- 1Research Group of Gastrointestinal Diseases, The Second Affiliated Hospital of Zhengzhou University, Zhengzhou, Henan, China
- 2Faculty of Health Science, Nord University, Levanger, Norway
- 3College of Medical Imaging, Mudanjiang Medical University, Mudanjiang, China
- 4School of Stomatology, Wuhan University, Wuhan, China
The majority of colorectal cancers (CRCs) are thought to arise from precancerous adenomas. Upon exposure to diverse microenvironmental factors, precancerous stem cells (pCSCs) undergo complex genetic/molecular changes and gradually progress to form cancer stem cells (CSCs). Accumulative evidence suggests that the pCSC/CSC niche is an inflammatory dominated milieu that contains different cytokines that function as the key communicators between pCSCs/CSCs and their niche and have a decisive role in promoting CRC development, progression, and metastasis. In view of the importance and increasing data about cytokines in modulating pCSCs/CSC stemness properties and their significance in CRC, this review summarizes current new insights of cytokines, such as interleukin (IL)-4, IL-6, IL-8, IL-17A, IL-22, IL-23, IL-33 and interferon (IFN)-γ, involving in the modulation of pCSC/CSC properties and features in precancerous and cancerous lesions and discusses the possible mechanisms of adenoma progression to CRCs and their therapeutic potential.
Introduction
Globally, colorectal cancer (CRC) is the fourth most frequent cancer and it seriously endangers the public health because of its high incidence and mortality. According to data from 2020, 1.8 million newly diagnosed cases and 0.8 million deaths were estimated worldwide (1). Although the precise mechanism for CRC development is not fully clear, numerous clinical and basic studies have strongly suggested that the development of CRC is mostly a result of a stepwise process of histological, genetic, molecular, and immunological alterations in precancerous adenomas over time (2). Clinical observations also revealed that only a small number of adenomas eventually progress to CRCs and most adenomas maintain a relatively stable status for years (3). Furthermore, although the exact mechanisms by which various factors determine the progression of precancerous adenoma to CRC remain incompletely resolved, extensive evidence suggests that cancers originally develop from a subpopulation of tumor-initiating cells, termed cancer stem cells (CSCs) (4). CSCs are involved not only in tumor initiation, but also in tumor progression, invasion, metastasis, and resistance to anticancer therapies (5). Precancerous stem cells, referred to as pCSCs, in adenomatous lesions may have the potential for bidirectional differentiation into both benign and malignant lesions, depending on their niche (6). Studies have shown that the interaction between pCSCs and their niche has a decisive role in directing the differentiation of pCSCs to CSCs, which initiates the transitional process of adenoma to CRC (7). Currently, significant efforts have been made toward the evaluation of modulatory factors’ effects on pCSCs/CSCs within the tumor microenvironment (TME) (8).
The adenoma/CRC TME is an inflammatory milieu, in which several types of immune cells are recruited, activated, and then undergo phenotypic and functional changes (9). Accumulated data show that these immune cells together with other types of cells, e.g., tumor cells, stromal cells, mesenchymal stem cells (MSCs) and tumor endothelial cells, produce large amounts of cytokines that play multiple modulatory roles in immunosuppression regulation, tumor cell growth and angiogenesis processes during colorectal carcinogenesis. The overexpression of cytokines has been shown to be closely associated with adenoma progression and CRC metastasis (2). Emerging evidence indicates that stem cells (SCs) in both physiological and pathological conditions deeply interact with their inflammatory niche (10), in which cytokines may function as the main communicators to control the CSC properties and features that are strongly linked to the recurrence and metastasis of CRC (11). Therefore, we hypothesize that one of the potential mechanisms by which cytokines can promote the development of CRC is via the modulation of pCSCs/CSCs from colorectal precancerous adenomas to CRCs.
In view of the importance and increasing data about cytokines as communicators in mediating crosstalk between pCSCs/CSCs and their surrounding niche, understanding this modulatory effect is essential to exploit the functional mechanisms of pCSCs/CSCs as their translation from precancerous lesions to cancerous lesions. Thus, this review will summarize recent advances in the research field of cytokines involved in the modulation of pCSC/CSC properties and functions. In addition, the possible mechanisms of the progression of pCSCs to CSCs and their therapeutic potential are discussed.
pCSCs/CSCs in the adenoma and CRC tissues
Previous studies have revealed that pCSCs/CSCs can be identified in both precancerous and cancer lesions (12). By using reverse transcription-polymerase chain reaction and RNA in-situ hybridization techniques, Jang et al. (13) evaluated the expression of various SC markers, e.g., leucine-rich repeat-containing G-protein-coupled receptor 5 (LGR5), ASCL2, EPHB2 and olfactomedin-4 (OLFM4) in the precancerous lesions of CRC. In the normal colonic mucosa, hyperplastic polyps, and sessile serrated adenomas, SCs positive for these markers were distributed in the base region of colonic crypts. However, the distribution of SCs in adenomas was presented as a diffuse pattern in the adenomatous epithelium (13). Another study by Baker et al. (14) also showed that conventional colorectal adenomas diffusely expressed high levels of LGR5. In contrast, serrated lesions displayed basal localization of LGR5 as seen in a normal crypt. Bartley and colleagues (15) used aldehyde dehydrogenase isoform 1A1 (ALDH1A1) as a marker to identify SCs in precancerous colorectal adenomas and found that adenomas located in the right site of the colon tended to express a higher level of ALDH1A1 labeling indices than those in the left site of the colon.
Furthermore, a study by Humphries et al. (16) used a combination of nuclear and mitochondrial DNA lesions and epigenetic markers to track the lineage of SCs in human adenomas and identified SCs in precancerous adenomas. They showed that new growth of intratumor clones of SCs occurred infrequently, suggesting that SCs in precancerous adenomas were mostly in a stable condition (16).
We have previously used a various set of cell markers, such as Musashi, CD133, LGR5 and ALDH1, as biomarkers to examine the presentation of SCs in different compartments of adenomas (17). We found that populations of SCs labeled by diverse markers were increased in the adenoma tissues. In addition, a spatial change in SCs in the adenoma was observed: SCs expanded from the base to the middle part of the transitional crypt and final finally reached the surface of the adenoma, reflecting a changed track pathway of SCs during the development of adenomas. Further analysis revealed that increased populations of LGR5 and aldehyde dehydrogenase 1 (ALDH1) labeled SCs in the adenoma were associated with the degree of dysplasia, suggesting the involvement of SCs in the establishment of adenomas (17).
In a very elegant study, Gao’s group has experimentally identified a new type of SC that has the potential for both benign and malignant differentiation in colorectal precancerous lesions and named these SCs ‘pCSCs’ (6). These pCSCs have the features of both normal SCs and CSCs and are potentially regulated by the stem-cell protein Piwil2 (6), which is widely expressed by cancer cells and precancerous cells in various types of cancers (18–23). Carpentino et al. found that ALDH1-positive pCSCs were involved in the transition of colitis to CRC in xenografting studies as well as in vitro (24), which was accompanied by upregulated expression of interleukin (IL)-6 and IL-8 in the TME (24). Therefore, exploring the mechanism by which pCSCs progress to CSCs is critically important for understanding CRC development mechanisms, and targeting pCSCs may have an important translational significance in blocking the transition of precancerous lesions to cancerous lesions.
Similarly, the evaluation of CSCs in CRC has also been conducted. CSCs labeled by various markers, e.g., Musashi, CD44, CD133, CD166, epithelial cell adhesion molecules, LGR5 and ALDH1, were observed in the CRC epithelium (13–15, 17, 25–30). Further studies showed that the density of CSCs was associated with the progression, metastasis, and prognosis of patients with CRC (30–34). We tracked the temporal and spatial changes of CSCs in CRC tissues and found that CSCs were moving up from the basal to the middle region inside the adjacent transitional crypts and finally reached the surface of CRC epithelium, with an increased population (17). Recently, studies reported that CSCs increased the tumor’s ability to resist chemotherapy or immunotherapy (33, 35, 36), indicating that CSCs are as an effective target for improving the treatment of CRCs (37, 38).
The regulation of CSC properties and features is an important research field. Compelling evidence has shown that that both pCSCs in adenomas and CSCs in CRCs live in a milieu that contains dense immune cells and stromal cells (11, 39–46); these cells produce high amounts of cytokines and form an inflammatory niche that modulates pCSCs renewal, differentiation and the early onset of cancer in a coordinated manner (8, 44–46). Emerging research has shown that cytokines are involved in the modulation of CSCs (11). Therefore, it is necessary to evaluate cytokines in the surrounding niche that can specifically affect the properties and features of CSCs and determine the recurrence and metastasis of CRC.
Changes in the cytokine profile in the TME along the colorectal adenoma-carcinoma sequence
It is well known that the adenoma TME contains high densities of immune cells that produce large amounts of cytokines (2). These cytokines have complex functions by modulating immune function and interacting with premalignant adenoma cells, which determines whether premalignant lesions remain stable or progress (2). For example, we have demonstrated that the levels of interferon (IFN)-γ, IL-12A and IL-18 were increased in adenoma tissues and decreased in CRC tissues (47). Since these three cytokines have been recognized as critical factors in maintaining antitumor immunity, the increasing IFN-γ, IL-12 and IL-18 in the TME may imply an initial effort of the host to combat the appearance of adenomas in the colorectal mucosa. Importantly, IFN-γ is a cytokine that plays a critical role in controlling the survival of CSCs (48). Increased IFN-γ levels at the adenoma stage may help the host immunity to selectively eliminate pCSCs sensitive to immunosurveillance and allow pCSCs resistant to immunosurveillance to survive and progress to CSCs.
From the adenoma stage to the CRC stage, tumor-promoting relevant cytokines, such as IL-6, IL-8, IL-17A and IL-33, are significantly increased (49–52). These cytokines have been shown to stimulate CRC invasion and metastasis by inducing immunosuppression and enhancing angiogenesis (53, 54). Mechanistically, cytokine-promoted CRC progression occurs through the activation of diverse signaling pathways (41). For example, IL-4 stimulation induced the activation of signal transducer and activator of transcription (STAT)-6 phosphorylation in epithelial cells (55). Enhanced STAT-6 phosphorylation in patients with CRC was correlated with an advanced stage and decreased survival (56). Animal experiments showed that IL-6 promoted the proliferation of CRC cells and the progression of both inflammation-related and sporadic CRCs via the activation of STAT-3 signaling in mice (57, 58). Similarly, IL-17A could indirectly activate STAT-3 through IL-6.
Furthermore, the promoting effect of IL-8 on cancer cell proliferation, invasion, and angiogenesis occurred through activation of the Akt and MAPK signaling pathways (59). The nuclear factor kappa B (NF-κB) signaling pathway is also a key regulator of cancer cell proliferation, progression and metastasis, and activation of NF-κB protein cascade complexes by cytokines has been observed in patients with CRC (60). Somone et al. (61) demonstrated that the stimulatory effect of TH17 cytokines, TNF-α and IL-6, could synergistically activate the NF-κB signaling pathway and then promote CRDC cell growth. Their results also showed that IL-22 and IL-6 could activate STAT-3 signaling.
Interestingly, several studies have revealed that the activation of these signaling pathways contributes to the stemness of CSCs in CRC. For example, persistent action of STAT-3 was correlated with enhanced stemness and proliferation of CRC cells (58). Additionally, aberrant NF-κB signaling has been identified in colon cancer cells, which was regulated by downregulating miR-195-5p/497–5p and upregulating MCM2 (62). These data suggest that the modulatory effect of cytokines on CSCs may occur via the activation of diverse signaling pathways in CSCs, targeting signaling pathways by specific inhibitors may block the effect of cytokines (63).
It must be noted that cytokines with anticancer capacity have been reported. For example, studies have shown that IL-12 produced from mature dendritic cells is a strong inducer of the antitumor-specific immune response (64), and IFN-γ, secreted by T helper (TH)-1 cells, cytotoxic T cells and nature killer cells, plays a key role in the activation of anticancer cellular immunity and the induction of tumor cell apoptosis (65). Studies have revealed that decreased levels of IL-12 and IFN-γ in patients with CRC were associated with CRC progression (47, 65), indicating they have a critical modulatory effect on anticancer immunity.
The potential of cytokine-based immunotherapy in patients with CRC has been intensively evaluated. For example, a study by Ying et al. (66) showed that blocking IL-6 signaling with anti-IL-6 or anti-IL-6 receptor antibodies suppressed STAT-3 signaling pathway and enhanced the efficacy of anticancer drugs in vitro. Furthermore, a phase I/II clinical trial with siltuximab (anti-IL-6 monoclonal antibody) monotherapy that included 32 CRC patients showed that patients tolerated it well at different doses. Studies have also shown the benefits of a combination of anti-PD-1 with anti-IL-17 therapy in microsatellite stable (MSS) CRC (67), and blocking IL-17 signaling may significantly enhance the response to anti-PD1 treatment both in vitro and in vivo (68). In addition, studies have demonstrated that cytokines were associated with resistance to therapies in CRC (69–72). Therefore, targeting these cytokine or receptor signals, e.g., IL-17A, may have significant clinical potential and improve the response to therapeutics and this has been considered a promising immunotherapeutic strategy (68, 73, 74).
Role of cytokines as communicators in mediating crosstalk between CSCs and their niche
Persistent exposure of the niche to proinflammatory cytokines may significantly influence the biological features and functions of CSCs in CRC. In the following paragraphs, we briefly summarize and discuss the significance of cytokines as the main communicators in mediating the interaction between CSCs and their niche.
IL-4
IL-4 is a multifunctional cytokine that plays a critical role in the regulation of host antitumor immunity in diverse types of cancers. Recently, the modulatory effect of IL-4 on CSCs has been identified (75, 76). Research data indicated that IL-4 could potentially stimulate stemness genes and CSC survival, which were associated with cancer metastasis, recurrence, and drug-resistance (77–81). Abrogation of IL-4 signaling with an IL-4Rα antagonist or anti-IL-4 neutralizing antibody could strongly inhibit the survival of colon CSCs and block resistance to chemotherapeutic drugs (82). Interestingly, studies also revealed that IL-4 could function as an autocrine factor to participate in the regulation of CSCs and the induction of drug resistance (82).
IL-6
IL-6 is an inflammatory cytokine produced by several types of cells e.g., T lymphocytes, macrophages, adenoma/CRC cells, and surrounding stromal cells (83). The current scientific evidence suggests that IL-6 and its functional receptor IL-6R are involved in the pathogenesis of CRC development, progression, and metastasis (84, 85). Previous studies have shown that IL-6 can activate STAT-3, the major downstream signaling pathway for IL-6, and it plays a role in the maintenance of CRC cell survival and tumor initiation (86, 87). JAK-STAT signaling is highly active in CSCs, and studies have reported that activation of STAT-3 by IL-6 has a profound effect on tumor initiation and progression, invasion and metastasis by protecting tumor cells from apoptosis, driving epithelial–mesenchymal plasticity, and enhancing angiogenesis (86, 88, 89). In addition, IL-6//STAT-3 is involved in the induction of drug resistance in CRC (86, 88, 89).
Notch is another important oncogenic signaling pathway that contributes to metastasis by inducing epithelial–mesenchymal transition (EMT) in CRC (90). Studies have shown that the activation of Notch1 leads to changes in CSC phenotypes and properties (91, 92). The interplay between IL-6 and Notch has been studied, in which IL-6 was one of the main inducers of the activation of Notch signaling in both MSCs (93) and CSCs (94), and it controbuted to the induction of metastasis and drug resistance (94). Thus, a regulatory effect of IL-6 on CSCs has been postulated. Indeed, Wang et al. (95) reported that IL-6 induced the deacetylation of FRA1 at the Lys-116 residue located within its DNA-binding domain and promoted CRC stem-like properties by affecting transcriptional and posttranslational regulation.
A study by Ying et al. (66) demonstrated that the expression of Notch 3 was significantly upregulated in colon cancer spheroid-forming cells compared with adherent cells, which was upregulated by IL-6 administration, and blocked by an anti-human IL-6R monoclonal antibody. Furthermore, they confirmed that blocking the IL-6 receptor or Notch3 inhibition may be superior to STAT-3 inhibition for CSC-targeting therapies concomitant with anticancer drugs in CRC cell lines (66). Kim et al. (96) found that IL-6 and IL-8 produced by stromal myofibroblasts could expand CSCs by activating Notch/HES1 and STAT-3 pathways in early CRCs, indicating an important role of cytokines in mediating the crosstalk between stromal cells and CRCs. Interestingly, studies have also revealed that the activation of Notch signaling could enhance the production of IL-6 in stromal cells in the TME and then promote tumor cell growth and disease progression (97, 98). The study conducted by Jin et al. (99) indicated that the activation of Notch signaling was mediated by two components of the NF-κB cascade that could upregulate IL-6 expression in breast tumor cells. In turn IL-6 enhanced the activation of Notch again in an autocrine action pathway (100). Other studies reported that IL-6/Notch signals could cooperatively stimulate tumor metastasis and drug-resistance (101, 102). In addition, Wongchana and Palaga (103) found a direct regulatory effect of Notch on IL-6 activation at the transcript level in immune cells, and another study (104) found that IL-6 was also a mediator of crosstalk between fibroblasts and tumor cells in the CRC TME. Taken together, these results strongly suggest that IL-6 is a critical mediator of the interaction between CSCs and their surrounding cells within the TME.
IL-8
Previous studies have suggested that IL-8 is an inflammatory cytokine released from many types of cells, such as macrophages, stromal, endothelial, epithelial and tumor cells, involved in promoting EMT, angiogenesis, tumor growth, metastasis and the immunosuppressive microenvironment in human cancers including CRC (105–112). Previous studies also revealed that a high expression level of IL-8 in human CRC tissues, mediated by IL-8 functional receptors IL-8RA and IL-8RB (43, 110, 113–115), was particularly associated with the properties and features of CSCs (51, 113). Recent studies have confirmed that IL-8 is an of the important modulators of the biological behavior of CSCs e.g., stemness properties that contribute to CRC recurrence and metastasis (42, 113–117). These regulatory effects were found to be mediated via a direct impact on the generation and maintenance of CSCs (42, 113–117) through its functional receptors IL-8RA and IL-8RB expressed in CSCs (43, 110, 113–115). A study by Hwang et al. revealed that the stemness properties of CD44-positive CSCs in CRC were regulated by Snail-IL-8 axis. Results from their works demonstrated a significant correlation between Snail and both IL-8 and CD44 expression; Snail and IL-8 are frequently expressed in CD44-positive CSCs, which have significant stemness properties and more malignant features. The authors further revealed that Snail could directly modulate IL-8 at the transcriptional level. Blocking IL-8 signaling by using shRNA or neutralizing antibody could significantly decrease the expression of stemness genes and reduce drug-resistance. Their findings provided direct evidence to show the modulatory effect of Snail and IL-8 on stem-like properties in CRC.
Luo et al. (118) also demonstrated that mono (2-ethylhexyl) phthalate treatment could remarkably increase the population of CSCs and promote the association of the β-catenin-TCF with the IL-8 promoter, both in cell lines and in mice. Chang et al. (119) demonstrated that Oct4 is a gene involved in the stemness of SCs and it is regulated by cytokines. They reported that IL-8 stimulated the expression of OCT4 and stemness properties in tumor cells (119). Fisher et al. (120) confirmed that targeting IL-8 signaling in the TME could remarkably decrease the tumor volume in mice with xenografts. They further showed that IL-8 stimulation induced a dose-response in in vitro CSCs, and blocking IL-8 or IL-8 receptor signals significantly inhibited cell cycle progression (cyclin D1 and B1) and induced a decreased rate of proliferation and angiogenesis in both in vitro CSCs and in vivo xenograft mice (120). Recently, Shimizu and Tanaka (121) showed that overexpression of IL-8 could induce increased glucose uptake, which was required for the generation and maintenance of CSC characteristics in colon cancer cells.
The effect of IL-8 produced by stromal cells on CSCs was also studied. Kim et al. (96) showed that IL-8 produced from stromal myofibroblasts could expand the population of CSCs by activating hes family bHLH transcription factor 1 in early CRCs, suggesting an interaction between CSCs and stromal cells. Recent evidence has shown that MSCs are an important source of IL-8, and MSC-derived IL-8 levels are even higher than CRC cell-derived IL-8 levels in CRC (122), which stimulates angiogenesis in a paracrine manner (113). Furthermore, the activation of the IL-8 network, modulated by a set of cytokines such as IL-1β (123), in the TME may contribute to the establishment of an immunosuppressive CSC niche (11, 113–115). In a recent study, we showed that the IL-8 niche of CSCs is regulated by IL-1β throughout the colorectal adenoma-carcinoma sequence (123). Interestingly, recent studies also reported (124) that IL-8-derived from CRC cells with high stemness features stimulated the process of angiogenesis. Therefore, this evidence suggests that crosstalk between CSCs and their surrounding cells contributes to tumor angiogenesis and progression, in which IL-8 functions as a key regulator of CSCs in CRC (113).
IL-10
IL-10 is produced by many types of cells, including T cells, monocytes, macrophages, and epithelial cells. IL-10 exhibits a dual role, both promoting and protecting effects, in CRC development and progression. Studies have shown that binding of IL-10 to its receptor IL-10Rα results in the activation of signals e.g., STAT-1, STAT-3, and STAT-5, and then activates selected genes (125). Kang et al. previously reported that IL-10 promoted self-renewal of hematopoietic SCs (126). Recently, Biton et al. (127) found that intestinal SCs (ISCs) expressed several cytokine receptors including the IL-10 receptor IL-10Rα. They reported that both coculture with intestinal regulatory T cells (Tregs) and stimulation with IL-10 led to ISC expansion within organoids, and cells from IL-10-treated organoids had a significantly more “stem-like” pseudotime distribution (127). Their results indicated that IL-10 exhibits modulatory potential on ISC renewal. Since the modulatory mechanisms of cytokines on CSCs are similar to those on ISCs, we postulate that IL-10 may have a modulatory effect on CSCs. Future studies that identify the expression of IL-10Rα in pCSCs/CSCs and the exact effects of IL-10 on pCSCs/CSCs need to be performed.
IL-17A
IL-17 is an inflammatory cytokine that released from numerous types of cells such as TH17 cells, gamma-delta-T cells (γδ/IL17 cells), Tregs, stromal cells, and tumor cells. Extensive studies have shown that IL-17A contributes to the initiation, progression, metastasis, and drug-resistance in diverse types of human cancers (69, 128). More recently, IL-17A has been implicated in the regulation of CSCs in cancers (83, 129). Previously, a study by Sui et al. (69) reported that IL-17A contributed to resistance to the chemotherapeutic drug cisplatin in vivo. Their results showed that IL-17 promoted the viability of HCT116 colorectal cells treated with cisplatin, while blocking IL-17 signaling downregulated apoptosis-related proteins and led to an increased rate of apoptosis in HCT116 colorectal cells.
Furthermore, Lotti et al. (130) found that IL-17A produced by tumor-associated fibroblasts in the CRC TME may drive the growth of CSCs. Their data showed that exogenous IL-17A enhanced CSC self-renewal and invasion, and targeting IL-17A signaling could significantly suppress CSC growth. They reported that fibroblasts in the TME were activated in response to chemotherapy in patients with CRC, which further resulted in increased production of IL-17A from activated fibroblasts and functioned as a mediator for the induction of drug-resistance. Therefore, they suggested that blocking IL-17A signaling may be a potential therapeutic strategy for overcoming drug resistance in CRC.
IL-22
IL-22 is the main cytokine product of TH22 cells (53). The majority of studies showed a positive link between IL-22 and CRC initiation and progression (131–134). Recent scientific evidence suggests that the promoting effect of IL-22 on CRC development occurs via the induction of stemness in tumor cells (135). In line with this hypothesis, Xi et al. (136) demonstrated that IL-22 could stimulate the activation of STAT-3 and then upregulate the expression of PD-1 in human colon cancer cells. Since STAT-3 is highly expressed in CSCs, it is reasonable to speculate about potential modulatory effect of IL-22 on CSCs.
Furthermore, studies have reported that the promoting effect of IL-22 on CRC development occurs through the activation of STAT-3 (137), and neutralizing both IL-22 and STAT-3 signals reduces tumorigenesis in vivo and in vitro (61). Kryczek et al. (138) have previously shown that IL-22 could enhance CRC cell stemness and tumorigenic potential by upregulating core stem cell genes that relied on a STAT-3-dependent pathway, and pretreatment with anti-IL-22 antibody could significantly suppress tumor development and growth in mice after the subcutaneous injection of primary colon cancer cells. Lindemans et al. (139) further confirmed that recombinant IL-22 could directly increase proliferation and promote intestinal SC expansion through the activation of STAT-3 phosphorylation in LGR5-positive SCs and that this process was involved in intestinal epithelial regeneration.
OLFM4 is a member of the olfactomedin domain-containing protein family, and overexpression of OLFM4 has been identified in intestinal SCs (140). Recently, Neyazi et al. (141) reported that overexpression of OLFM4 in the colonic epithelium was modulated by IL-22. By using patient-derived colonic epithelial organoid cultures (EpOCs) model, they were able to show that IL-22 stimulation significantly upregulated the expression of OLFM4. Dame et al. (142) confirmed that OLFM4 in LGR5 positive pCSCs in the colonic adenomatous epithelium was modulated by IL-22. Their findings indicated that pCSCs are regulated by IL-22 in precancerous adenomas. Finally, studies indicated that IL-22 might also be implicated in drug resistance in both CRC patients (143) and CRC cell lines (144). Additionally, studies that focus on the contributory mechanisms of IL-22 to drug resistance in CRC are necessary.
IL-23
IL-23 is an inflammatory cytokine mainly produced by TH cells, macrophages, and dendritic cells. In patients with adenoma and CRC, increased IL-23 has been detected and may function as an upstream factor to regulate the production of IL-17A from TH17 cells (145). A study by Wang et al. (146) showed that IL-23 stimulated the self-renewal of CD133 positive CSCs in ovarian cancer in an autocrine manner by activating the STAT-3 and NF-κB signaling pathways. In addition, IL-23 can activate stemness-relevant genes, such as the Wnt/Notch signaling pathway in esophageal carcinoma (147) and the STAT-3 signaling pathway in gastric cancer (148). Furthermore, a study by Chan et al. (149) showed that IL-23 was sufficient to induce colorectal tumorigenesis. Suzuki et al. (150) reported that IL-23 could directly stimulate the proliferative and invasive activities of CRC cells, and Zhang et al. (151) reported a selective effect of IL-23 on CRC metastasis both in vitro and in vivo; thus, all this evidence may suggest a direct or indirect effect of IL-23 on CSCs.
IL-33
As reviewed by us and others, the contribution of IL-33 to the development and progression of CRC has been intensively studied (54, 152). Considerable evidence suggests that IL-33 promotes the development of CRC through several mechanisms, such as stromal remodeling, proangiogenesis, the induction of other protumor factors and immunosuppression (54). The modulatory effect of IL-33 on the properties of SCs has been reported. For example, Huang et al. (153) reported that hematopoietic SC regeneration after radiation injury could be stimulated by IL-33. Taniguchi and colleagues (154) reported that the formation of an IL-33–TGF-β niche was vital for the promoting effect of CSCs on carcinogenesis in a mouse model of squamous cell carcinoma, in which IL-33 stimulated the differentiation of macrophages and then sent TGF-β signals to CSCs to induce invasion and drug-resistance. Zhang et al.’s study showed that the serum level of IL-33 may serve as a predicator of cetuximab treatment efficacy in patients with CRC (72).
Regarding the role of IL-33 in colorectal adenomas, IL-33 signaling has been reported to promote intestinal polyposis via activation of the tumor stroma (155). More recently, Fang et al. (156) revealed a promoting effect of IL-33 on stemness properties in CRC. They showed that IL-33 significantly activated the core stem cell genes NANOG, NOTCH3, and OCT3/4 via the ST2 signaling pathway, activated the phosphorylation of c-Jun N-terminal kinase (JNK) and enhanced binding of c-Jun to the promoters of the core stem cell genes.
IFN-γ
Several studies have shown that IFN-γ is a critical cytokine regulating CSC survival and stemness features (48, 157, 158). Ni et al. (48) reported that IFN-γ selectively upregulated the apoptosis pathway and promoted the apoptosis process by binding to receptors expressed in colon cancer LGR5-positive CSCs both in vitro and in vivo. In addition, the authors found that the administration of IFN-γ with the chemotherapy agent oxaliplatin resulted in a synergistic anticancer effect (48). Furthermore, a study by Song et al. (159) showed that non-small cell lung cancer-derived cell lines treatment with IFN-γ at a low dose induced a higher proportion of CD133 positive CSCs, and treatment with IFN-γ at a high dose resulted in enhanced apoptosis of tumor cells, indicating a modulatory effect of a low dose of IFN-γ on tumor cell stemness. More recently, Zhuang et al. (160) showed that IFN-γ at a dose of 26.68 ng/mL could remarkably inhibit ALDH1-positive CSCs and decrease the expression of ALDHA1 protein in breast cancer cells. Therefore, IFN-γ might be proposed as a selective anti-CSC cytokine, and modulating the IFN-γ response could function as a targeting strategy for CSCs in cancers (161). Indeed, we have previously found that the expression level of IFN-γ in the adenoma stage was slightly increased and then it was significantly decreased in the CRC stage, implying a selective role of IFN-γ in the management of malignant status along the adenoma-carcinoma sequence (47), in which increased IFN-γ in the adenoma stage maintains pCSCs in a relatively stable state, and decreased IFN-γ level in the CRC stage enhances CSC stemness and malignancy. In the future, the roles and exact mechanisms of IFN-γ as a key factor in modulating pCSCs/CSCs along the adenoma-carcinoma sequence need further exploration.
Despite the complexity of the cytokine network, there are now considerable data suggesting the modulatory effect of cytokines on pCSCs/CSCs during the process of CRC (Table 1). Based on current findings, the possible regulatory mechanisms of protumor cytokines on CSCs are summarized in Figure 1.
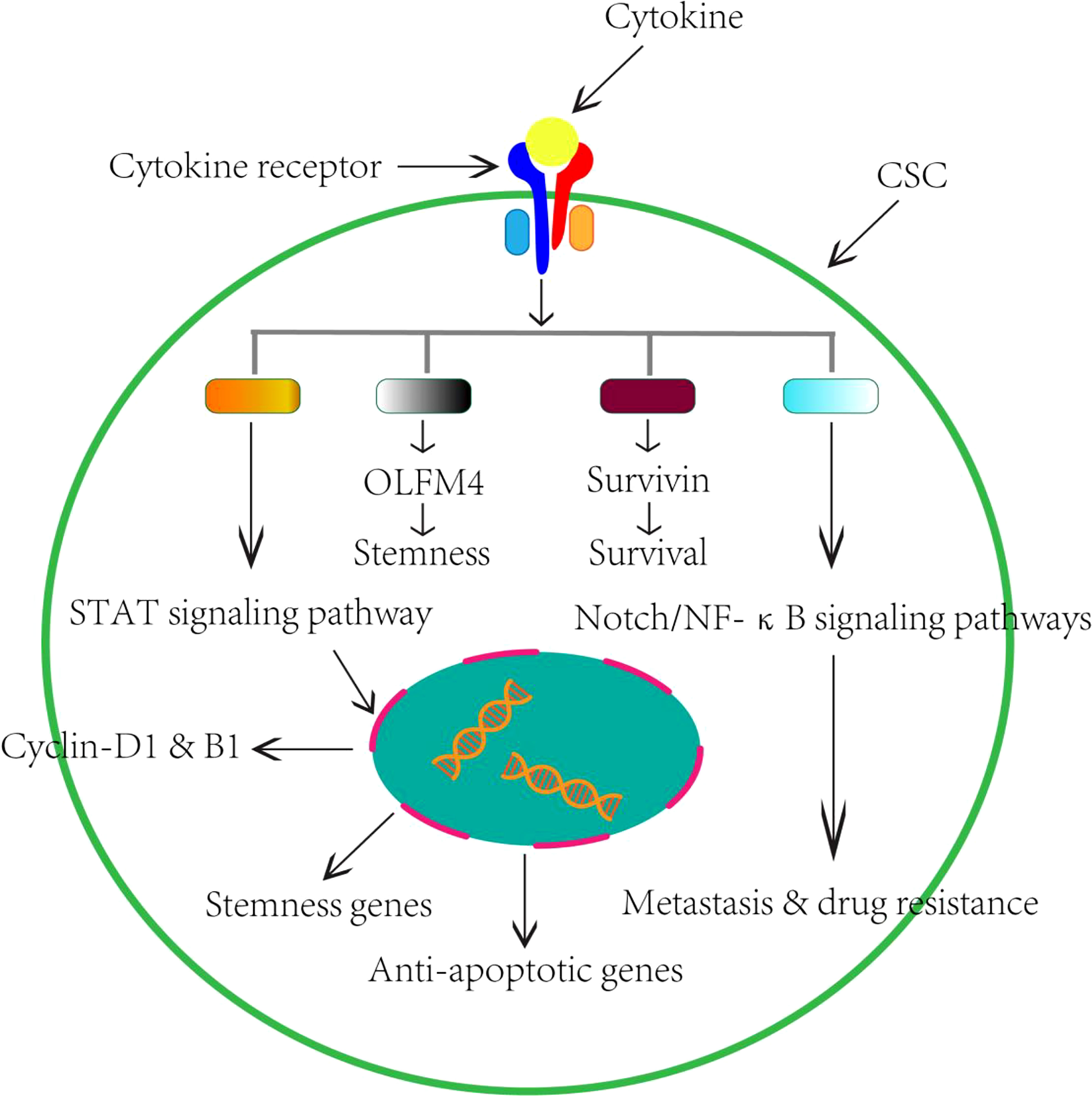
Figure 1 Schematic perspectives of potential role of cytokines on CSCs in the CRC. Based on current research findings, we therefore summarized the regulatory effects of cytokines on CSCs in the CRC.
Clinical implications for CRC development, prevention, and treatment
Could modulation of cytokine signaling affect the function of MSCs?
Published literature indicated that the interaction between MSCs and cytokines might create a favorable milieu to promote tumor angiogenesis, metastasis, drug resistance, and recurrence (122, 162, 163). Recently, we showed that TH17/IL-17A signal activation is associated with dynamic reaction of stromal cells including MSCs throughout the colorectal adenoma-carcinoma sequence (50), which has been verified as a promoting factor contributing to the adenoma and CRC progression (164). Thus, targeting cells or elements, e.g., fibroblasts, macrophages and MSCs and extracellular matrix (ECM) components within the TME, could remodel the CSC niche and this would affect CSC characteristics (165). For example, tumor stromal fibroblast-derived IL-8 stimulates the stemness properties in ovarian cancer cells (166). Similarly, tumor-associated macrophage-derived cytokines, such as transforming growth factor (TGF)-β, tumor necrosis factor (TNF)-α, IL-6 and IL-10, promote CSC transition and characteristics through epithelial–mesenchymal transition (EMT) in diverse types of cancers (167). Shintani et al. (168) reported that fibroblast-derived IL-6 mediated the communication between fibroblasts and cancer cells and contributed to chemoresistance by enhancing EMT in non-small-cell lung carcinoma. In breast cancer, blocking Hedgehog signaling in fibroblasts could restore the sensitivity of resident CSCs to chemotherapy agents (169). Stromal cells can also function as a vehicle to deliver cytokines. Experimental studies have shown that MSCs could be used as a cytokine delivery approach that provides a sustained release of target cytokines in the TME and leads to a more effective antitumor response (170). Therefore, the potential of MSCs as a vehicle for the delivery of bioagents that target cytokine signals into the CSC niche and have a modulatory effect on CSCs should be evaluated.
Are cytokines involved in the modulation of pCSCs/CSCs in colitis-associated CRC?
Previously, Yasuda reported different rates of expression of the stem cell markers CD133, OCT4 and NANOG between CAC and sporadic CRC tissues (171). Iwaya et al. (172) found that the density of CSCs labeled by LGR5 in CAC was lower than that in sporadic CRC. However, Nakagomi et al. more recently reported that increased expression of CSCs is observed in both sporadic CRC (N=23) and CAC (N=22) neoplastic lesions. They found that the expression of CSCs labeled by ALDH1A1 in CAC tissues was higher in those with a longer disease duration than in those with shorter disease duration. CAC exhibits extensive and long-lasting chronic inflammation, and cytokines have been shown to be the most important driving mediators in the induction and maintenance of chronic inflammation (173). These results may imply a key role for cytokines in affecting CSCs in CAC.
Kazama et al. (174) showed that the rate of CD133-positive CSCs in CAC tissues was remarkably higher than that in dysplastic tissues, but the rate of LGR5-positive CSCs was not. Their results may suggest that pCSCs also exist in colitis-associated dysplasia.
Furthermore, their results explained the difference in the CSC-positive rate between different markers in CAC tissues. By using a mouse CAC development model induced by azoxymethane and dextran sodium sulfate treatment, Kim et al. (175) showed that CSCs in crypt base regions detected by RNA in situ hybridization in all dysplasia and CAC samples were increased. Carpentino et al. (24) used fluorescence-activated cell sorting to isolate and identify pCSCs labeled by ALDH from human colitis tissues and their transition to CSCs in both xenografting experiments and in vitro. They demonstrated that pCSCs/CSCs contribute significantly to colorectal carcinogenesis from colitis to CAC (24). Therefore, current scientific evidence suggests the involvement of pCSCs in the development of CAC. The precise modulatory effects of cytokines on pCSCs/CSCs throughout the inflammation-dysplasia-CAC sequence remain unclear, however, a hypothesis has been proposed (176) in which the promoting role of cytokines on pCSCs/CSCs should not be ignored.
How does cytokines modulate pCSCs/CSCs in CRC with a familial adenomatous polyposis background?
Similar to sporadic adenoma polyps, several studies have identified an increased number and enhanced survival of pCSCs in FAP tissues (177, 178) (198). For example, Jennelle et al. (179) showed an increased rate of pCSCs positive for LGR5 in 7 subjects with Lynch syndrome, 4 subjects with FAP and 1 subject with MUTYH-associated polyposis syndrome, which were expressed in colon crypts of subjects with FAP. Ma et al. (180) reported that sulindac treatment of FAP patients might significantly change their pCSC dynamics, indicating that pCSC alterations may be a promising biomarker. However, since FAP is a rare disease, the modulatory effect of cytokines on FAP pCSCs/CSCs remains to be evaluated.
Can selective reprogramming of the pCSC/CSC niche by engineering cellular or inflammatory components affect CRC initiation and progression?
In CRC prevention, one of the most important steps is to reduce the occurrence of precancerous adenomas. Clinically, the management of adenomas in patients relies on colonoscopy, during which the majority of adenomatous polyps can be removed. After the polyps are removed, patients, particularly elderly patients, are advised to undergo regular colonoscopy follow-ups to prevent subsequent CRC (181, 182).
Recent clinical trials have shown that chemoprevention is a promising method to reduce the occurrence of adenomas (183), and nonsteroidal anti-inflammatory drugs (NSAIDs) can significantly reduce the number and size of adenomas in patients with familial adenomatous polyposis (181). However, the effect is incomplete and it is unlikely to replace colonoscopic polypectomy as primary therapy. Furthermore, this NSAID-associated adenomatous polyp reduction cannot decrease the long-term CRC risk. Long-term treatment with NSAIDs may also increase the risks for severe side effects, such as cardiological toxicity (184), renal functional impairment (185) and gastrointestinal mucosa damage (186). For example, approximately 1 to 2% of patients experience a serious gastrointestinal complication during treatment with NSAIDs, which include mucosa severe mucosal damage, ulcers and bleeding (186). Therefore, there is an urgent need to seek novel preventive drugs that are more effective and safer.
It has recently been discussed that therapeutics that target pCSCs combined with their regulatory signals represent an attractive approach. Studies have revealed that pCSCs have the potential for bidirectional differentiation into both benign and malignant lesions, which depends on the interactions between pCSCs and their niche (6). Therefore, how to induce a pCSC to differentiate to a benign direction becomes important. Since cytokines have been reported to be modulators for CSCs, blocking cytokine signals within the niche may have an impact on the differentiation of pCSCs to CSCs and then affect the initiation of precancerous adenomas to CRCs. Several possible strategies need to be experimentally evaluated and addressed. For example, can we use blockades of cytokines combined with selective bioagents targeting pCSCs to modulate the malignant perspectives of a pCSC toward a CSC? Could pCSCs be reversible to SCs? Finally, the efficacy of cytokine signaling blockade combined with CSC targeted therapy in CRC needs to be assessed (refer to Figure 2). In the future, answers to these questions are important for understanding the mechanisms of the progression of a pCSC to a CSC and the identification of novel therapeutics to block this process.
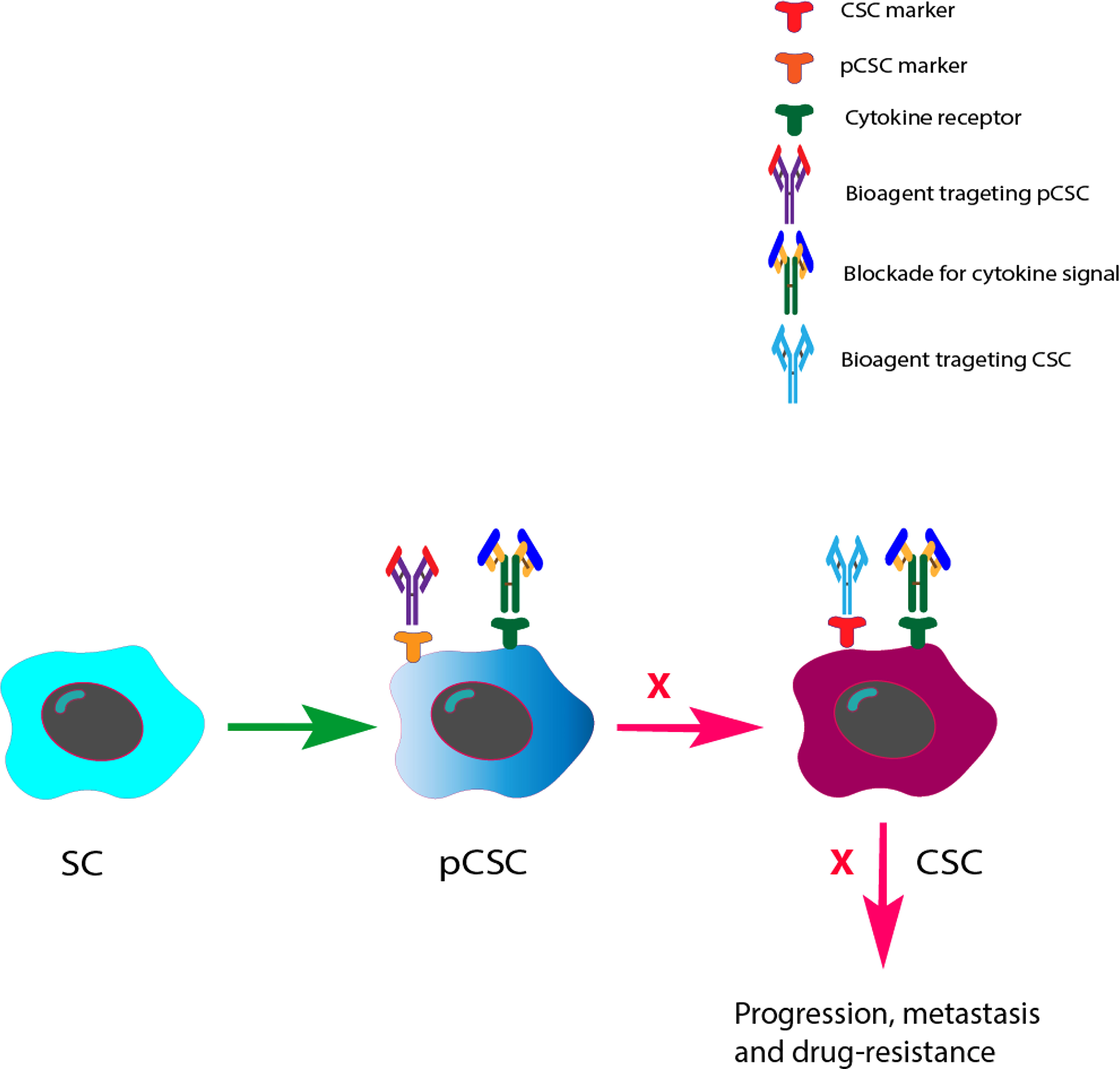
Figure 2 Future perspectives of cytokine signal blockades with pCSC/CSC target therapies in the prevention and inhibition of CRC development and progression. Based on the previous finding that pCSCs have an potential for bidirectional differentiation to both benign and malignant lesions, we therefore prospected that bioagents that can precisely target pCSCs/CSCs with cytokine signals may change both the differentiation direction of pCSCs at the precancerous stage and the effect of CSCs at the CRC stage.
As mentioned above, the biological behavior of pCSCs/CSCs in adenoma/CRC mass is modulated by their niche, which consists of distinct types of cells and factors. Therefore, selective reprogramming of the pCSC/CSC niche by engineering cellular or inflammatory components may affect the biological functions of pCSCs/CSCs (187). Indeed, Park et al. (188) demonstrated that caffeic acid could effectively inhibit self-renewal capacity, stem-like characteristics, and migratory capacity of CD44-positive and CD133-positive CSCs both in vitro and in vivo. Moreover, Park et al. (188) revealed that PI3K/Akt signaling might be linked to multiple CSC-associated characteristics, such as radioresistance, stem-like properties, and tumorigenic potential in CRC. Therefore, signaling pathways that regulate CSC biology and function may be identified as promising CSC targets in the future.
The SC stemness landscape is associated with the response to immunotherapy in CRC (35). Cytokines, as modulators of CSC stemness properties, may also serve as therapeutic targets in CRC. Indeed, previous studies have reported that increased expression levels of cytokines are associated with the initiation of adenoma and CRC in both Apc (Min/+) mice (143) and humans (51, 189), and blocking cytokines or their receptors by the administration of specific antibodies, e.g., anti-IL6 and anti-IL-17A/receptors, effectively suppresses the development and progression of CRC (190, 191). Recently, SC therapies that target CSCs or MSCs have been tested and have shown certain efficacy in established CRCs, which suggests that selectively targeting CSCs is a promising therapeutic strategy to eliminate the development of CRCs and reduce the risk of recurrence (37). Therefore, targeting cytokine signals that modulate CSC differentiation and function might represent an attractive and novel approach to combat CRC and improve the current CSC targeted therapy.
Can targeting cytokine signaling improve drug sensitivity?
Drug-resistance is a major problem for cancer patients treated with chemotherapy. Studies have shown that CSCs function as a major driving force of drug-resistance and they are deeply involved in the pathogenesis of drug resistance (192, 193). Targeting cytokine/receptor signals holds promise for overcoming drug resistance and restoring sensitivity to anticancer therapies (77, 194). For example, IL-4 has been reported to protect CRC CSCs from apoptosis and the potential benefits of standard chemotherapies, radiotherapies or immunotherapies in combination with IL-4 inhibitors may enhance the therapeutic efficacy in the context of CRC (77).
A study by Jiang et al. (194) assessed the efficacy of targeting IL.-8 signaling to overcome drug resistance in advanced gastric cancer, and their results showed that increased expression of IL8 significantly activated drug resistance. IL8 signaling targeted by RNA interference or reparixin reversed chemotherapy resistance with limited toxicity in vivo and in vitro. Targeting CSCs has been recognized as an effective therapeutic for CRC (37). Studies have revealed that the effect of IFN-γ on CSCs was dose-dependent (159). In non-small cell lung cancer-derived cell lines, IFN-γ at a low concentration induced enhanced stemness, and IFN-γ at a high concentration caused apoptosis in CSCs, which could improve the therapeutic efficacy of chemotherapeutic drugs (48, 159). This bidirectional effect of IFN-γ on CSCs may suggest a possible strategy for modulating IFN-γ signaling to selectively inhibit CSC survival and stemness.
However, how to reduce the side effects of cytokine-based immunotherapy is still a challenging issue (195). Most cytokines exhibit a pleiotropic effect and work in a complex network. They play a significant role in immune regulation, antimicrobial infection, and modulating the body’s response to disease. Systematic blockade or enhancement of cytokine signaling may significantly influence host immune homeostasis. In addition, bioagents that target cytokine signals may have their own side effects and drug toxicity (195). Therefore, a better approach for cytokine-based therapy would be the precise targeting of local cytokine signals that can be reached by a selective delivery vehicle (170).
Conclusions
In light of the current knowledge, we may conclude that cytokines function as the main communicators to mediate crosstalk between pCSCs/CSCs and their niche, which is involved in both adenomatous polyp and sporadic CRC onset and progression. Additional studies focused on the pathways of pCSCs/CSCs regulated by cytokines could reserve new perspectives in the field of precision cancer-targeted therapy. In the future, more attention should be given to evaluating the mechanisms of cytokines in regulating the progression of pCSCs to CSCs in adenomas that hold promise for the design of novel therapeutics to prevent the onset of CRC. Finally, pCSCS/CSCs, together with cytokine signals, are attractive potential targets for the treatment of CRC. The therapeutic efficacy of targeting cytokine/receptor signals to inhibit the progression of pCSCs to CSCs is waiting to be evaluated in the future.
Author contributions
GC had the idea for this review and performed the electronic search for literatures, ZW and HL performed literature selection, data extraction and analysis. ZP joined the data analysis and discussion. All authors contributed to the article and approved the submitted version.
Funding
This study was supported by the grants from National Natural Science Foundation of China (81071969) and Medical Research Program, Northern Norway Regional Health Authority (SFP-44-04). The funder did not play any role in paper design, data collection, data analysis, interpretation, writing of the paper.
Conflict of interest
The authors declare that the research was conducted in the absence of any commercial or financial relationships that could be construed as a potential conflict of interest.
Publisher’s note
All claims expressed in this article are solely those of the authors and do not necessarily represent those of their affiliated organizations, or those of the publisher, the editors and the reviewers. Any product that may be evaluated in this article, or claim that may be made by its manufacturer, is not guaranteed or endorsed by the publisher.
Abbreviations
ALDH1, aldehyde dehydrogenase 1; ALDH1A1, aldehyde dehydrogenase isoform 1A1; CRC, colorectal cancer; CSCs, cancer stem cells; ECM, extracellular matrix; EMT, epithelial–mesenchymal transition; EpOCs, epithelial organoid cultures; IFN, interferon; IL, interleukin; ISCs, intestinal stem cells; LGR5, leucine-rich repeat-containing G-protein-coupled receptor 5; MSCs, mesenchymal stem cells; NSAIDs, nonsteroidal anti-inflammatory drugs; NF-κB, nuclear factor Kappa B; OLFM4, olfactomedin-4; pCSCs, precancerous cancer stem cells; SCs, stem cells; STAT, signal transducer and activator of transcription; TGF-β, transforming growth factor-β; TNF-α, tumor necrosis factor-α; Tregs, regulatory T cells.
References
1. Sung H, Ferlay J, Siegel RL, Laversanne M, Soerjomataram I, Jemal A, et al. Global cancer statistics 2020: GLOBOCAN estimates of incidence and mortality worldwide for 36 cancers in 185 countries. CA Cancer J Clin (2021) 71(3):209–49. doi: 10.3322/caac.21660
2. Cui G. Immune battle at the premalignant stage of colorectal cancer: focus on immune cell compositions, functions and cytokine products. Am J Cancer Res (2020) 10(5):1308–20.
3. Conteduca V, Sansonno D, Russi S, Dammacco F. Precancerous colorectal lesions (Review). Int J Oncol (2013) 43(4):973–84. doi: 10.3892/ijo.2013.2041
4. Gao W, Chen L, Ma Z, Du Z, Zhao Z, Hu Z, et al. Isolation and phenotypic characterization of colorectal cancer stem cells with organ-specific metastatic potential. Gastroenterology (2013) 145(3):636–46 e5. doi: 10.1053/j.gastro.2013.05.049
5. Beck B, Blanpain C. Unravelling cancer stem cell potential. Nat Rev Cancer (2013) 13(10):727–38. doi: 10.1038/nrc3597
6. Chen L, Shen R, Ye Y, Pu XA, Liu X, Duan W, et al. Precancerous stem cells have the potential for both benign and malignant differentiation. PloS One (2007) 2(3):e293. doi: 10.1371/journal.pone.0000293
7. Lin CC, Liao TT, Yang MH. Immune adaptation of colorectal cancer stem cells and their interaction with the tumor microenvironment. Front Oncol (2020) 10:588542. doi: 10.3389/fonc.2020.588542
8. Muller L, Tunger A, Plesca I, Wehner R, Temme A, Westphal D, et al. Bidirectional crosstalk between cancer stem cells and immune cell subsets. Front Immunol (2020) 11:140. doi: 10.3389/fimmu.2020.00140
9. Borowczak J, Szczerbowski K, Maniewski M, Kowalewski A, Janiczek-Polewska M, Szylberg A, et al. The role of inflammatory cytokines in the pathogenesis of colorectal carcinoma-recent findings and review. Biomedicines (2022) 10(7):1670. doi: 10.3390/biomedicines10071670
10. Chen Y, Ye Z, Seidler U, Tian D, Xiao F. Microenvironmental regulation of intestinal stem cells in the inflamed intestine. Life Sci (2021) 273:119298. doi: 10.1016/j.lfs.2021.119298
11. Korkaya H, Liu S, Wicha MS. Regulation of cancer stem cells by cytokine networks: attacking cancer's inflammatory roots. Clin Cancer Res (2011) 17(19):6125–9. doi: 10.1158/1078-0432.CCR-10-2743
12. Gao JX. Cancer stem cells: The lessons from pre-cancerous stem cells. J Cell Mol Med (2008) 12(1):67–96. doi: 10.1111/j.1582-4934.2007.00170.x
13. Jang BG, Kim HS, Kim KJ, Rhee YY, Kim WH, Kang GH. Distribution of intestinal stem cell markers in colorectal precancerous lesions. Histopathology (2016) 68(4):567–77. doi: 10.1111/his.12787
14. Baker AM, Graham TA, Elia G, Wright NA, Rodriguez-Justo M. Characterization of LGR5 stem cells in colorectal adenomas and carcinomas. Sci Rep (2015) 5:8654. doi: 10.1038/srep08654
15. Bartley AN, Parikh N, Hsu CH, Roe DJ, Buckmeier JA, Corley L, et al. Colorectal adenoma stem-like cell populations: associations with adenoma characteristics and metachronous colorectal neoplasia. Cancer Prev Res (Phila) (2013) 6(11):1162–70. doi: 10.1158/1940-6207.CAPR-13-0113
16. Humphries A, Cereser B, Gay LJ, Miller DS, Das B, Gutteridge A, et al. Lineage tracing reveals multipotent stem cells maintain human adenomas and the pattern of clonal expansion in tumor evolution. Proc Natl Acad Sci U.S.A. (2013) 110(27):E2490–9. doi: 10.1073/pnas.1220353110
17. Cui G, Xu G, Zhu L, Pang Z, Zheng W, Li Z, et al. Temporal and spatial changes of cells positive for stem-like markers in different compartments and stages of human colorectal adenoma-carcinoma sequence. Oncotarget (2017) 8(28):45311–22. doi: 10.18632/oncotarget.17330
18. Lee JH, Schutte D, Wulf G, Fuzesi L, Radzun HJ, Schweyer S, et al. Stem-cell protein Piwil2 is widely expressed in tumors and inhibits apoptosis through activation of Stat3/Bcl-XL pathway. Hum Mol Genet (2006) 15(2):201–11. doi: 10.1093/hmg/ddi430
19. Ye Y, Yin DT, Chen L, Zhou Q, Shen R, He G, et al. Identification of Piwil2-like (PL2L) proteins that promote tumorigenesis. PloS One (2010) 5(10):e13406. doi: 10.1371/journal.pone.0013406
20. Wang S, Li F, Fan H, Xu J, Hu Z. Expression of PIWIL2 in oral cancer and leukoplakia: Prognostic implications and insights from tumors. Cancer biomark (2019) 26(1):11–20. doi: 10.3233/CBM-182009
21. Liu JJ, Shen R, Chen L, Ye Y, He G, Hua K, et al. Piwil2 is expressed in various stages of breast cancers and has the potential to be used as a novel biomarker. Int J Clin Exp Pathol (2010) 3(4):328–37.
22. Oh SJ, Kim SM, Kim YO, Chang HK. Clinicopathologic implications of PIWIL2 expression in colorectal cancer. Korean J Pathol (2012) 46(4):318–23. doi: 10.4132/KoreanJPathol.2012.46.4.318
23. He G, Chen L, Ye Y, Xiao Y, Hua K, Jarjoura D, et al. Piwil2 expressed in various stages of cervical neoplasia is a potential complementary marker for p16. Am J Transl Res (2010) 2(2):156–69.
24. Carpentino JE, Hynes MJ, Appelman HD, Zheng T, Steindler DA, Scott EW, et al. Aldehyde dehydrogenase-expressing colon stem cells contribute to tumorigenesis in the transition from colitis to cancer. Cancer Res (2009) 69(20):8208–15. doi: 10.1158/0008-5472.CAN-09-1132
25. Rassouli FB, Matin MM, Saeinasab M. Cancer stem cells in human digestive tract malignancies. Tumour Biol (2016) 37(1):7–21. doi: 10.1007/s13277-015-4155-y
26. Zhou F, Mu YD, Liang J, Liu ZX, Zhou D, Ning WL, et al. Aldehyde dehydrogenase 1: a specific cancer stem cell marker for human colorectal carcinoma. Mol Med Rep (2015) 11(5):3894–9. doi: 10.3892/mmr.2015.3195
27. Hong I, Hong SW, Chang YG, Lee WY, Lee B, Kang YK, et al. Expression of the cancer stem cell markers CD44 and CD133 in colorectal cancer: An immunohistochemical staining analysis. Ann Coloproctol (2015) 31(3):84–91. doi: 10.3393/ac.2015.31.3.84
28. Fan LF, Dong WG, Jiang CQ, Xia D, Liao F, Yu QF. Expression of putative stem cell genes musashi-1 and beta1-integrin in human colorectal adenomas and adenocarcinomas. Int J Colorectal Dis (2010) 25(1):17–23. doi: 10.1007/s00384-009-0791-2
29. Dalerba P, Dylla SJ, Park IK, Liu R, Wang X, Cho RW, et al. Phenotypic characterization of human colorectal cancer stem cells. Proc Natl Acad Sci U.S.A. (2007) 104(24):10158–63. doi: 10.1073/pnas.0703478104
30. Kalantari E, Taheri T, Fata S, Abolhasani M, Mehrazma M, Madjd Z, et al. Significant co-expression of putative cancer stem cell markers, EpCAM and CD166, correlates with tumor stage and invasive behavior in colorectal cancer. World J Surg Oncol (2022) 20(1):15. doi: 10.1186/s12957-021-02469-y
31. Leedham SJ, Wright NA. Expansion of a mutated clone: from stem cell to tumour. J Clin Pathol (2008) 61(2):164–71. doi: 10.1136/jcp.2006.044610
32. Quante M, Wang TC. Stem cells in gastroenterology and hepatology. Nat Rev Gastroenterol Hepatol (2009) 6(12):724–37. doi: 10.1038/nrgastro.2009.195
33. Hervieu C, Christou N, Battu S, Mathonnet M. The role of cancer stem cells in colorectal cancer: From the basics to novel clinical trials. Cancers (Basel) (2021) 13(5):1092. doi: 10.3390/cancers13051092
34. Gonzalez-Villarreal CA, Quiroz-Reyes AG, Islas JF, Garza-Trevino EN. Colorectal cancer stem cells in the progression to liver metastasis. Front Oncol (2020) 10:1511. doi: 10.3389/fonc.2020.01511
35. Zheng H, Liu H, Li H, Dou W, Wang J, Zhang J, et al. Characterization of stem cell landscape and identification of stemness-relevant prognostic gene signature to aid immunotherapy in colorectal cancer. Stem Cell Res Ther (2022) 13(1):244. doi: 10.1186/s13287-022-02913-0
36. Codony-Servat J, Rosell R. Cancer stem cells and immunoresistance: clinical implications and solutions. Trans Lung Cancer Res (2015) 4(6):689–703.
37. Ma YS, Li W, Liu Y, Shi Y, Lin QL, Fu D. Targeting colorectal cancer stem cells as an effective treatment for colorectal cancer. Technol Cancer Res Treat (2020) 19:1533033819892261. doi: 10.1177/1533033819892261
38. Frank MH, Wilson BJ, Gold JS, Frank NY. Clinical implications of colorectal cancer stem cells in the age of single-cell omics and targeted therapies. Gastroenterology (2021) 160(6):1947–60. doi: 10.1053/j.gastro.2020.12.080
39. Andrews C, McLean MH, Durum SK. Cytokine tuning of intestinal epithelial function. Front Immunol (2018) 9:1270. doi: 10.3389/fimmu.2018.01270
40. Wang Y, Kim R, Hwang SJ, Dutton J, Sims CE, Allbritton NL. Analysis of interleukin 8 secretion by a stem-Cell-Derived human-Intestinal-Epithelial-Monolayer platform. Anal Chem (2018) 90(19):11523–30. doi: 10.1021/acs.analchem.8b02835
41. Mager LF, Wasmer MH, Rau TT, Krebs P. Cytokine-induced modulation of colorectal cancer. Front Oncol (2016) 6:96. doi: 10.3389/fonc.2016.00096
42. Singh JK, Simoes BM, Clarke RB, Bundred NJ. Targeting IL-8 signalling to inhibit breast cancer stem cell activity. Expert Opin Ther Targets (2013) 17(11):1235–41. doi: 10.1517/14728222.2013.835398
43. Ning Y, Lenz HJ. Targeting IL-8 in colorectal cancer. Expert Opin Ther Targets (2012) 16(5):491–7. doi: 10.1517/14728222.2012.677440
44. Bayik D, Lathia JD. Cancer stem cell-immune cell crosstalk in tumour progression. Nat Rev Cancer (2021) 21(8):526–36. doi: 10.1038/s41568-021-00366-w
45. Ciardiello C, Leone A, Budillon A. The crosstalk between cancer stem cells and microenvironment is critical for solid tumor progression: The significant contribution of extracellular vesicles. Stem Cells Int (2018) 2018:6392198. doi: 10.1155/2018/6392198
46. Dutta A, Sengupta D, Paul S, Chakraborty S, Mukherjee S, Das T. A new insight into tumour immune-evasion: Crosstalk between cancer stem cells and T regulatory cells. Ann Oncol (2019) 30 (Suppl. 9):IX113.
47. Cui G, Goll R, Olsen T, Steigen SE, Husebekk A, Vonen B, et al. Reduced expression of microenvironmental Th1 cytokines accompanies adenomas-carcinomas sequence of colorectum. Cancer Immunol Immunother (2007) 56(7):985–95.
48. Ni C, Wu P, Zhu X, Ye J, Zhang Z, Chen Z, et al. IFN-gamma selectively exerts pro-apoptotic effects on tumor-initiating label-retaining colon cancer cells. Cancer Lett (2013) 336(1):174–84. doi: 10.1016/j.canlet.2013.04.029
49. Cui G, Yuan A, Sun Z, Zheng W, Pang Z. IL-1β/IL-6 network in the tumor microenvironment of human colorectal cancer. Pathol - Res Pract (2018) 214(7):986–92. doi: 10.1016/j.prp.2018.05.011
50. Cui G, Li Z, Florholmen J, Goll R. Dynamic stromal cellular reaction throughout human colorectal adenoma-carcinoma sequence: A role of TH17/IL-17A. BioMed Pharmacother (2021) 140:111761. doi: 10.1016/j.biopha.2021.111761
51. Cui G, Yuan A, Goll R, Vonen B, Florholmen J. Dynamic changes of interleukin-8 network along the colorectal adenoma-carcinoma sequence. Cancer Immunol Immunother (2009) 58(11):1897–905. doi: 10.1007/s00262-009-0702-y
52. Cui G, Qi H, Gundersen MD, Yang H, Christiansen I, Sorbye SW, et al. Dynamics of the IL-33/ST2 network in the progression of human colorectal adenoma to sporadic colorectal cancer. Cancer Immunol Immunother (2015) 64(2):181–90. doi: 10.1007/s00262-014-1624-x
53. Cui G. TH9, TH17, and TH22 cell subsets and their main cytokine products in the pathogenesis of colorectal cancer. Front Oncol (2019) 9:1002. doi: 10.3389/fonc.2019.01002
54. Cui G, Yuan A, Pang Z, Zheng W, Li Z, Goll R. Contribution of IL-33 to the pathogenesis of colorectal cancer. Front Oncol (2018) 8:561. doi: 10.3389/fonc.2018.00561
55. Khaled WT, Read EK, Nicholson SE, Baxter FO, Brennan AJ, Came PJ, et al. The IL-4/IL-13/Stat6 signalling pathway promotes luminal mammary epithelial cell development. Development (2007) 134(15):2739–50. doi: 10.1242/dev.003194
56. Wang CG, Ye YJ, Yuan J, Liu FF, Zhang H, Wang S. EZH2 and STAT6 expression profiles are correlated with colorectal cancer stage and prognosis. World J Gastroenterol (2010) 16(19):2421–7. doi: 10.3748/wjg.v16.i19.2421
57. Grivennikov S, Karin E, Terzic J, Mucida D, Yu GY, Vallabhapurapu S, et al. IL-6 and Stat3 are required for survival of intestinal epithelial cells and development of colitis-associated cancer. Cancer Cell (2009) 15(2):103–13. doi: 10.1016/j.ccr.2009.01.001
58. Corvinus FM, Orth C, Moriggl R, Tsareva SA, Wagner S, Pfitzner EB, et al. Persistent STAT3 activation in colon cancer is associated with enhanced cell proliferation and tumor growth. Neoplasia (2005) 7(6):545–55. doi: 10.1593/neo.04571
59. Waugh DJ, Wilson C. The interleukin-8 pathway in cancer. Clin Cancer Res an Off J Am Assoc Cancer Res (2008) 14(21):6735–41. doi: 10.1158/1078-0432.CCR-07-4843
60. Soleimani A, Rahmani F, Ferns GA, Ryzhikov M, Avan A, Hassanian SM. Role of the NF-kappaB signaling pathway in the pathogenesis of colorectal cancer. Gene (2020) 726:144132. doi: 10.1016/j.gene.2019.144132
61. De Simone V, Franze E, Ronchetti G, Colantoni A, Fantini MC, Di Fusco D, et al. Th17-type cytokines, IL-6 and TNF-alpha synergistically activate STAT3 and NF-kB to promote colorectal cancer cell growth. Oncogene (2015) 34(27):3493–503. doi: 10.1038/onc.2014.286
62. Wang L, Guo J, Zhou J, Wang D, Kang X, Zhou L. NF-kappaB maintains the stemness of colon cancer cells by downregulating miR-195-5p/497-5p and upregulating MCM2. J Exp Clin Cancer Res (2020) 39(1):225. doi: 10.1186/s13046-020-01704-w
63. Kaltschmidt B, Witte KE, Greiner JFW, Weissinger F, Kaltschmidt C. Targeting NF-kappaB signaling in cancer stem cells: A narrative review. Biomedicines (2022) 10(2). doi: 10.3390/biomedicines10020261
64. Nishimura T, Nakui M, Sato M, Iwakabe K, Kitamura H, Sekimoto M, et al. The critical role of Th1-dominant immunity in tumor immunology. Cancer Chemother Pharmacol (2000) 46 Suppl:S52–61. doi: 10.1007/pl00014051
65. Jorgovanovic D, Song M, Wang L, Zhang Y. Roles of IFN-gamma in tumor progression and regression: a review. biomark Res (2020) 8(49):261. doi: 10.1186/s40364-020-00228-x
66. Ying J, Tsujii M, Kondo J, Hayashi Y, Kato M, Akasaka T, et al. The effectiveness of an anti-human IL-6 receptor monoclonal antibody combined with chemotherapy to target colon cancer stem-like cells. Int J Oncol (2015) 46(4):1551–9. doi: 10.3892/ijo.2015.2851
67. Li S, Na R, Li X, Zhang Y, Zheng T. Targeting interleukin-17 enhances tumor response to immune checkpoint inhibitors in colorectal cancer. Biochim Biophys Acta Rev Cancer (2022) 1877(4):188758. doi: 10.1016/j.bbcan.2022.188758
68. Liu C, Liu R, Wang B, Lian J, Yao Y, Sun H, et al. Blocking IL-17A enhances tumor response to anti-PD-1 immunotherapy in microsatellite stable colorectal cancer. J Immunother Cancer (2021) 9(1):e001895. doi: 10.1136/jitc-2020-001895
69. Sui G, Qiu Y, Yu H, Kong Q, Zhen B. Interleukin-17 promotes the development of cisplatin resistance in colorectal cancer. Oncol Lett (2019) 17(1):944–50. doi: 10.3892/ol.2018.9645
70. Du J, He Y, Li P, Wu W, Chen Y, Ruan H. IL-8 regulates the doxorubicin resistance of colorectal cancer cells via modulation of multidrug resistance 1 (MDR1). Cancer Chemother Pharmacol (2018) 81(6):1111–9. doi: 10.1007/s00280-018-3584-x
71. Burz C, Bojan A, Balacescu L, Pop VV, Silaghi C, Lupan I, et al. Interleukin 8 as predictive factor for response to chemotherapy in colorectal cancer patients. Acta Clin Belg (2021) 76(2):113–8. doi: 10.1080/17843286.2019.1680133
72. Zhang X, Bi K, Tu X, Zhang Q, Cao Q, Liang Y, et al. Interleukin-33 as an early predictor of cetuximab treatment efficacy in patients with colorectal cancer. Cancer Med (2021) 10(23):8338–51. doi: 10.1002/cam4.4331
73. Ibrahim S, Girault A, Ohresser M, Lereclus E, Paintaud G, Lecomte T, et al. Monoclonal antibodies targeting the IL-17/IL-17RA axis: An opportunity to improve the efficiency of anti-VEGF therapy in fighting metastatic colorectal cancer? Clin Colorectal Cancer (2018) 17(1):e109–e13. doi: 10.1016/j.clcc.2017.10.003
74. Van der Jeught K, Sun Y, Fang Y, Zhou Z, Jiang H, Yu T, et al. ST2 as checkpoint target for colorectal cancer immunotherapy. JCI Insight (2020) 5(9):e136073. doi: 10.1172/jci.insight.136073
75. Nappo G, Handle F, Santer FR, McNeill RV, Seed RI, Collins AT, et al. The immunosuppressive cytokine interleukin-4 increases the clonogenic potential of prostate stem-like cells by activation of STAT6 signalling. Oncogenesis (2017) 6(5):e342. doi: 10.1038/oncsis.2017.23
76. Di Stefano AB, Iovino F, Lombardo Y, Eterno V, Hoger T, Dieli F, et al. Survivin is regulated by interleukin-4 in colon cancer stem cells. J Cell Physiol (2010) 225(2):555–61. doi: 10.1002/jcp.22238
77. Todaro M, Perez Alea M, Scopelliti A, Medema JP, Stassi G. IL-4-mediated drug resistance in colon cancer stem cells. Cell Cycle (2008) 7(3):309–13. doi: 10.4161/cc.7.3.5389
78. Francipane MG, Alea MP, Lombardo Y, Todaro M, Medema JP, Stassi G. Crucial role of interleukin-4 in the survival of colon cancer stem cells. Cancer Res (2008) 68(11):4022–5. doi: 10.1158/0008-5472.CAN-07-6874
79. Conticello C, Pedini F, Zeuner A, Patti M, Zerilli M, Stassi G, et al. IL-4 protects tumor cells from anti-CD95 and chemotherapeutic agents via up-regulation of antiapoptotic proteins. J Immunol (2004) 172(9):5467–77. doi: 10.4049/jimmunol.172.9.5467
80. Todaro M, Zerilli M, Ricci-Vitiani L, Bini M, Perez Alea M, Maria Florena A, et al. Autocrine production of interleukin-4 and interleukin-10 is required for survival and growth of thyroid cancer cells. Cancer Res (2006) 66(3):1491–9. doi: 10.1158/0008-5472.CAN-05-2514
81. Li Z, Jiang J, Wang Z, Zhang J, Xiao M, Wang C, et al. Endogenous interleukin-4 promotes tumor development by increasing tumor cell resistance to apoptosis. Cancer Res (2008) 68(21):8687–94. doi: 10.1158/0008-5472.CAN-08-0449
82. Todaro M, Alea MP, Di Stefano AB, Cammareri P, Vermeulen L, Iovino F, et al. Colon cancer stem cells dictate tumor growth and resist cell death by production of interleukin-4. Cell Stem Cell (2007) 1(4):389–402. doi: 10.1016/j.stem.2007.08.001
83. Huynh PT, Beswick EJ, Coronado YA, Johnson P, O'Connell MR, Watts T, et al. CD90(+) stromal cells are the major source of IL-6, which supports cancer stem-like cells and inflammation in colorectal cancer. Int J Cancer (2016) 138(8):1971–81. doi: 10.1002/ijc.29939
84. Turano M, Cammarota F, Duraturo F, Izzo P, De Rosa M. A potential role of IL-6/IL-6R in the development and management of colon cancer. Membranes (Basel) (2021) 11(5):312. doi: 10.3390/membranes11050312
85. Sun Q, Shang Y, Sun F, Dong X, Niu J, Li F. Interleukin-6 promotes epithelial-mesenchymal transition and cell invasion through integrin beta6 upregulation in colorectal cancer. Oxid Med Cell Longev (2020) 2020:8032187. doi: 10.1155/2020/8032187
86. Grivennikov S, Karin M. Autocrine IL-6 signaling: a key event in tumorigenesis? Cancer Cell (2008) 13(1):7–9. doi: 10.1016/j.ccr.2007.12.020
87. Lin L, Fuchs J, Li C, Olson V, Bekaii-Saab T, Lin J. STAT3 signaling pathway is necessary for cell survival and tumorsphere forming capacity in ALDH(+)/CD133(+) stem cell-like human colon cancer cells. Biochem Biophys Res Commun (2011) 416(3-4):246–51. doi: 10.1016/j.bbrc.2011.10.112
88. Lin Y, He Z, Ye J, Liu Z, She X, Gao X, et al. Progress in understanding the IL-6/STAT3 pathway in colorectal cancer. Onco Targets Ther (2020) 13:13023–32. doi: 10.2147/OTT.S278013
89. Rokavec M, Oner MG, Li H, Jackstadt R, Jiang L, Lodygin D, et al. IL-6R/STAT3/miR-34a feedback loop promotes EMT-mediated colorectal cancer invasion and metastasis. J Clin Invest (2014) 124(4):1853–67. doi: 10.1172/JCI73531
90. Tyagi A, Sharma AK, Damodaran C. A review on notch signaling and colorectal cancer. Cells (2020) 9(6):1549. doi: 10.3390/cells9061549
91. Fan X, Matsui W, Khaki L, Stearns D, Chun J, Li YM, et al. Notch pathway inhibition depletes stem-like cells and blocks engraftment in embryonal brain tumors. Cancer Res (2006) 66(15):7445–52. doi: 10.1158/0008-5472.CAN-06-0858
92. Fender AW, Nutter JM, Fitzgerald TL, Bertrand FE, Sigounas G. Notch-1 promotes stemness and epithelial to mesenchymal transition in colorectal cancer. J Cell Biochem (2015) 116(11):2517–27. doi: 10.1002/jcb.25196
93. Lin JT, Wang JY, Chen MK, Chen HC, Chang TH, Su BW, et al. Colon cancer mesenchymal stem cells modulate the tumorigenicity of colon cancer through interleukin 6. Exp Cell Res (2013) 319(14):2216–29. doi: 10.1016/j.yexcr.2013.06.003
94. Sansone P, Ceccarelli C, Berishaj M, Chang Q, Rajasekhar VK, Perna F, et al. Self-renewal of CD133(hi) cells by IL6/Notch3 signalling regulates endocrine resistance in metastatic breast cancer. Nat Commun (2016) 7:10442. doi: 10.1038/ncomms10442
95. Wang T, Song P, Zhong T, Wang X, Xiang X, Liu Q, et al. The inflammatory cytokine IL-6 induces FRA1 deacetylation promoting colorectal cancer stem-like properties. Oncogene (2019) 38(25):4932–47. doi: 10.1038/s41388-019-0763-0
96. Kim B, Seo Y, Kwon JH, Shin Y, Kim S, Park SJ, et al. IL-6 and IL-8, secreted by myofibroblasts in the tumor microenvironment, activate HES1 to expand the cancer stem cell population in early colorectal tumor. Mol Carcinog (2021) 60(3):188–200. doi: 10.1002/mc.23283
97. Colombo M, Mirandola L, Chiriva-Internati M, Basile A, Locati M, Lesma E, et al. Cancer cells exploit notch signaling to redefine a supportive cytokine milieu. Front Immunol (2018) 9:1823. doi: 10.3389/fimmu.2018.01823
98. Colombo M, Galletti S, Bulfamante G, Falleni M, Tosi D, Todoerti K, et al. Multiple myeloma-derived jagged ligands increases autocrine and paracrine interleukin-6 expression in bone marrow niche. Oncotarget (2016) 7(35):56013–29. doi: 10.18632/oncotarget.10820
99. Jin S, Mutvei AP, Chivukula IV, Andersson ER, Ramskold D, Sandberg R, et al. Non-canonical notch signaling activates IL-6/JAK/STAT signaling in breast tumor cells and is controlled by p53 and IKKalpha/IKKbeta. Oncogene (2013) 32(41):4892–902. doi: 10.1038/onc.2012.517
100. Sansone P, Storci G, Giovannini C, Pandolfi S, Pianetti S, Taffurelli M, et al. p66Shc/Notch-3 interplay controls self-renewal and hypoxia survival in human stem/progenitor cells of the mammary gland expanded in vitro as mammospheres. Stem Cells (2007) 25(3):807–15. doi: 10.1634/stemcells.2006-0442
101. Sethi N, Dai X, Winter CG, Kang Y. Tumor-derived JAGGED1 promotes osteolytic bone metastasis of breast cancer by engaging notch signaling in bone cells. Cancer Cell (2011) 19(2):192–205. doi: 10.1016/j.ccr.2010.12.022
102. Koerdel K, Spitzner M, Meyer T, Engels N, Krause F, Gaedcke J, et al. NOTCH activation via gp130/STAT3 signaling confers resistance to chemoradiotherapy. Cancers (Basel) (2021) 13(3):455. doi: 10.3390/cancers13030455
103. Wongchana W, Palaga T. Direct regulation of interleukin-6 expression by notch signaling in macrophages. Cell Mol Immunol (2012) 9(2):155–62. doi: 10.1038/cmi.2011.36
104. Karakasheva TA, Lin EW, Tang Q, Qiao E, Waldron TJ, Soni M, et al. IL-6 mediates cross-talk between tumor cells and activated fibroblasts in the tumor microenvironment. Cancer Res (2018) 78(17):4957–70. doi: 10.1158/0008-5472.CAN-17-2268
105. Li A, Dubey S, Varney ML, Dave BJ, Singh RK. IL-8 directly enhanced endothelial cell survival, proliferation, and matrix metalloproteinases production and regulated angiogenesis. J Immunol (2003) 170(6):3369–76.
106. Li A, Varney ML, Valasek J, Godfrey M, Dave BJ, Singh RK. Autocrine role of interleukin-8 in induction of endothelial cell proliferation, survival, migration and MMP-2 production and angiogenesis. Angiogenesis (2005) 8(1):63–71.
107. Li A, Varney ML, Singh RK. Expression of interleukin 8 and its receptors in human colon carcinoma cells with different metastatic potentials. Clin Cancer Res (2001) 7(10):3298–304.
108. Chung YC, Chang YF. Significance of inflammatory cytokines in the progression of colorectal cancer. Hepatogastroenterology (2003) 50(54):1910–3.
109. Yuan A, Chen JJ, Yao PL, Yang PC. The role of interleukin-8 in cancer cells and microenvironment interaction. Front Biosci (2005) 10:853–65.
110. Asfaha S, Dubeykovskiy AN, Tomita H, Yang X, Stokes S, Shibata W, et al. Mice that express human interleukin-8 have increased mobilization of immature myeloid cells, which exacerbates inflammation and accelerates colon carcinogenesis. Gastroenterology (2013) 144(1):155–66. doi: 10.1053/j.gastro.2012.09.057
111. Ning Y, Manegold PC, Hong YK, Zhang W, Pohl A, Lurje G, et al. Interleukin-8 is associated with proliferation, migration, angiogenesis and chemosensitivity in vitro and in vivo in colon cancer cell line models. Int J Cancer (2011) 128(9):2038–49. doi: 10.1002/ijc.25562
112. Liu Q, Li A, Tian Y, Wu JD, Liu Y, Li T, et al. The CXCL8-CXCR1/2 pathways in cancer. Cytokine Growth Factor Rev (2016) 31:61–71. doi: 10.1016/j.cytogfr.2016.08.002
113. Conciatori F, Bazzichetto C, Falcone I, Ferretti G, Cognetti F, Milella M, et al. Colorectal cancer stem cells properties and features: evidence of interleukin-8 involvement. Cancer Drug Resistance (2019) 2(4):968–79. doi: 10.20517/cdr.2019.56
114. Corro C, Healy ME, Engler S, Bodenmiller B, Li Z, Schraml P, et al. IL-8 and CXCR1 expression is associated with cancer stem cell-like properties of clear cell renal cancer. J Pathol (2019) 248(3):377–89. doi: 10.1002/path.5267
115. Chen L, Fan J, Chen H, Meng Z, Chen Z, Wang P, et al. The IL-8/CXCR1 axis is associated with cancer stem cell-like properties and correlates with clinical prognosis in human pancreatic cancer cases. Sci Rep (2014) 4:5911. doi: 10.1038/srep05911
116. David JM, Dominguez C, Hamilton DH, Palena C. The IL-8/IL-8R axis: A double agent in tumor immune resistance. Vaccines (Basel) (2016) 4(3):22. doi: 10.3390/vaccines4030022
117. Bazzichetto C, Conciatori F, Falcone I, Cognetti F, Milella M, Ciuffreda L. Advances in tumor-stroma interactions: Emerging role of cytokine network in colorectal and pancreatic cancer. J Oncol (2019) 2019:5373580. doi: 10.1155/2019/5373580
118. Luo CW, Hsiao IL, Wang JY, Wu CC, Hung WC, Lin YH, et al. Cell motility facilitated by Mono(2-ethylhexyl) phthalate via activation of the AKT-beta-Catenin-IL-8 axis in colorectal cancer. J Agric Food Chem (2018) 66(37):9635–44. doi: 10.1021/acs.jafc.8b03558
119. Chang CJ, Chien Y, Lu KH, Chang SC, Chou YC, Huang CS, et al. Oct4-related cytokine effects regulate tumorigenic properties of colorectal cancer cells. Biochem Biophys Res Commun (2011) 415(2):245–51. doi: 10.1016/j.bbrc.2011.10.024
120. Fisher RC, Bellamkonda K, Alex Molina L, Xiang S, Liska D, Sarvestani SK, et al. Disrupting inflammation-associated CXCL8-CXCR1 signaling inhibits tumorigenicity initiated by sporadic- and colitis-colon cancer stem cells. Neoplasia (2019) 21(3):269–81. doi: 10.1016/j.neo.2018.12.007
121. Shimizu M, Tanaka N. IL-8-induced O-GlcNAc modification via GLUT3 and GFAT regulates cancer stem cell-like properties in colon and lung cancer cells. Oncogene (2019) 38(9):1520–33. doi: 10.1038/s41388-018-0533-4
122. Wang J, Wang Y, Wang S, Cai J, Shi J, Sui X, et al. Bone marrow-derived mesenchymal stem cell-secreted IL-8 promotes the angiogenesis and growth of colorectal cancer. Oncotarget (2015) 6(40):42825–37. doi: 10.18632/oncotarget.5739
123. Cui G, Li G, Pang Z, Florholmen J, Goll R. The presentation and regulation of the IL-8 network in the epithelial cancer stem-like cell niche in patients with colorectal cancer. BioMed Pharmacother (2022) 152:113252. doi: 10.1016/j.biopha.2022.113252
124. Chung SY, Chao TC, Su Y. The stemness-high human colorectal cancer cells promote angiogenesis by producing higher amounts of angiogenic cytokines via activation of the Egfr/Akt/Nf-kappaB pathway. Int J Mol Sci (2021) 22(3):1355. doi: 10.3390/ijms22031355
125. Wehinger J, Gouilleux F, Groner B, Finke J, Mertelsmann R, Weber-Nordt RM. IL-10 induces DNA binding activity of three STAT proteins (Stat1, Stat3, and Stat5) and their distinct combinatorial assembly in the promoters of selected genes. FEBS Lett (1996) 394(3):365–70. doi: 10.1016/0014-5793(96)00990-8
126. Kang YJ, Yang SJ, Park G, Cho B, Min CK, Kim TY, et al. A novel function of interleukin-10 promoting self-renewal of hematopoietic stem cells. Stem Cells (2007) 25(7):1814–22. doi: 10.1634/stemcells.2007-0002
127. Biton M, Haber AL, Rogel N, Burgin G, Beyaz S, Schnell A, et al. T Helper cell cytokines modulate intestinal stem cell renewal and differentiation. Cell (2018) 175(5):1307–20 e22. doi: 10.1016/j.cell.2018.10.008
128. Zhao J, Chen X, Herjan T, Li X. The role of interleukin-17 in tumor development and progression. J Exp Med (2020) 217(1):e20190297. doi: 10.1084/jem.20190297
129. Zhang Y, Zoltan M, Riquelme E, Xu H, Sahin I, Castro-Pando S, et al. Immune cell production of interleukin 17 induces stem cell features of pancreatic intraepithelial neoplasia cells. Gastroenterology (2018) 155(1):210–23 e3. doi: 10.1053/j.gastro.2018.03.041
130. Lotti F, Jarrar AM, Pai RK, Hitomi M, Lathia J, Mace A, et al. Chemotherapy activates cancer-associated fibroblasts to maintain colorectal cancer-initiating cells by IL-17A. J Exp Med (2013) 210(13):2851–72. doi: 10.1084/jem.20131195
131. Kirchberger S, Royston DJ, Boulard O, Thornton E, Franchini F, Szabady RL, et al. Innate lymphoid cells sustain colon cancer through production of interleukin-22 in a mouse model. J Exp Med (2013) 210(5):917–31. doi: 10.1084/jem.20122308
132. Chen Y, Vandereyken M, Newton IP, Moraga I, Nathke IS, Swamy M. Loss of adenomatous polyposis coli function renders intestinal epithelial cells resistant to the cytokine IL-22. PloS Biol (2019) 17(11):e3000540. doi: 10.1371/journal.pbio.3000540
133. McCuaig S, Barras D, Mann EH, Friedrich M, Bullers SJ, Janney A, et al. The interleukin 22 pathway interacts with mutant KRAS to promote poor prognosis in colon cancer. Clin Cancer Res (2020) 26(16):4313–25. doi: 10.1158/1078-0432.CCR-19-1086
134. Huber S, Gagliani N, Zenewicz LA, Huber FJ, Bosurgi L, Hu B, et al. IL-22BP is regulated by the inflammasome and modulates tumorigenesis in the intestine. Nature (2012) 491(7423):259–63. doi: 10.1038/nature11535
135. Koltsova EK, Grivennikov SI. IL-22 gets to the stem of colorectal cancer. Immunity (2014) 40(5):639–41. doi: 10.1016/j.immuni.2014.04.014
136. Xi X, Hu R, Wang Q, Xu K, Yang H, Cui Z, et al. Interleukin-22 promotes PD-L1 expression via STAT3 in colon cancer cells. Oncol Lett (2021) 22(4):716. doi: 10.3892/ol.2021.12977
137. Jiang R, Wang H, Deng L, Hou J, Shi R, Yao M, et al. IL-22 is related to development of human colon cancer by activation of STAT3. BMC Cancer (2013) 13:59. doi: 10.1186/1471-2407-13-59
138. Kryczek I, Lin Y, Nagarsheth N, Peng D, Zhao L, Zhao E, et al. IL-22(+)CD4(+) T cells promote colorectal cancer stemness via STAT3 transcription factor activation and induction of the methyltransferase DOT1L. Immunity (2014) 40(5):772–84. doi: 10.1016/j.immuni.2014.03.010
139. Lindemans CA, Calafiore M, Mertelsmann AM, O'Connor MH, Dudakov JA, Jenq RR, et al. Interleukin-22 promotes intestinal-stem-cell-mediated epithelial regeneration. Nature (2015) 528(7583):560–4. doi: 10.1038/nature16460
140. Luebbering N, Alder MN, Davies SM. 602 - increased expression of stem cell marker olfactomedin-4 in gastrointestinal graft-Versus-Host disease. Biol Blood Marrow Transplant (2018) 24(3, Supplement):S437. doi: 10.1016/j.bbmt.2017.12.525
141. Neyazi M, Bharadwaj SS, Bullers S, Varenyiova Z, Oxford IBDCSI, Travis S, et al. Overexpression of cancer-associated stem cell gene OLFM4 in the colonic epithelium of patients with primary sclerosing cholangitis. Inflammation Bowel Dis (2021) 27(8):1316–27. doi: 10.1093/ibd/izab025
142. Dame MK, Attili D, McClintock SD, Dedhia PH, Ouillette P, Hardt O, et al. Identification, isolation and characterization of human LGR5-positive colon adenoma cells. Development (2018) 145(6):dev153049. doi: 10.1242/dev.153049
143. Akhmaltdinova L, Sirota V, Babenko D, Zhumaliyeva V, Kadyrova I, Maratkyzy M, et al. Proinflammatory cytokines and colorectal cancer - the impact of the stage. Contemp Oncol (Pozn) (2020) 24(4):207–10. doi: 10.5114/wo.2020.102551
144. Wu T, Wang Z, Liu Y, Mei Z, Wang G, Liang Z, et al. Interleukin 22 protects colorectal cancer cells from chemotherapy by activating the STAT3 pathway and inducing autocrine expression of interleukin 8. Clin Immunol (2014) 154(2):116–26. doi: 10.1016/j.clim.2014.07.005
145. Cui G, Yuan A, Goll R, Florholmen J. IL-17A in the tumor microenvironment of the human colorectal adenoma-carcinoma sequence. Scand J Gastroenterol (2012) 47(11):1304–12. doi: 10.3109/00365521.2012.725089
146. Wang D, Xiang T, Zhao Z, Lin K, Yin P, Jiang L, et al. Autocrine interleukin-23 promotes self-renewal of CD133+ ovarian cancer stem-like cells. Oncotarget (2016) 7(46):76006–20. doi: 10.18632/oncotarget.12579
147. Zhou Y, Su Y, Zhu H, Wang X, Li X, Dai C, et al. Interleukin-23 receptor signaling mediates cancer dormancy and radioresistance in human esophageal squamous carcinoma cells via the Wnt/Notch pathway. J Mol Med (Berl) (2019) 97(2):177–88. doi: 10.1007/s00109-018-1724-8
148. Xu X, Yang C, Chen J, Liu J, Pa Li, Shi Y, et al. Interleukin-23 promotes the migration and invasion of gastric cancer cells by inducing epithelial-to-mesenchymal transition via the STAT3 pathway. Biochem Biophys Res Commun (2018) 499(2):273–8. doi: 10.1016/j.bbrc.2018.03.144
149. Chan IH, Jain R, Tessmer MS, Gorman D, Mangadu R, Sathe M, et al. Interleukin-23 is sufficient to induce rapid de novo gut tumorigenesis, independent of carcinogens, through activation of innate lymphoid cells. Mucosal Immunol (2014) 7(4):842–56. doi: 10.1038/mi.2013.101
150. Suzuki H, Ogawa H, Miura K, Haneda S, Watanabe K, Ohnuma S, et al. IL-23 directly enhances the proliferative and invasive activities of colorectal carcinoma. Oncol Lett (2012) 4(2):199–204. doi: 10.3892/ol.2012.739
151. Zhang L, Li J, Li L, Zhang J, Wang X, Yang C, et al. IL-23 selectively promotes the metastasis of colorectal carcinoma cells with impaired Socs3 expression via the STAT5 pathway. Carcinogenesis (2014) 35(6):1330–40. doi: 10.1093/carcin/bgu017
152. Akimoto M, Takenaga K. Role of the IL-33/ST2L axis in colorectal cancer progression. Cell Immunol (2019) 343:103740. doi: 10.1016/j.cellimm.2017.12.014
153. Huang P, Li X, Meng Y, Yuan B, Liu T, Jiao M, et al. Interleukin-33 regulates hematopoietic stem cell regeneration after radiation injury. Stem Cell Res Ther (2019) 10(1):123. doi: 10.1186/s13287-019-1221-1
154. Taniguchi S, Elhance A, Van Duzer A, Kumar S, Leitenberger JJ, Oshimori N. Tumor-initiating cells establish an IL-33-TGF-beta niche signaling loop to promote cancer progression. Science (2020) 369(6501):eaay1813. doi: 10.1126/science.aay1813
155. Maywald RL, Doerner SK, Pastorelli L, De Salvo C, Benton SM, Dawson EP, et al. IL-33 activates tumor stroma to promote intestinal polyposis. Proc Natl Acad Sci U.S.A. (2015) 112(19):E2487–96. doi: 10.1073/pnas.1422445112
156. Fang M, Li Y, Huang K, Qi S, Zhang J, Zgodzinski W, et al. IL33 promotes colon cancer cell stemness via JNK activation and macrophage recruitment. Cancer Res (2017) 77(10):2735–45. doi: 10.1158/0008-5472.CAN-16-1602
157. Matteucci C, Balestrieri E, Argaw-Denboba A, Sinibaldi-Vallebona P. Human endogenous retroviruses role in cancer cell stemness. Semin Cancer Biol (2018) 53:17–30. doi: 10.1016/j.semcancer.2018.10.001
158. Martin-Hijano L, Sainz B Jr. The interactions between cancer stem cells and the innate interferon signaling pathway. Front Immunol (2020) 11:526. doi: 10.3389/fimmu.2020.00526
159. Song M, Ping Y, Zhang K, Yang L, Li F, Zhang C, et al. Low-dose IFNgamma induces tumor cell stemness in tumor microenvironment of non-small cell lung cancer. Cancer Res (2019) 79(14):3737–48. doi: 10.1158/0008-5472.CAN-19-0596
160. Zhuang X, Shi G, Hu X, Wang H, Sun W, Wu Y. Interferon-gamma inhibits aldehyde dehydrogenasebright cancer stem cells in the 4T1 mouse model of breast cancer. Chin Med J (Engl) (2021) 135(2):194–204. doi: 10.1097/CM9.0000000000001558
161. Huang JL, Chen SY, Lin CS. Targeting cancer stem cells through epigenetic modulation of interferon response. J Pers Med (2022) 12(4):556. doi: 10.3390/jpm12040556
162. Bhat AA, Nisar S, Singh M, Ashraf B, Masoodi T, Prasad CP, et al. Cytokine- and chemokine-induced inflammatory colorectal tumor microenvironment: Emerging avenue for targeted therapy. Cancer Commun (Lond) (2022) 42(8):689–715. doi: 10.1002/cac2.12295
163. Singh G KH. Cytokine regulation of stem cells. PeerJ Preprints (2016) 4:e2347v1. doi: 10.7287/peerj.preprints.2347v1
164. Cui G, Yuan A, Vonen B, Florholmen J. Progressive cellular response in the lamina propria of the colorectal adenoma-carcinoma sequence. Histopathology (2009) 54(5):550–60. doi: 10.1111/j.1365-2559.2009.03273.x
165. Sun HR, Wang S, Yan SC, Zhang Y, Nelson PJ, Jia HL, et al. Therapeutic strategies targeting cancer stem cells and their microenvironment. Front Oncol (2019) 9:1104. doi: 10.3389/fonc.2019.01104
166. Ji Z, Tian W, Gao W, Zang R, Wang H, Yang G. Cancer-associated fibroblast-derived interleukin-8 promotes ovarian cancer cell stemness and malignancy through the Notch3-mediated signaling. Front Cell Dev Biol (2021) 9:684505. doi: 10.3389/fcell.2021.684505
167. Chen Y, Tan W, Wang C. Tumor-associated macrophage-derived cytokines enhance cancer stem-like characteristics through epithelial-mesenchymal transition. Onco Targets Ther (2018) 11:3817–26. doi: 10.2147/OTT.S168317
168. Shintani Y, Fujiwara A, Kimura T, Kawamura T, Funaki S, Minami M, et al. IL-6 secreted from cancer-associated fibroblasts mediates chemoresistance in NSCLC by increasing epithelial-mesenchymal transition signaling. J Thorac Oncol (2016) 11(9):1482–92. doi: 10.1016/j.jtho.2016.05.025
169. Najafi M, Farhood B, Mortezaee K. Cancer stem cells (CSCs) in cancer progression and therapy. J Cell Physiol (2019) 234(6):8381–95. doi: 10.1002/jcp.27740
170. Razeghian E, Margiana R, Chupradit S, Bokov DO, Abdelbasset WK, Marofi F, et al. Mesenchymal Stem/Stromal cells as a vehicle for cytokine delivery: An emerging approach for tumor immunotherapy. Front Med (Lausanne) (2021) 8:721174. doi: 10.3389/fmed.2021.721174
171. Yasuda H, Tanaka K, Okita Y, Araki T, Saigusa S, Toiyama Y, et al. CD133, OCT4, and NANOG in ulcerative colitis-associated colorectal cancer. Oncol Lett (2011) 2(6):1065–71. doi: 10.3892/ol.2011.415
172. Iwaya M, Ota H, Nakajima T, Uehara T, Riddell R, Conner J. Most colitis associated carcinomas lack expression of LGR5: a preliminary study with implications for unique pathways of carcinogenesis compared to sporadic colorectal carcinoma. BMC Cancer (2021) 21(1):119. doi: 10.1186/s12885-021-07835-3
173. Romano M, DEF F, Zarantonello L, Ruffolo C, Ferraro GA, Zanus G, et al. From inflammation to cancer in inflammatory bowel disease: Molecular perspectives. Anticancer Res (2016) 36(4):1447–60.
174. Kazama S, Kishikawa J, Tanaka T, Hata K, Kawai K, Nozawa H, et al. Immunohistochemical expression of CD133 and LGR5 in ulcerative colitis-associated colorectal cancer and dysplasia. In Vivo (2019) 33(4):1279–84. doi: 10.21873/invivo.11600
175. Kim HS, Lee C, Kim WH, Maeng YH, Jang BG. Expression profile of intestinal stem cell markers in colitis-associated carcinogenesis. Sci Rep (2017) 7(1):6533. doi: 10.1038/s41598-017-06900-x
176. Hynes MJ, Huang KM, Huang EH. Review paper: Implications of the "cancer stem cell" hypothesis on murine models of colon cancer and colitis-associated cancer. Vet Pathol (2009) 46(5):819–35. doi: 10.1354/vp.08-VP-0172-H-REV
177. Campbell F, Geraghty JM, Appleton MAC, Williams ED, Williams GT. Increased stem cell somatic mutation in the non-neoplastic colorectal mucosa of patients with familial adenomatous polyposis. Hum Pathol (1998) 29(12):1531–5. doi: 10.1016/S0046-8177(98)90026-0
178. Kim KM, Calabrese P, Tavare S, Shibata D. Enhanced stem cell survival in familial adenomatous polyposis. Am J Pathol (2004) 164(4):1369–77. doi: 10.1016/S0002-9440(10)63223-3
179. Jennelle LT, Dampier CH, Tring S, Powell S, Casey G. Colon crypts of subjects with familial adenomatous polyposis show an increased number of LGR5+ ectopic stem cells. Clin Transl Gastroenterol (2021) 12(5):e00353. doi: 10.14309/ctg.0000000000000353
180. Ma H, Brosens LAA, Elias SG, Morsink FHM, Nijman IJ, Hylind LM, et al. Longitudinal analysis of colon crypt stem cell dynamics in sulindac treated familial adenomatous polyposis patients. Sci Rep (2017) 7(1):11972. doi: 10.1038/s41598-017-11865-y
181. Giardiello FM, Hamilton SR, Krush AJ, Piantadosi S, Hylind LM, Celano P, et al. Treatment of colonic and rectal adenomas with sulindac in familial adenomatous polyposis. N Engl J Med (1993) 328(18):1313–6. doi: 10.1056/NEJM199305063281805
182. Click B, Pinsky PF, Hickey T, Doroudi M, Schoen RE. Association of colonoscopy adenoma findings with long-term colorectal cancer incidence. JAMA (2018) 319(19):2021–31. doi: 10.1001/jama.2018.5809
183. Patel R, Hyer W. Practical management of polyposis syndromes. Frontline Gastroenterol (2019) 10(4):379–87. doi: 10.1136/flgastro-2018-101053
184. Varga Z, Sabzwari SRA, Vargova V. Cardiovascular risk of nonsteroidal anti-inflammatory drugs: An under-recognized public health issue. Cureus (2017) 9(4):e1144. doi: 10.7759/cureus.1144
185. Davis A, Robson J. The dangers of NSAIDs: look both ways. Br J Gen Pract (2016) 66(645):172–3. doi: 10.3399/bjgp16X684433
186. Sostres C, Gargallo CJ, Lanas A. Nonsteroidal anti-inflammatory drugs and upper and lower gastrointestinal mucosal damage. Arthritis Res Ther (2013) 15 Suppl 3:S3. doi: 10.1186/ar4175
187. Shahid A, Bharadwaj M. The connection between the Th17 cell related cytokines and cancer stem cells in cancer: Novel therapeutic targets. Immunol Lett (2019) 213:9–20. doi: 10.1016/j.imlet.2019.07.001
188. Park SR, Kim SR, Hong IS, Lee HY. A novel therapeutic approach for colorectal cancer stem cells: Blocking the PI3K/Akt signaling axis with caffeic acid. Front Cell Dev Biol (2020) 8:585987. doi: 10.3389/fcell.2020.585987
189. Cui G, Yuan A, Li Z, Goll R, Florholmen J. ST2 and regulatory T cells in the colorectal adenoma/carcinoma microenvironment: implications for diseases progression and prognosis. Sci Rep (2020) 10(1):5892. doi: 10.1038/s41598-020-62502-0
190. Qi H, Yang H, Xu G, Ren J, Hua W, Shi Y, et al. Therapeutic efficacy of IL-17A antibody injection in preventing the development of colitis associated carcinogenesis in mice. Immunobiology (2015) 220(1):54–9. doi: 10.1016/j.imbio.2014.09.002
191. Angevin E, Tabernero J, Elez E, Cohen SJ, Bahleda R, van Laethem JL, et al. A phase I/II, multiple-dose, dose-escalation study of siltuximab, an anti-interleukin-6 monoclonal antibody, in patients with advanced solid tumors. Clin Cancer Res (2014) 20(8):2192–204. doi: 10.1158/1078-0432.CCR-13-2200
192. Li Y, Wang Z, Ajani JA, Song S. Drug resistance and cancer stem cells. Cell Commun Signal (2021) 19(1):19. doi: 10.1186/s12964-020-00627-5
193. Yuan Z, Liang X, Zhan Y, Wang Z, Xu J, Qiu Y, et al. Targeting CD133 reverses drug-resistance via the AKT/NF-kappaB/MDR1 pathway in colorectal cancer. Br J Cancer (2020) 122(9):1342–53. doi: 10.1038/s41416-020-0783-0
194. Jiang H, Cui J, Chu H, Xu T, Xie M, Jing X, et al. Targeting IL8 as a sequential therapy strategy to overcome chemotherapy resistance in advanced gastric cancer. Cell Death Discovery (2022) 8(1):235. doi: 10.1038/s41420-022-01033-1
Keywords: cytokine, colorectum, tumorigenesis, stem cell, cancer stem cells
Citation: Cui G, Wang Z, Liu H and Pang Z (2022) Cytokine-mediated crosstalk between cancer stem cells and their inflammatory niche from the colorectal precancerous adenoma stage to the cancerous stage: Mechanisms and clinical implications. Front. Immunol. 13:1057181. doi: 10.3389/fimmu.2022.1057181
Received: 29 September 2022; Accepted: 03 November 2022;
Published: 17 November 2022.
Edited by:
Giovanni Monteleone, University of Rome Tor Vergata, ItalyReviewed by:
Carmine Stolfi, University of Rome Tor Vergata, ItalyIrene Marafini, Policlinico Tor Vergata, Italy
Federica Facciotti, European Institute of Oncology (IEO), Italy
Copyright © 2022 Cui, Wang, Liu and Pang. This is an open-access article distributed under the terms of the Creative Commons Attribution License (CC BY). The use, distribution or reproduction in other forums is permitted, provided the original author(s) and the copyright owner(s) are credited and that the original publication in this journal is cited, in accordance with accepted academic practice. No use, distribution or reproduction is permitted which does not comply with these terms.
*Correspondence: Guanglin Cui, guanglin.cui@nord.no