inflammatory cytokines at day 3 and increased IFNy at day 7 post-IAV BSI. WT and Ifnar2−/− mice were infected with IAV or PBS on day 0 and challenged with S. aureus on day 3 (A) or day 7 (B). Indicated cytokines were measured in cell-free BALF 24 h after S.a. challenge on day 3 post-IAV. N.D. is not detectable. **P >0.01; *P > 0.05.
STAT3 Contributes to the Increased Susceptibility of Ifnar1−/− Mice, but Not Ifnar2−/− Mice to Day 3 BSI Post- IAV
IFN-β and the IFN-α's are known to have different affinities for IFNAR1 and IFNAR2 and to induce different gene expression profiles depending on their concentration and timing (11, 24). Thus, we next wanted to determine whether engagement of either IFNAR subunit by IAV resulted in induction of distinct anti-viral pathways. We found that both IFNAR subunits were required for the early expression of STAT1/2 and IRF3/7 to the levels found in WT mice (IFNAR+/+) following viral recognition (Figure 6A). Interestingly, early IAV infection in Ifnar1−/− mice resulted in a significant increase in STAT3 expression in the lung compared to both WT and Ifnar2−/− mice. This increase in expression of STAT3 in Ifnar1−/− mice corresponded to the high level of nuclear STAT3 protein found in Ifnar1−/− primary pulmonary epithelial cells regardless of IAV infection (Figure 6B). This indicated that STAT3 activation in Ifnar1−/− mice may have a role in early anti-viral immunity and subsequent BSI severity at day 3 in these mice. To address this possibility, we treated the IFNAR subunit-knockout mice with a STAT3 phosphorylation inhibitor (15) 6 h after IAV infection and determined their susceptibility to BSI at day 3 post-IAV. We found that P-STAT3 inhibition in both the WT and Ifnar1−/− mice resulted in reduced susceptibility to BSI, 0.5 log and 1 log (Figure 6C), respectively, compared to untreated littermate mice. Additionally, we found that P-STAT3 inhibition did not alter susceptibility of Ifnar2−/− mice to BSI on day 3, indicating that IFNAR1 and IFNAR2 induce disparate mechanisms in response to viral infection. Upon analyzing the cell abundances in the BALF of the WT, Ifnar1−/− and Ifnar2−/− mice we only found the P-STAT3-inhibited Ifnar1−/− mice to have decreased levels of neutrophils (Figure 6D). To determine whether P-STAT3 inhibition altered the anti-viral state of mice at 24 h after day 3 BSI, we analyzed their cytokine profiles. When compared to untreated littermates, the P-STAT3 inhibition did not alter cytokines produced by WT mice (Figure 6E), but resulted in decreased IFNγ and IL-6 in Ifnar2−/− mice (Figure 6E). The decreased IFNγ and IL-6 in P-STAT3-inhibited Ifnar2−/− mice did not appear to significantly affect BSI outcome in these mice (Figure 6C). In Ifnar1−/− mice superinfected on day 3, P-STAT3 inhibition resulted in decreased TNFα and a 10-fold increase in IL-13 levels compared to untreated superinfected Ifnar1−/− mice. These results are consistent with our previous findings that treatment with mrIL-13 reversed the increased day 3 BSI susceptibility and resulted in decreased neutrophil accumulation in Ifnar1−/− mice (9). Therefore, our results expand our previous findings by demonstrating that STAT3 contributes to increased susceptibility of Ifnar1−/− mice to BSI at day 3 post-IAV at least in part by inhibition of IL-13 production.
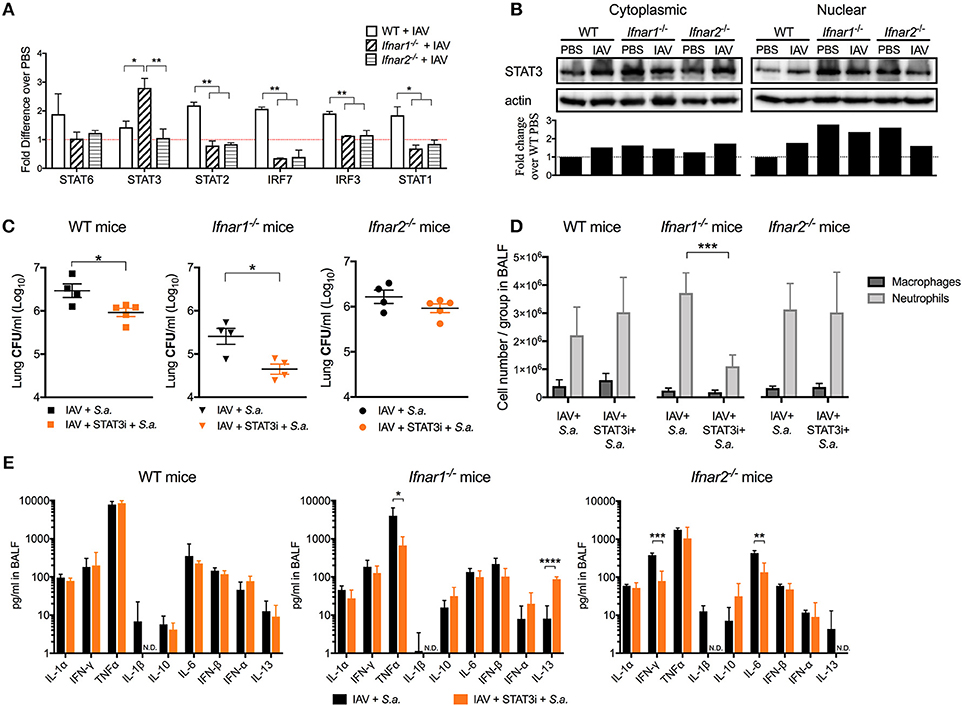
Figure 6. STAT3 contributes to the increased susceptibility of day 3 BSI-Ifnar1−/− mice post-IAV. (A) WT, Ifnar1−/−, and Ifnar2−/− mice were infected with IAV or PBS on day 0 and gene expression from RNA isolated from the whole lung was analyzed. (B) STAT3 protein was analyzed from cytoplasmic and nuclear fractions isolated from WT, Ifnar1−/−, and Ifnar2−/− primary alveolar epithelial cells infected with IAV or inoculated PBS for 24 h. STAT3 was normalized to b-actin protein level and fold change over WT-PBS was calculated (Whole western blots: Supplemental Figures 2–5). (C–E) WT, Ifnar1−/−, and Ifnar2−/− mice were infected with IAV on day 0, inoculated with STAT3 inhibitor or PBS 6 h post-IAV, and challenged with S. aureus on day 3. (C) Lung CFUs, (D) differential counts from the cell-free BALF, and (E) indicated cytokines from cell-free BALF were analyzed 24 h post- S.a.-challenge. Data shown are mean ± SD results of ≥4 animals per group from one representative experiment. N.D. is not detectable. ***P >0.001; **P >0.01; *P > 0.05.
IFN-β Signaling Through Ifnar2 Rescued Mice From Morbidity and Mortality Upon Lethal IAV Infection
Our results thus far indicate that engagement of either IFNAR subunit individually differentially shapes both anti-IAV immunity and BSI susceptibility. These two IFNAR subunits are known to bind IFN-α's and IFN-β with different affinity inducing distinct gene profiles (11, 25). We found that IFN-αA, but not IFN-β inhibited production of IL-13 resulting in increased host susceptibility to BSI and that IFN-αA was involved in mediating increased susceptibility to S. aureus (9). Therefore, here we sought to determine whether IFN-αA and IFN-β have different effects on protection from IAV infection while signaling via distinct IFNAR subunits. IFNαA treatment of mice deficient in either IFNAR subunit (Figure 7A orange traces) had no effect on IAV-induced body weight loss when compared to either their untreated littermates or WT mice (open symbols). Treatment of mice that have functional IFNAR2 (Ifnar1−/− mice) with mrIFN-β (black triangles) protected these mice from morbidity and increased their survival compared to IAV-infected littermates (open triangle) or IAV-infected WT mice (open squares) (Figures 7A,B). Treatment of mice without functional IFNAR2 (Ifnar2−/− mice) with mrIFN-β (black circles) did not protect these mice from morbidity or mortality when compared to mrIFN-treated Ifnar1−/− mice. The mrIFN-β treatment of Ifnar2−/− mice however, did slightly but not significantly accelerate and worsen body weight loss when compared to untreated Ifnar2−/− mice (open circles). These results indicate that presence of IFNAR2 and signaling by IFN-β are sufficient to induce a protective anti-IAV state. Our results also suggest that IFN-β produced in the Ifnar1−/− and WT mice following IAV infection (Figures 2A, 6A) is not sufficient to provide protection and that the protective effects only occur when IFN-β is present at the very beginning of viral infection (9).
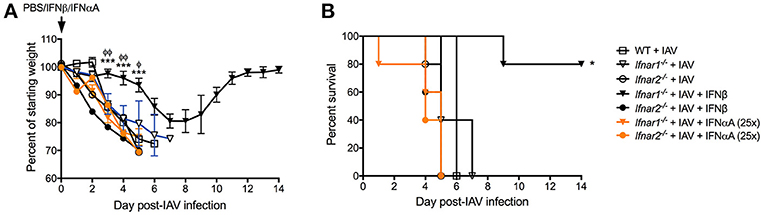
Figure 7. IFN-β can rescue influenza-mediated morbidity and mortality in Ifnar1−/− mice. WT, Ifnar1−/−, and Ifnar2−/− mice were infected with IAV or PBS on day 0 and treated with PBS, rIFN-β, or rIFN-αA at 0, 3, and 6 h post-IAV. (A) Weights were monitored daily and are represented as percent of starting body weight depicted as average/group (Significance represents Ifnar1−/−+IAV+β compared to *WT or φIfnar1−/−+IAV). (B) Survival is represented as percent survival/group Data shown are mean ± SD results of ≥4 animals per group from one representative experiment. ***P > 0.001; φφP > 0.01, φP > 0.05.
Discussion
Type I IFNs play a role in determining influenza and BSI severity. While cell surface receptor for type I IFNs consists of two distinct subunits (IFNAR1 and IFNAR2), almost all research on determining the role of type I IFNs in susceptibility to influenza and subsequent BSIs has focused solely on the involvement of IFNAR1 (using Ifnar1−/− mice). To this end our lab and others have shown that IFNAR1 is important in regulating time-dependent susceptibility to BSI during IAV infection (4, 7, 9). In the last decade, a number of reports demonstrated the ability of IFNAR1 and IFNAR2 to bind individual IFNs independent of one another, with different affinities, and subsequently causing induction of different gene (11, 24, 25). These reports alluded that the receptor subunits have distinct functions in relation to disease outcomes (25). Here, we begin to unravel how the absence of IFNAR2 affects IAV and BSI severity.
Our study revealed that IFNAR2 is required for effective anti-IAV immune responses, particularly as it relates to protection from influenza-mediated morbidity and mortality. While our results combined with work by others (5) suggest that a complete IFNAR receptor is important for protection from influenza, the accelerated morbidity and mortality, as well as increased viral burden of the Ifnar2−/− mice indicate that IFNAR2 plays a larger role than IFNAR1 in regulating the anti-viral response. That IFNAR2 deficiency resulted in increased neutrophil recruitment and an increased level of LDH production by day 7 of IAV infection further suggests that IFNAR2 may also control damage response during viral infections. Further studies are necessary to elucidate the exact mechanism by which IFNAR2 regulates anti-IAV immunity.
As it relates to host susceptibility to post-IAV BSIs, we demonstrate that IFNAR2 has both similar and distinct roles in BSI susceptibility compared to IFNAR1. Specifically, we found that IFNAR1 signaling in the absence of IFNAR2 (Ifnar2−/− mice) was sufficient to prevent the increased BSI susceptibility that we previously found to occur in Ifnar1−/− mice at day 3 post-IAV (9), suggesting that IFNAR1 alone is able to control bacterial burden more than IFNAR2 alone at day 3 post-IAV. In regards to BSI induced at day 7 post-IAV, Ifnar2−/− mice shared the same decrease in susceptibility as has been previously found for Ifnar1−/− mice (4, 9). As WT mice are more susceptible to day 7 BSI than either IFNAR subunit knockout mice, our combined results from Ifnar2−/− mice and previous results from Ifnar1−/− mice (4, 7, 9) suggest that a complete IFNAR is required for the increased susceptibility phenotype and that absence of either receptor subunit is sufficient to provide protection.
Conventional type I IFN signaling utilizes STAT1/2 heterodimer (26). A side by side comparison of intracellular signaling molecules known to be engaged in type I IFN cascade revealed preferential engagement of STAT3 upon IAV infection of Ifnar1−/−, but not Ifnar2−/− or WT mice. We found that this engagement of STAT3 contributed to the increased BSI susceptibility of Ifnar1−/− mice at day 3 post-IAV. Our previous work demonstrated that the Ifnar1−/− day 3 BSI phenotype was at least in part due to the absence of IL-13 as increased susceptibility to BSI at that time post-IAV could be reversed by treating the Ifnar1−/− mice with mrIL-13, leading to a reduction in neutrophils and bacterial burden (9). Here, we found that P-STAT3 inhibition in Ifnar1−/− mice prior to day 3 BSI similarly reduced the level of neutrophil recruitment and caused a 10-fold increase in IL-13 in response to day 3 BSI. STAT3 has been previously reported to be involved in regulating inflammatory mediators and subsequent neutrophil trafficking during infection, where inhibition of STAT3 reduced neutrophil chemokines and recruitment (27, 28). During viral infection, type I IFN signaling through IFNAR induces STAT1/STAT2 activity, but also leads to the induction of STAT3, which is thought to provide negative feedback keeping the IFN response under control (29). STAT3 deficiency was found to enhance anti-viral activity and gene expression in response to type I IFNs (30). Thus, it is tempting to speculate that in the presence of IFNAR2, the STAT3 activation in IAV-infected Ifnar1−/− mice does not allow for induction of an anti-IAV immune response. Our data imply that STAT3 induction in Ifnar1−/− mice may be involved in preventing the increase in IL-13 that is required for controlling neutrophil recruitment and bacterial killing. How IFNAR1- or IFNAR2-induced STAT3, whether in conjunction with STAT1 or other STATs, is involved in viral and BSI susceptibility remains unknown.
Differential signaling through a single cytokine receptor is not a new concept in immunology. Results of interactions between cytokines and their cognate receptors can vary depending on the specific tissue and cellular environment, availability of the substrate and/or receptor, and affinity and avidity of the interactions (31). There are multiple examples where differential cellular processes induced by different cytokines occurs through a shared common receptor (31–33). As for type I IFNs, IFN-β, and the IFN-α's have been shown to induce distinct signaling pathways depending on their abundance, with all IFNs inducing “robust” genes (anti-viral) at low concentrations, and only IFN-β inducing “tunable” (anti-proliferative) at higher, still physiologic, concentrations (34–36). Importantly, de Weerd and colleagues established that type I IFNs are able to bind the individual IFNAR subunits independently (11). Specifically, they found that IFN-β ligates IFNAR1 independently of IFNAR2 and also the opposite, that IFN-β can ligate IFNAR2 independently of IFNAR1. However, human IFNα2, which shares high homology with mouse IFN-αA, was only able to form a stable complex with the extracellular domain of IFNAR2, which is the high-affinity portion of the IFNAR receptor. Here, we demonstrate that the morbidity and mortality associated with IAV infection of mice lacking IFNAR1 can be rescued by the administration of mrIFN-β at the time of IAV infection, but IFN-β treatment did not rescue mice lacking IFNAR2. These results suggest that the presence of IFNAR2 is required to generate a protective anti-viral response to IAV infection when stimulated with IFN-β, but that presence of IFNAR1 is not. Which response, whether it be the robust or tunable, and which of those genes are important for providing protection to IAV infection by IFNAR2 in the Ifnar1−/− mice will provide insight into how these receptor subunits are regulating the immune response to IAV. A better understanding of how the IFN-β-IFNAR2 complex in the absence of IFNAR1 can lead to protection will improve our knowledge of viral immunity.
Collectively our results demonstrate that either IFNAR subunit is sufficient for interferon signaling in vivo. As we began to elucidate differences in how the individual subunits shape the anti-viral immune response we found that IFNAR2 plays a non-redundant role in induction to anti-viral immunity. As such, we found IFNAR2 to be essential for both decreasing the morbidity and mortality associated with IAV infections and in altering subsequent host susceptibility to BSI. While further studies will determine the intracellular signaling mechanisms utilized by individual IFNAR subunits and whether these subunits have distinct outcomes in other viral and bacterial infections, results presented here set a stage for these mechanistic studies by emphasizing the importance of understanding the contributions of the entire receptor to disease outcomes.
Ethics Statement
All animal experiments were approved by the Montana State University Institutional Animal Care and Use Committee.
Author Contributions
KMS designed and performed experiments, analyzed data, and contributed to writing of the paper. KL and LJ designed and performed experiments and analyzed data. KS, HC, JW, and HH performed experiments. AR-A designed experiments, analyzed data, and contributed to writing of the paper.
Funding
This work was supported by the following grants: National Institutes of Health (NIH/NIAID) R01AI04905, (NIH/NIAID) R21AI119772, (NIH/NIGMS) (P30GM110732), Francis Family Foundation: Parker B. Francis Fellowship Program, Montana State University Agricultural Experiment Station, MJ Murdock Charitable Trust, and, Montana University System Research Initiative (51040-MUSRI2015-03).
Conflict of Interest Statement
The authors declare that the research was conducted in the absence of any commercial or financial relationships that could be construed as a potential conflict of interest.
Acknowledgments
We thank Victor Huber for valuable discussion and Diane Bimczok for valuable comments and suggestions.
Supplementary Material
The Supplementary Material for this article can be found online at: https://www.frontiersin.org/articles/10.3389/fimmu.2018.02589/full#supplementary-material
References
1. Rice TW, Rubinson L, Uyeki TM, Vaughn FL, John BB, Miller RRI, et al. Critical illness from 2009 pandemic influenza A virus and bacterial coinfection in the United States. Crit Care Med. (2012) 40:1487–98. doi: 10.1097/CCM.0b013e3182416f23
2. Wong KK, Jain S, Blanton L, Dhara R, Brammer L, Fry AM, Finelli L. Influenza-associated pediatric deaths in the United States, 2004-2012. Pediatrics (2013) 132:796–804. doi: 10.1542/peds.2013-1493
3. Morens DM, Taubenberger JK, Fauci AS. Predominant role of bacterial pneumonia as a cause of death in pandemic influenza: implications for pandemic influenza preparedness. J Infect Dis. (2008) 198:962–70. doi: 10.1086/591708
4. Shahangian A, Chow EK, Tian X, Kang JR, Ghaffari A, Liu SY, et al. Type I IFNs mediate development of postinfluenza bacterial pneumonia in mice. J Clin Invest. (2009) 119:1910–20. doi: 10.1172/JCI35412
5. Seo S-U, Kwon H-J, Ko H-J, Byun Y-H, Seong BL, Uematsu S, et al. Type I interferon signaling regulates Ly6C(hi) monocytes and neutrophils during acute viral pneumonia in mice. PLoS Pathog. (2011) 7:1001304. doi: 10.1371/journal.ppat.1001304
6. Li W, Moltedo B, Moran TM. Type I interferon induction during influenza virus infection increases susceptibility to secondary Streptococcus pneumoniae infection by negative regulation of γδ T cells. J Virol. (2012) 86:12304–12. doi: 10.1128/JVI.01269-12
7. Lee B, Robinson KM, McHugh KJ, Scheller EV, Mandalapu S, Chen C, et al. Influenza-induced type I interferon enhances susceptibility to gram-negative and gram-positive bacterial pneumonia in mice. Am J Physiol Lung Cell Mol Physiol. (2015) 309:L158–67. doi: 10.1152/ajplung.00338.2014
8. Garcia-Sastre A, Durbin RK, Zheng HY, Palese P, Gertner R, Levy DE, et al. The role of interferon in influenza virus tissue tropism. J Virol. (1998) 72:8550–8.
9. Shepardson KM, Larson K, Morton RV, Prigge JR, Schmidt EE, Huber VC, et al. Differential type I interferon signaling is a master regulator of susceptibility to postinfluenza bacterial superinfection. mBio (2016) 7:e00506–16. doi: 10.1128/mBio.00506-16
10. Davidson S, Crotta S, McCabe TM, Wack A. Pathogenic potential of interferon αβ in acute influenza infection. Nat Commun. (2014) 5:3864. doi: 10.1038/ncomms4864
11. de Weerd NA, Vivian JP, Nguyen TK, Mangan NE, Gould JA, Braniff S-J, et al. Structural basis of a unique interferon-β signaling axis mediated via the receptor IFNAR1. Nat Immunol. (2013) 14:901–7. doi: 10.1038/ni.2667
12. National Research Council. Guide for the Care and Use of Laboratory Animals. 8th ed. Washington, DC: National Academies Press (2011).
13. Rynda-Apple A, Dobrinen E, McAlpine M, Read A, Harmsen A, Richert LE, et al. Virus-like particle-induced protection against MRSA pneumonia is dependent on IL-13 and enhancement of phagocyte function. Am J Pathol. (2012) 181:196–210. doi: 10.1016/j.ajpath.2012.03.018
14. Wiley JA, Cerwenka A, Harkema JR, Dutton RW, Harmsen AG. Production of interferon-γ by influenza hemagglutinin-specific CD8 effector T cells influences the development of pulmonary immunopathology. Am J Pathol. (2001) 158:119–30. doi: 10.1016/S0002-9440(10)63950-8
15. Onimoe G-I, Liu A, Lin L, Wei C-C, Schwartz EB, Bhasin D, et al. Small molecules, LLL12 and FLLL32, inhibit STAT3 and exhibit potent growth suppressive activity in osteosarcoma cells and tumor growth in mice. Invest New Drugs (2012) 30:916–26. doi: 10.1007/s10637-011-9645-1
16. Rynda-Apple A, Harmsen A, Erickson AS, Larson K, Morton RV, Richert LE, et al. Regulation of IFN-γ by IL-13 dictates susceptibility to secondary postinfluenza MRSA pneumonia. Eur J Immunol. (2014) 44:3263–72. doi: 10.1002/eji.201444582
17. Zhao W, Li Q, Ayers S, Gu Y, Shi Z, Zhu Q, et al. Jmjd3 inhibits reprogramming by upregulating expression of INK4a/Arf and targeting PHF20 for ubiquitination. Cell (2013) 152:1037–50. doi: 10.1016/j.cell.2013.02.006
18. Hiroi M, Sakaeda Y, Yamaguchi H, Ohmori Y. Anti-inflammatory cytokine interleukin-4 inhibits inducible nitric oxide synthase gene expression in the mouse macrophage cell line RAW264.7 through the repression of octamer-dependent transcription. Mediators Inflamm. (2013) 2013:14. doi: 10.1155/2013/369693
19. Baccam P, Beauchemin C, Macken CA, Hayden FG, Perelson AS. Kinetics of influenza A virus infection in humans. J Virol. (2006) 80:7590–9. doi: 10.1128/JVI.01623-05
20. Sun K, Metzger DW. Inhibition of pulmonary antibacterial defense by interferon-γ during recovery from influenza infection. Nat Med. (2008) 14:558–64. doi: 10.1038/nm1765
21. Robinson KM, Choi SM, McHugh KJ, Mandalapu S, Enelow RI, Kolls JK, et al. Influenza A exacerbates Staphylococcus aureus pneumonia by attenuating IL-1β production in mice. J Immunol. (2013) 191:5153–9. doi: 10.4049/jimmunol.1301237
22. Sun K, Metzger DW. Influenza infection suppresses NADPH oxidase-dependent phagocytic bacterial clearance and enhances susceptibility to secondary methicillin-resistant Staphylococcus aureus infection. J Immunol. (2014) 192:3301–7. doi: 10.4049/jimmunol.1303049
23. Lekstrom-Himes JA, Gallin JI. Immunodeficiency diseases caused by defects in phagocytes. N Engl J Med. (2000) 343:1703–14. doi: 10.1056/NEJM200012073432307
24. Porritt RA, Hertzog PJ. Dynamic control of type I IFN signalling by an integrated network of negative regulators. Trends Immunol. (2015) 36:150–60. doi: 10.1016/j.it.2015.02.002
25. de Weerd NA, Samarajiwa SA, Hertzog PJ. Type I interferon receptors: biochemistry and biological functions. J Biol Chem. (2007) 282:20053–7. doi: 10.1074/jbc.R700006200
26. Au-Yeung N, Mandhana R, Horvath CM. Transcriptional regulation by STAT1 and STAT2 in the interferon JAK-STAT pathway. JAK-STAT (2013) 2:e23931. doi: 10.4161/jkst.23931
27. Fielding CA, McLoughlin RM, McLeod L, Colmont CS, Najdovska M, Grail D, et al. IL-6 regulates neutrophil trafficking during acute inflammation via STAT3. J Immunol. (2008) 181:2189–95. doi: 10.4049/jimmunol.181.3.2189
28. Zhang S, Hwaiz R, Luo L, Herwald H, Thorlacius H. STAT3-dependent CXC chemokine formation and neutrophil migration in streptococcal M1 protein-induced acute lung inflammation. Am J Physiol Lung Cell Mol Physiol. (2015) 308:L1159–67. doi: 10.1152/ajplung.00324.2014
29. Ho HH, Ivashkiv LB. Role of STAT3 in type I interferon responses - Negative regulation of STAT1-dependent inflammatory gene activation. J Biol Chem. (2006) 281:14111–8. doi: 10.1074/jbc.M511797200
30. Wang W-B, Levy DE, Lee C-K. STAT3 negatively regulates type I IFN-mediated antiviral response. J Immunol. (2011) 187:2578–85. doi: 10.4049/jimmunol.1004128
31. Moraga I, Spangler J, Mendoza JL, Garcia KC. Multifarious determinants of cytokine receptor signaling specificity. Adv Immunol. (2014) 121:1–39. doi: 10.1016/B978-0-12-800100-4.00001-5
32. Moussawi Al K, Kazmierczak BI. Distinct contributions of Interleukin (IL)-1α and IL-1β to innate immune recognition of Pseudomonas aeruginosa in the lung. Infect Immun. (2014) 82:4204–11. doi: 10.1128/IAI.02218-14
33. Caffrey AK, Lehmann MM, Zickovich JM, Espinosa V, Shepardson KM, Watschke CP, et al. IL-1 alpha Signaling is critical for leukocyte recruitment after pulmonary aspergillus fumigatus challenge. PLoS Pathog. (2015) 11:1004625. doi: 10.1371/journal.ppat.1004625
34. Levin D, Schneider WM, Hoffmann H-H, Yarden G, Busetto AG, Manor O, et al. Multifaceted activities of type I interferon are revealed by a receptor antagonist. Sci Signal. (2014) 7:ra50. doi: 10.1126/scisignal.2004998
35. Levin D, Harari D, Schreiber G. Stochastic receptor expression determines cell fate upon interferon treatment. Mol Cell Biol. (2011) 31:3252–66. doi: 10.1128/MCB.05251-11
Keywords: IFNAR2, IFNAR1, Influenza, Staphylococcus aureus, superinfection
Citation: Shepardson KM, Larson K, Johns LL, Stanek K, Cho H, Wellham J, Henderson H and Rynda-Apple A (2018) IFNAR2 Is Required for Anti-influenza Immunity and Alters Susceptibility to Post-influenza Bacterial Superinfections. Front. Immunol. 9:2589. doi: 10.3389/fimmu.2018.02589
Received: 01 August 2018; Accepted: 22 October 2018;
Published: 09 November 2018.
Edited by:
John F. Alcorn, University of Pittsburgh, United StatesReviewed by:
Irving Coy Allen, Virginia Tech, United StatesHoward M. Johnson, University of Florida, United States
Copyright © 2018 Shepardson, Larson, Johns, Stanek, Cho, Wellham, Henderson and Rynda-Apple. This is an open-access article distributed under the terms of the Creative Commons Attribution License (CC BY). The use, distribution or reproduction in other forums is permitted, provided the original author(s) and the copyright owner(s) are credited and that the original publication in this journal is cited, in accordance with accepted academic practice. No use, distribution or reproduction is permitted which does not comply with these terms.
*Correspondence: Agnieszka Rynda-Apple, agnieszka.rynda@montana.edu