- 1Faculty of Medicine, University of Southampton, Southampton, United Kingdom
- 2School of Medicine, The University of Notre Dame Australia, Freemantle, WA, Australia
- 3Faculty of Environmental and Life Sciences, University of Southampton, Southampton, United Kingdom
- 4National Institute for Health and Care Research (NIHR) Southampton Biomedical Research Centre, University Hospital Southampton National Health Service (NHS) Foundation Trust and University of Southampton, Southampton, United Kingdom
Background: Obesity is associated with enhanced lipid accumulation and the expansion of adipose tissue accompanied by hypoxia and inflammatory signalling. Investigation in human subcutaneous white adipose tissue (scWAT) in people living with obesity in which metabolic complications such as insulin resistance are yet to manifest is limited, and the mechanisms by which these processes are dysregulated are not well elucidated. Long chain omega-3 polyunsaturated fatty acids (LC n-3 PUFAs) have been shown to modulate the expression of genes associated with lipid accumulation and collagen deposition and reduce the number of inflammatory macrophages in adipose tissue from individuals with insulin resistance. Therefore, these lipids may have positive actions on obesity associated scWAT hypertrophy and inflammation.
Methods: To evaluate obesity-associated tissue remodelling and responses to LC n-3 PUFAs, abdominal scWAT biopsies were collected from normal weight individuals and those living with obesity prior to and following 12-week intervention with marine LC n-3 PUFAs (1.1 g EPA + 0.8 g DHA daily). RNA sequencing, qRT-PCR, and histochemical staining were used to assess remodelling- and inflammatory-associated gene expression, tissue morphology and macrophage infiltration.
Results: Obesity was associated with scWAT hypertrophy (P < 0.001), hypoxia, remodelling, and inflammatory macrophage infiltration (P = 0.023). Furthermore, we highlight the novel dysregulation of Wnt signalling in scWAT in non-insulin resistant obesity. LC n-3 PUFAs beneficially modulated the scWAT environment through downregulating the expression of genes associated with inflammatory and remodelling pathways (P <0.001), but there were altered outcomes in individuals living with obesity in comparison to normal weight individuals.
Conclusion: Our data identify dysregulation of Wnt signalling, hypoxia, and hypertrophy, and enhanced macrophage infiltration in scWAT in non-insulin resistant obesity. LC n-3 PUFAs modulate some of these processes, especially in normal weight individuals which may be preventative and limit the development of restrictive and inflammatory scWAT in the development of obesity. We conclude that a higher dose or longer duration of LC n-3 PUFA intervention may be needed to reduce obesity-associated scWAT inflammation and promote tissue homeostasis.
Clinical Trial Registration: www.isrctn.com, identifier ISRCTN96712688.
Introduction
Obesity is characterised by an increase in adipose tissue mass and is accompanied by a state of chronic low-grade inflammation in which physiological functions of adipose tissue and whole-body homeostasis are dysregulated (1). Consequently, obesity and the expansion of white adipose tissue (WAT) are strongly associated with comorbidities such as insulin resistance and type 2 diabetes (2–5). WAT expansion, reorganisation, and associated inflammation link the pathophysiology of obesity with metabolic complications. The expansion of pre-existing adipocytes (hypertrophy) and reorganisation of the tissue environment are accompanied by reduced expandability of restricted adipocytes, distancing them from the vasculature resulting in regions of hypoxia (6–9), immune cell infiltration, and inflammatory signalling (10–14).
The expansion of WAT is under tight regulation of transcription factors, gene expression, and many inflammatory signalling mediators such as cytokines, which have a role in lipogenesis and lipolysis, and therefore energy regulation, as well as in the remodelling of the surrounding microenvironment (3, 15). Adipogenesis and WAT expansion are nutritionally regulated under the control of Wnt/β-catenin signalling (16). Wnt and dishevelled binding antagonist of beta catenin (DACT, alias DAPPER) ligand expression are differentially regulated in response to nutrient surplus, consequently upregulating the expression of adipogenic factors to accommodate the need for enhanced triglyceride (TG) storage (16). During WAT expansion, there is downregulation of Wnt expression, often observed in conjunction with upregulated expression of DACT genes (16, 17); however, regulation of this pathway in WAT in human obesity is not described (18). Furthermore, in adipocytes, the canonical Wnt/β-catenin pathway has been shown to regulate de novo lipogenesis and fatty acid monounsaturation (19).
As WAT expands and regions of the tissue become hypoxic, inflammatory signalling promotes the reorganisation of the tissue environment to reconnect with the vasculature. The presence and role of hypoxia in human obesity is not well described as reviewed by Ruiz-Odeja (20) but stabilisation of hypoxia inducible factor-1α (HIF-1α) in hypoxia stimulates the expression of a range of genes involved in angiogenesis, glycolysis, and erythropoiesis (7, 11, 20–23). Hypoxia promotes immune cell recruitment and elicits a fibrotic response by inducing the expression of many extracellular matrix (ECM) components such as collagens (11, 24). Examination of the WAT transcriptome has revealed dysregulated expression of many ECM components in obesity (25, 26). However, evaluation of changes to WAT morphology in human obesity is limited and inconsistent as reviewed by DeBari and Abbott (27).
Eicosapentaenoic acid (EPA) and docosahexaenoic acid (DHA) are the two most bioactive long chain omega-3 polyunsaturated fatty acids (LC n-3 PUFAs) and have been widely investigated for their anti-inflammatory actions. In addition to potentially influencing inflammatory mediator signalling, EPA and DHA elicit their actions via altering the activity of transcription factors to modulate inflammation and other processes in WAT (28, 29). We previously reported the modulation of the scWAT transcriptome in human obesity by EPA and DHA resulting in downregulation of genes involved in immune and inflammatory responses (30). Furthermore, EPA and DHA have been reported to regulate the expression of genes associated with lipid accumulation in WAT (31, 32), decrease hepatic fibrosis accompanying metabolic complication through decreasing expression of collagen-associated genes and the presence of collagen fibres (33, 34), and impair collagen reorganisation in wound healing in mice (35). Therefore, EPA and DHA may have the potential to modulate scWAT expansion and remodelling; however, the effects of LC n-3 PUFAs on these outcomes in scWAT in human obesity are not described.
In this study, we combine whole tissue transcriptome profiling, investigation of tissue morphology and macrophage infiltration, and inflammatory marker analysis to describe obesity-associated expansion and remodelling of scWAT, responses to chronic LC n-3 PUFA intervention, and possible mechanisms by which these occur.
Material and Methods
50 healthy normal weight (BMI 18.5 to 25 kg/m2) and 50 healthy obese (BMI 30 to 40 kg/m2, waist circumference ≥ 94 cm males and ≥ 80 cm females) individuals aged 18-65 years who were able to provide written informed consent were recruited into a double-blind placebo (comparator oil) controlled trial. Exclusion criteria included: being outside the defined age or BMI and waist circumference categories, having diagnosed metabolic disease (e.g. diabetes, cardiovascular disease) or chronic gastrointestinal problems (e.g. inflammatory bowel disease, celiac disease, and cancer), the use of prescribed medicine to control inflammation, blood lipids or blood pressure, consumption of more than one portion of oily fish per week (140 g cooked), use of fish oil or other oil supplements, being pregnant or planning to become pregnant during the study period, and participation in another clinical trial.
Study Design
Fasted blood and an abdominal scWAT biopsy (~1 g) were collected at baseline (week-0) and following 12-week intervention (week-12) during which participants were randomised to consume either 3 g of a concentrated fish oil (EPAX6000; EPAX, Alesund, Norway providing 1.1 g EPA + 0.8 g DHA) or 3 g of corn oil (providing 1.65 g linoleic acid and 0.81 g oleic acid) per day (Figure 1). Blinding, randomization, and supplement packaging were completed by the Research Pharmacy at Southampton General Hospital, Southampton, United Kingdom, by individuals independent of the researchers involved in the study. Treatment group blinding was maintained until completion of statistical analysis of all data.
Sample Preparation
Abdominal scWAT biopsies were collected by surgical removal under local anaesthetic (1% lidocaine) to provide ~1 g of intact tissue which was directly stored on ice. Tissue was divided into 5 x ~200 mg aliquots. scWAT designated for FA and lipid metabolite analyses was wrapped in foil, placed in cryovials and snap frozen in liquid nitrogen. scWAT designated for RNA analysis was stored in 4 mL of RNAlater (Sigma, St. Louis, Missouri, United States) and stored for 24 hours between 2-4°C and then at -20°C for longer storage.
Blood Analyses
Plasma was prepared from ~5 mL of heparinised blood as previously described (30). Plasma TG, cholesterol, high density lipoprotein cholesterol (HDL-C), nonesterified fatty acid (NEFA), and glucose concentrations were measured using an iLAB 600 clinical chemistry analyzer and software (Instrumentation Laboratories, Bedford, Massachusetts) and enzyme-based kits (Wako, Osaka, Japan). Low density lipoprotein cholesterol (LDL-C) concentrations were estimated by using the Friedwald equation. Plasma insulin concentrations were measured by ELISA (Dako, Agilent, Santa Clara, California) and homeostasis model assessment 2 of insulin resistance (HOMA2-IR) was calculated as follows as previously described (30). Concentrations of interleukin (IL)-6 and adiponectin were determined from fasted plasma using luminex performance assays as previously described (30).
Anthropometry
Height was measured using a Seca stadiometer (Seca, Hamburg, Germany), waist and hip circumference measurements were made using a tape measure, and weight and body composition measurements were made using digital impedance apparatus (TANITA BC-418) as previously described (36).
Fatty Acid Composition
Lipids were extracted from frozen scWAT and fatty acid methyl esters (FAMEs) formed as previously described (36). FAMEs were separated by gas chromatography on a BPX-70 fused silica capillary column (30 m x 0.2 mm x 0.25 µm; manufactured by SGE) in a HP6890 gas chromatograph fitted with a flame ionisation detector. Instrument run conditions were as described elsewhere (36).
Gene Expression
RNA was isolated from ~150 mg scWAT stored in RNAlater® using the RNeasy lipid tissue mini kit™ (QIAGEN, Hilden, Germany) as previously described (30). Sequencing was performed on a Hiseq2000 platform with 5 samples per lane in a total of 8 lanes (SE50) with a total of 20 million reads. RNA-Seq reads were aligned to the hg38.0 reference genome using TopHat (open source, Johns Hopkins University, Center for Computational Biology, Baltimore, United States) and a read count table produced using HTSeq (open source, Huber group, Heidelberg, Germany). The read counts were filtered, and normalised CPM were used to evaluate gene expression as previously described (30). The results from this subset were validated via qRT-PCR of RNA extracted from the whole cohort as previously described (30), and showed the subset to be representative of the whole cohort. Gene ontology functional annotation was performed using the Database for Annotation, Visualization and Integrated Discovery (DAVID) (37, 38). Gene set enrichment analysis was performed using GSEA (39, 40). Ingenuity pathway analysis (Qiagen, Hilden, Germany) was run to identify pathways and networks enriched amongst the differentially expressed genes.
Histochemical Analyses
scWAT was fixed in 4 mL of 10% neutral buffered formalin and stored at room temperature and then embedded in paraffin wax. 4 µM sections were cut using a Leica RM2125 RTS microtome (Leica Biosystems, Wetzlar, Germany) and dried onto glass slides at 37°C overnight. A sub-set of 20 paired pre- and post-intervention samples (10 normal weight, 10 obese, including the 20 samples analysed for RNA-Sequencing) were selected for H&E staining, Picro Sirius red staining, and CD68 staining.
Statistical Analysis
Sample size was calculated considering the typical distribution and expected response of circulating cytokines, not reported here (a 20% decrease following LC n-3 PUFA intervention) and participant drop out of 20%. A sample size of 25 participants per group (BMI and treatment subgroup) was determined to be able to detect changes in circulating cytokines at > 80% power and a 5% level of significance with consideration for 20% loss. No formal power calculation was performed specifically for the outcomes described herein. Not all data were normally distributed, and non-normal data could not be corrected with log10 transformation. Therefore, appropriate non-parametric tests were performed, and all data are displayed as median and interquartile range (IQR). 170 genes that were differentially expressed in individuals living with obesity by at least a 2 FC were selected for analysis using IPA.
Results
Participant Characteristics
Individuals living with obesity had significantly greater BMI, waist circumference, hip circumference, % body fat, body fat mass (kg), and higher blood concentrations of TG, total cholesterol, LDL-C, glucose, and insulin, in comparison to normal weight individuals (Table 1). Individuals living with obesity also had higher concentrations of IL-6 and leptin in comparison to normal weight individuals allocated to the corn oil intervention group. Individuals living with obesity also had lower adiponectin concentrations than normal weight individuals allocated to the corn oil intervention group (Table 1). Individuals living with obesity had a significantly higher average HOMA2-IR score; however, this was still within the ‘normal range’ (HOMA-IR < 1.95). As no individuals with diagnosed metabolic or inflammatory complications were recruited, and obese individuals did not exhibit clinical hypertriglyceridemia and had HOMA2-IR scores within the normal range, the obese cohort were defined as currently living with obesity in which metabolic syndrome is yet to manifest.
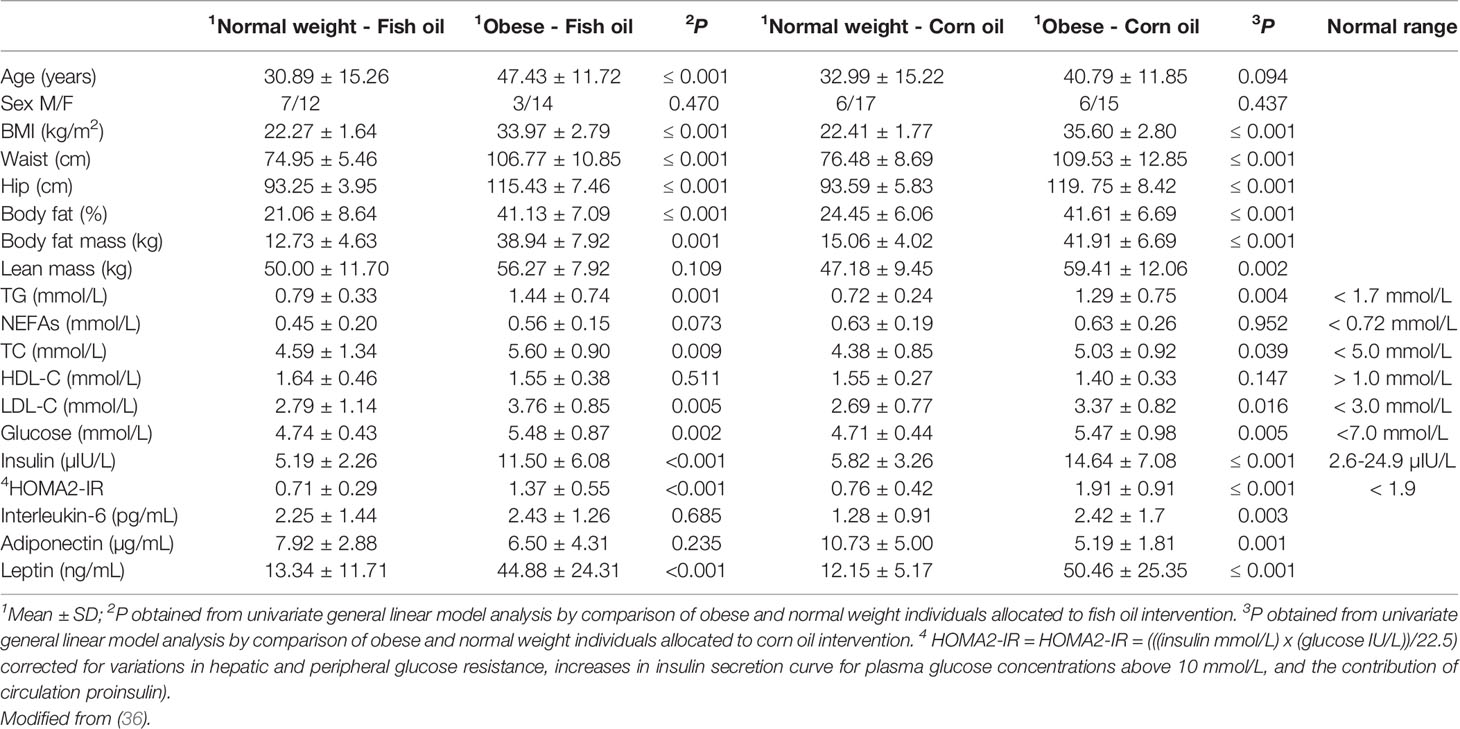
Table 1 Anthropometric and metabolic characteristics in normal weight and individuals living with obesity.
Obesity Is Associated With a Specific scWAT Transcriptome Suggestive of Enhanced Expansion and Remodelling in Response to Inflammation
Sequencing of RNA extracted from human scWAT identified 789 genes differentially expressed by at least a two-fold change in individuals living with obesity in comparison to normal weight individuals (P and FDR ≤0.05). These 789 genes were further examined, and GO identified 170 genes that were enriched in tissue structure, remodelling, and expansion processes. The top enriched process was ECM organisation; other enriched processes included collagen organisation, angiogenesis and blood vessel remodelling, cell proliferation, and response to hypoxia (P < 0.001 Table 2). Top upregulated genes include epidermal growth factor like protein-6 (EGFL6), MMPs, collagens, integrins, and other genes associated with ECM structure. Top downregulated genes included other collagens and alpha-2 glycoprotein-1 (AZGP1) (P ≤ 0.003, Table 3).
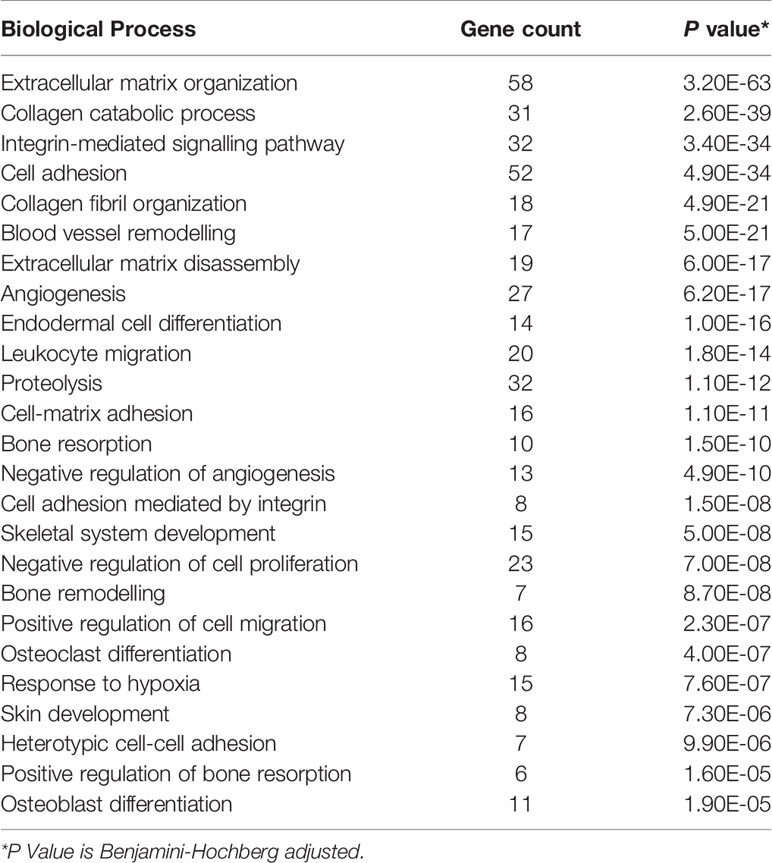
Table 2 Top 25 enriched biological processes, determined by GO, in scWAT in individuals living with obesity.
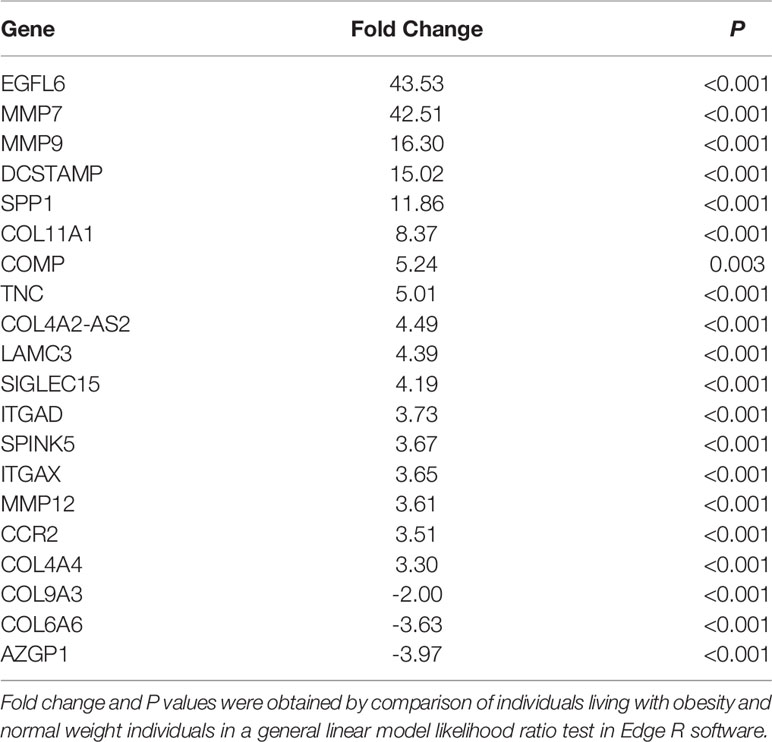
Table 3 The top differentially expressed genes associated with tissue structure and remodelling in scWAT in individuals living with obesity at study entry (week 0).
Ingenuity pathway analysis (IPA) (Qiagen, Hilden, Germany), which considers the differential expression, significance, counts per million (CPM), and false discovery rate (FDR) of the gene data, identified several canonical pathways to be upregulated in scWAT from individuals living with obesity. These processes fell into two major themes, upregulation of inflammatory and immune response processes and upregulation of tissue remodelling. We previously reported upregulation of the immune and inflammatory response in these individuals living with obesity when assessing the full gene set (30). In the current analysis, there is common overlap between inflammatory signalling and tissue expansion and remodelling. These enriched canonical pathways include upregulation of cytokine signalling, immune cell signalling and differentiation, and activation of inflammatory pathways such as the inflammasome pathway (P ≤ 0.05, Figure 2A). The enriched pathways involved in tissue remodelling include upregulation of hepatic fibrosis signalling, HIF-1α and vascular endothelial growth factor (VEGF) signalling, actin cytoskeleton signalling and dendritic cell maturation, Wnt/β-catenin signalling, and downregulation of inhibition of MMPs (P ≤ 0.05, Figure 2B).
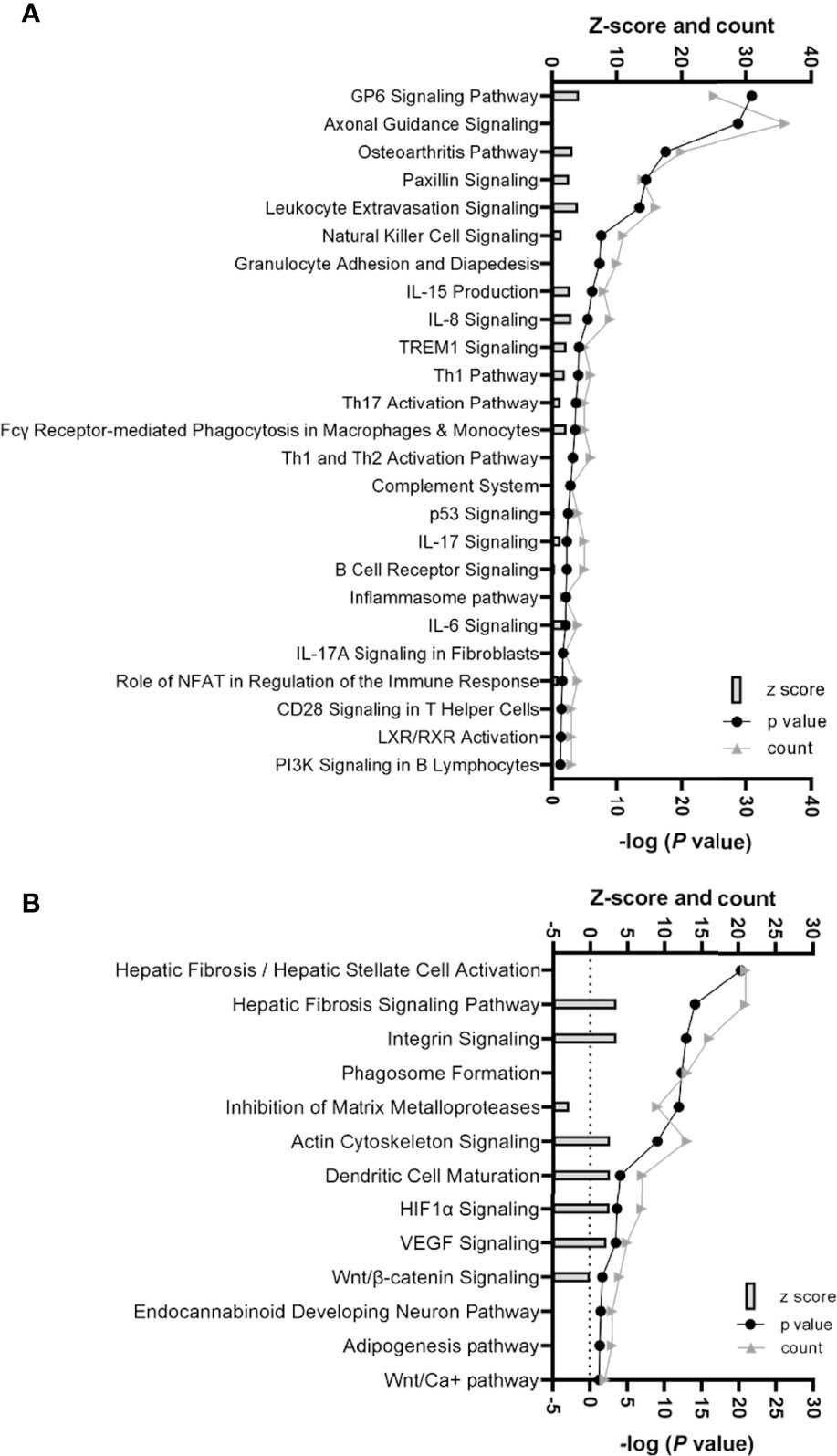
Figure 2 (A) Histogram of 25 significantly enriched canonical pathways involved in inflammation and immune response in scWAT in individuals living with obesity. (B) Histogram of significantly enriched canonical pathways involved in tissue remodelling and expansion in scWAT in individuals living with obesity. (A, B) The left y-axis represents the z-score (predicted score of activation/inhibition) and the number of genes counted for each of the representative canonical pathways, and the right y-axis represents -log (P-value), significance is deemed -log P> 1.3. Where z-score is not present, there was not enough evidence from current literature to determine if the altered gene expression is likely to result in activation or inhibition of a canonical pathway.
Selected genes involved in several of the above pathways are detailed in Table 3. Higher expression of angiopoietin-2 (ANGPT2), HIF-1α, EGF like domain multiple 6 (EGFL6), several MMP genes, and growth/differentiation factor-15 (GDF15) was observed in individuals living with obesity (P ≤ 0.008, Table 4). There was significant enrichment of the Wnt/β-catenin signalling pathway; there was an overall positive enrichment of genes in this pathway but several of these are negative regulators of Wnt signalling (Figure 3). In addition, there was negative enrichment of several key Wnt signalling genes (Figure 3). This was concordant with individual gene expression and overall downregulation of Wnt/β-catenin signalling pathway (Figure 4). Several genes associated with the Wnt/β-catenin signalling pathway involved in adipogenesis and lipogenesis, were differentially regulated in individuals living with obesity (Figure 2B). Of note, there was lower expression of DACT-2, Wnt family member-3a (WNT3A), Wnt family member-5a (WNT5A), Wnt family member-10B (WNT10B) (P ≤ 0.033, Table 4 and Figure 4), negative regulators of Wnt signalling, secreted frizzled related proteins SFRP2 and SFRP4 (P ≤ 0.001, Figure 4), and greater expression of Wnt receptors, frizzleds FZD1, FZD2, FZD5 and FZD8 (P = ≤ 0.048, Figure 4), co-receptors required for Wnt signalling, lipoprotein receptor-related proteins (LRPs) LRP1, LRP5 and LRP6 (P ≤ 0.032, Figure 4), and greater expression of T cell factor/lymphoid enhancer factors TCF7L1 and TCF7L2 (P ≤ 0.049, Figure 4) which repress target gene expression when Wnt signals are absent.
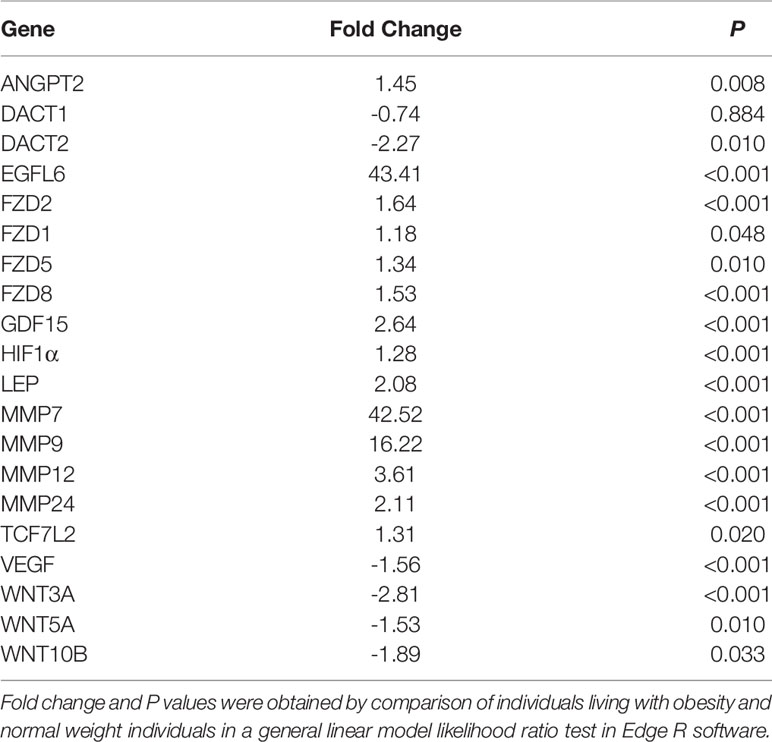
Table 4 Fold change of genes involved in expansion (hypertrophy and hyperplasia) and remodelling in scWAT in individuals living with obesity at study entry (week 0).
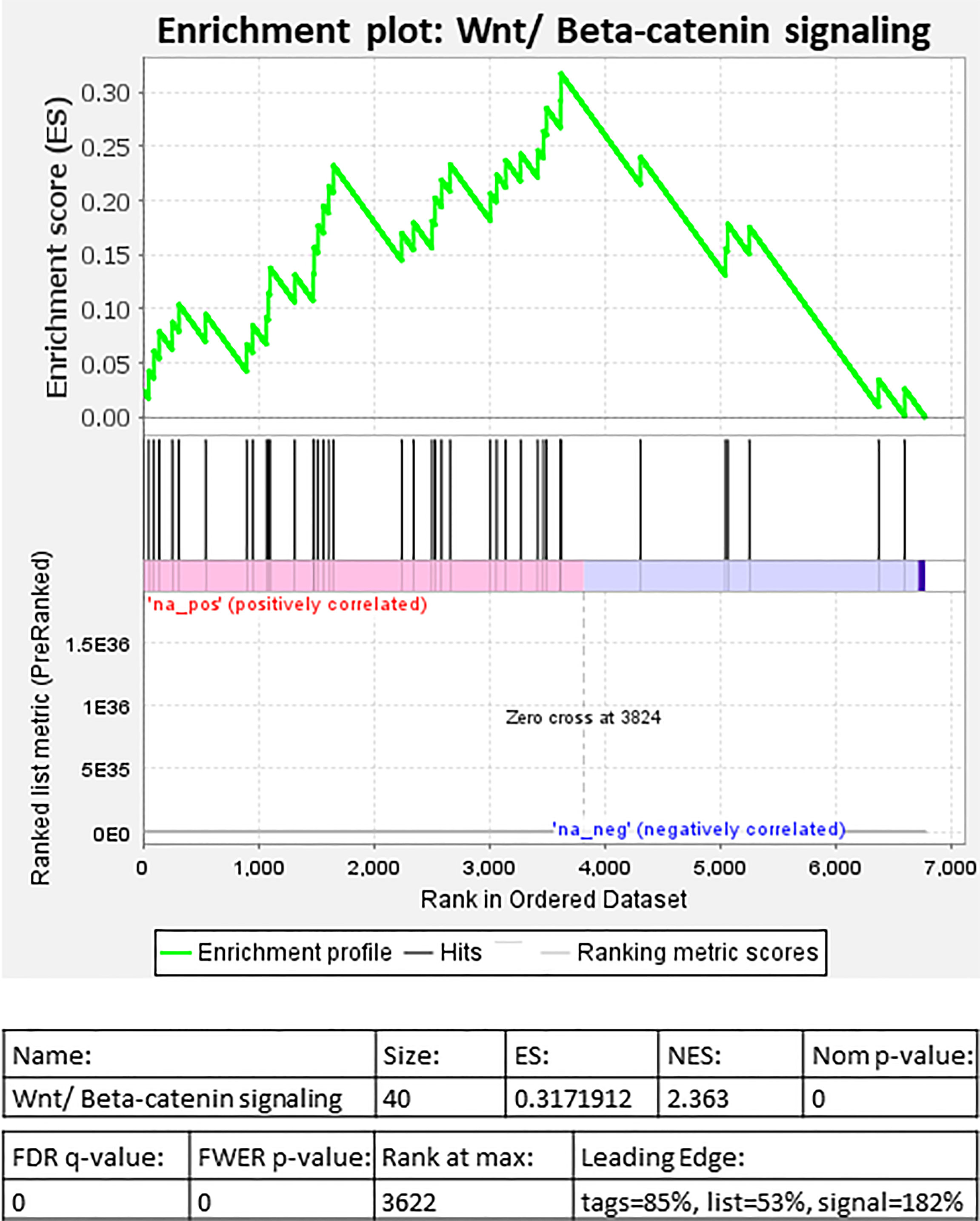
Figure 3 Gene set enrichment analysis (GSEA) of differentially expressed genes in individuals living with obesity at week-0: Enrichment plot for Wnt/β-Catenin signalling.
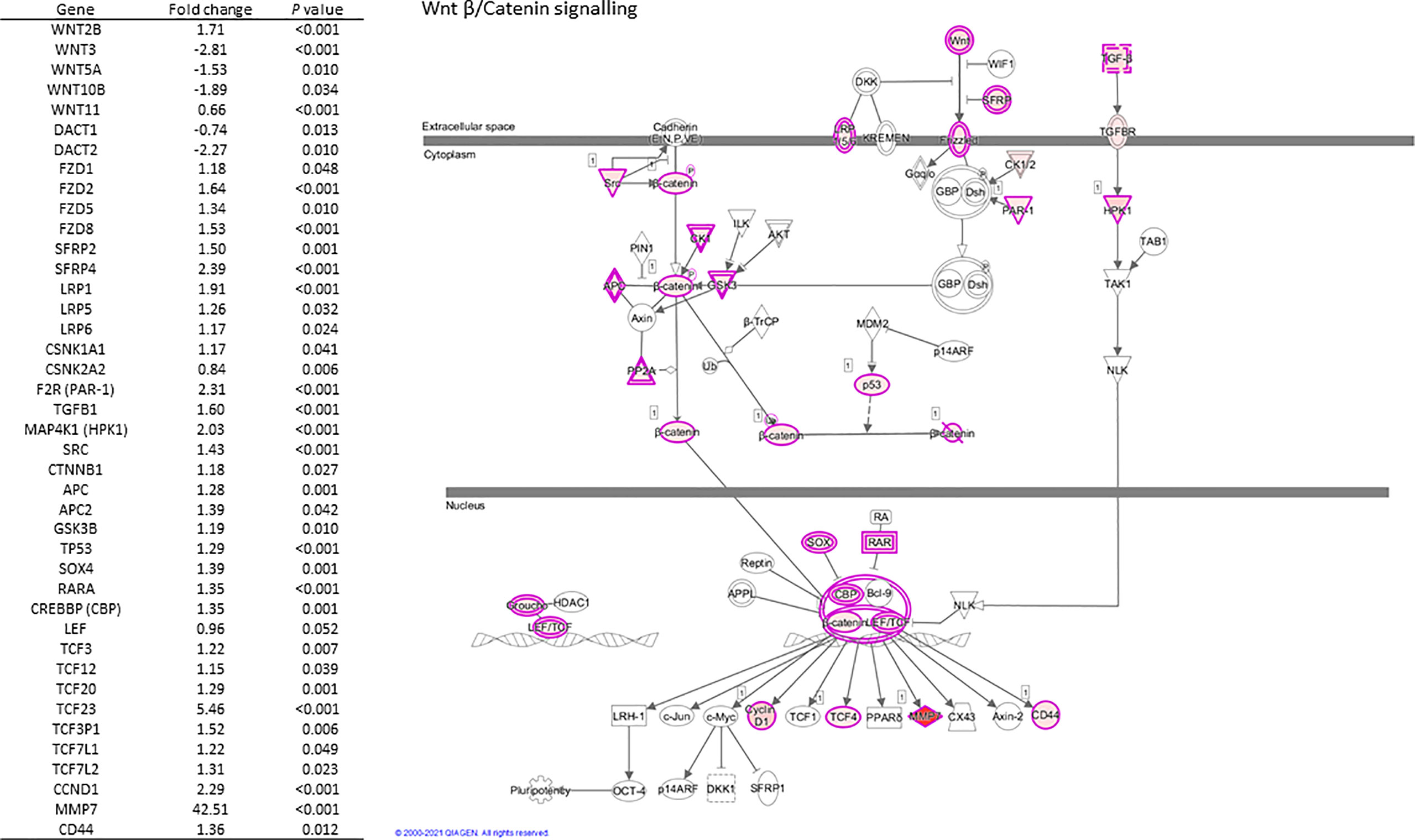
Figure 4 Regulation of scWATWnt/β-Catenin signalling and differential expression of pathway genes in individuals living with obesity at week-0.
These data suggest obesity in which metabolic complications are yet to manifest is associated with a specific adipose transcriptome profile indicative of enhanced tissue remodelling and expansion in response to obesity-associated tissue inflammation and upregulation of immune response processes. In addition, we identify the dysregulation of the scWAT Wnt signalling pathway in obesity.
Obesity Is Associated With scWAT Hypertrophy and Accumulation of Macrophages but Not Fibrosis
Histochemical staining of scWAT revealed individuals living with obesity exhibit tissue hypertrophy in which the average adipocyte size was larger, in addition to a greater number of large, very large, and extra-large adipocytes in comparison to normal weight individuals (P ≤ 0.050, Figures 5, 6). There was a greater number of macrophages accumulating in crown like structures (CLS) (P = 0.023) (defined as 3 or more macrophages aggregating around a single adipocyte) and a trend for higher numbers of macrophages in general (P = 0.063) in the scWAT of individuals living with obesity in comparison to normal weight individuals (Figure 7). The higher number of CLS present in scWAT of individuals living with obesity may reflect a higher proportion of pro-inflammatory M1 macrophages. The number of CLS per 100 cm2 of scWAT was positively correlated with BMI and body fat (kg) (ρ = 0.192, P = 0.023 and ρ = 0.219, P = 0.029 respectively) and number of macrophages per 100 cm2 of scWAT was positively correlated with body fat (kg) (ρ = 0.362, P = 0.041). However, despite being significant, these correlations are not particularly strong and do not indicate BMI or body fat (kg) to be a clear predictor of macrophage or CLS numbers. There were no significant correlations with insulin or HOMA2-IR (data not shown). There is variation in CLS number in obesity that is not explained by variation in blood lipid or metabolic parameters but may be due to variation in adipokines and cytokines. The number of CLS per 100 cm2 of scWAT was positively correlated with circulating IL-6 (ρ= 0.491, P = 0.028) and negatively correlated with circulating adiponectin concentrations (ρ = -0.477, P = 0.028). In addition, adipocyte size was positively correlated with IL-6 (ρ = 0.499, P = 0.025) and both adipocyte size and pericellular fibrosis were negatively correlated with adiponectin (ρ = -0.597, P = 0.005, and ρ = -0.617, P = 0.004, respectively). Pericellular fibrosis was also positively correlated with HOMA2-IR (ρ = 0.493, P = 0.027) but the level of this fibrosis was not altered in obesity suggesting this may be dependent on insulin sensitivity and may be more prevalent in the later stages of obesity coinciding with manifestation of metabolic syndrome.
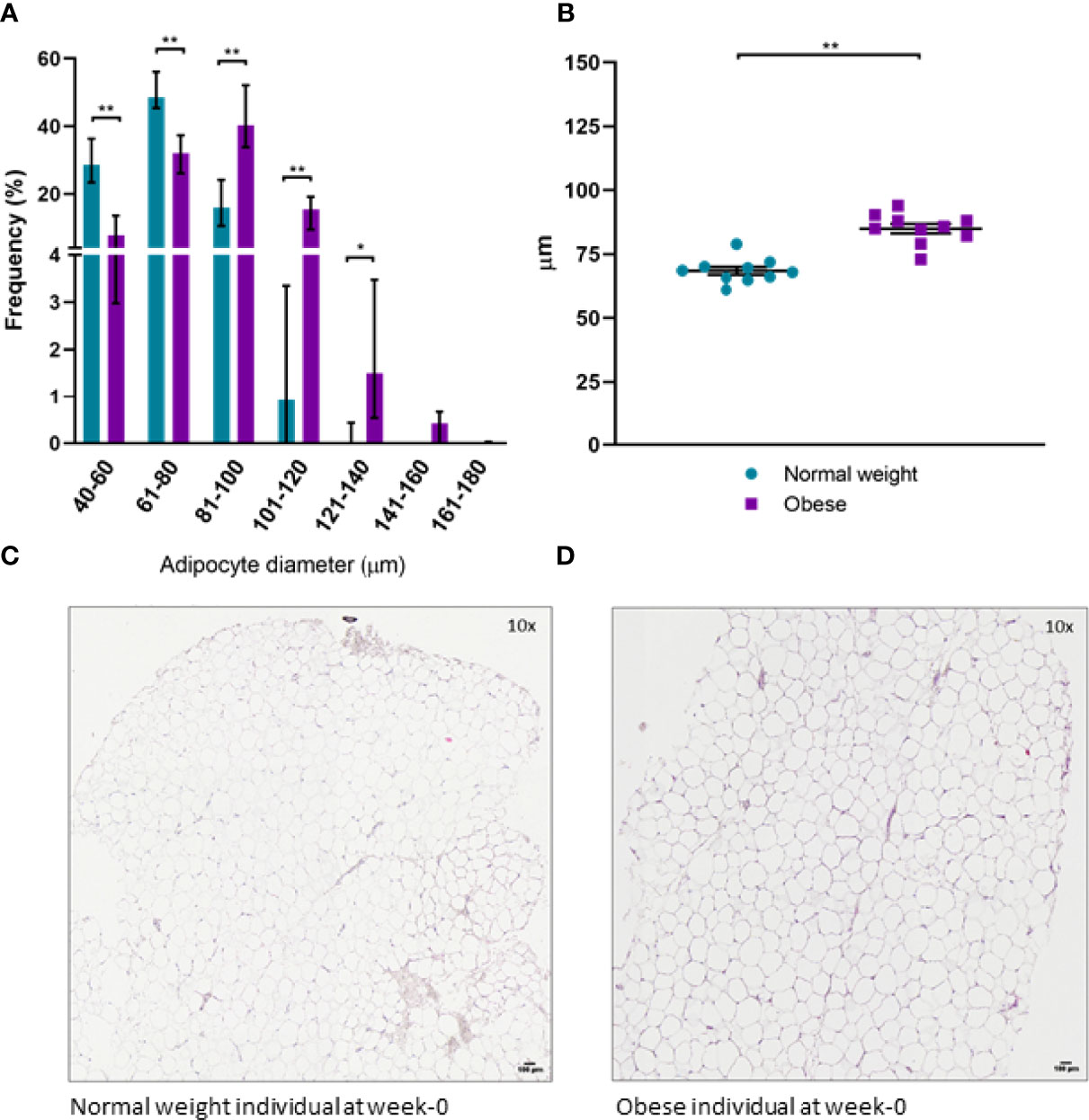
Figure 5 (A) Distribution of adipocyte size in scWAT in normal weight and individuals living with obesity at week-0; (B) Average adipocyte diameter in normal weight and individuals living with obesity at week-0; (C) H&E stained section of scWAT at 10 x magnification from a normal weight individual at week-0; (D) H&E stained section of scWAT at 10 x magnification from an individual living with obesity at week-0. **P < 0.001, *P < 0.050.
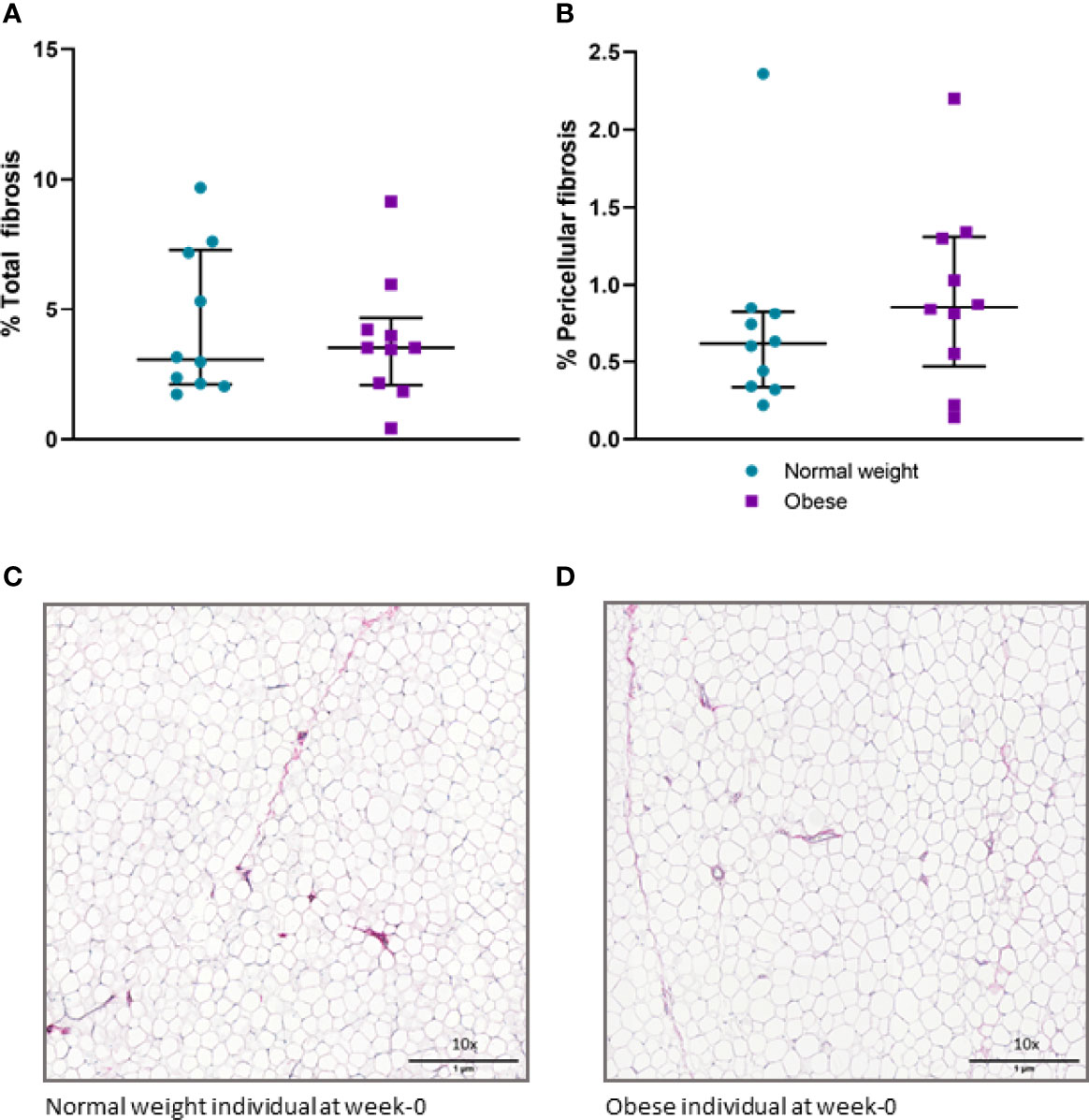
Figure 6 (A) Percentage of total fibrosis in scWAT in normal weight and individuals living with obesity at week-0; (B) Percentage of pericellular fibrosis in scWAT in normal weight and individuals living with obesity at week-0; (C) Picro Sirius red stained collagen in a section of scWAT at 10 x magnification from a normal weight individual at week-0; (D) Picro Sirius red stained collagen in a section of scWAT at 10 x magnification from an individual living with obesity at week-0.
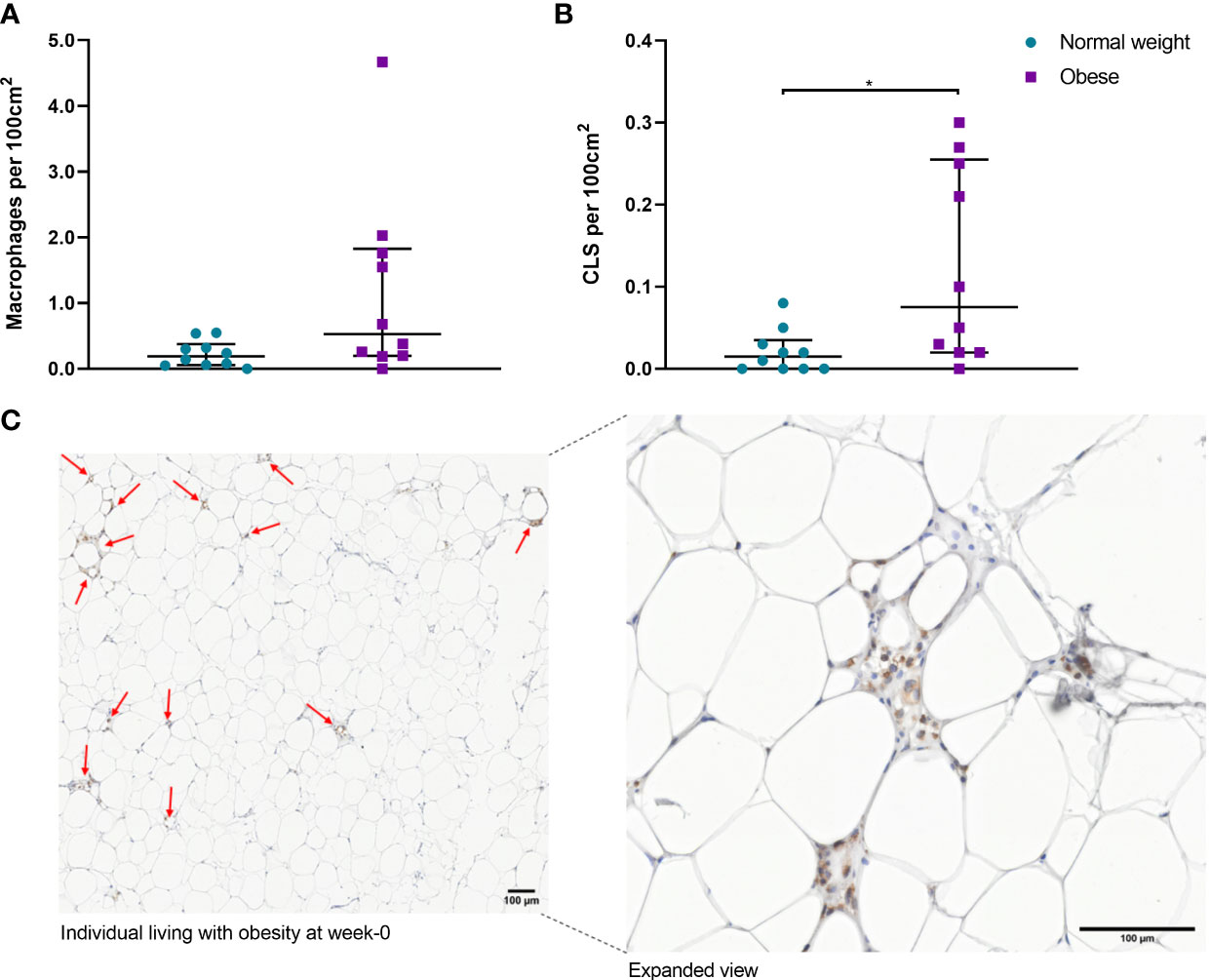
Figure 7 (A) Number of macrophages per 100cm2 of scWAT from normal weight and individuals living with obesity at week-0; (B) Number of CLS per 100 cm2 of scWAT from normal weight and individuals living with obesity at week-0; (C) CD68 stained macrophages in a section of scWAT at 20 x magnification from an individual living with obesity at week-0. *P = 0.023
A summary of the regulation of scWAT in obesity is depicted in Figure 8.
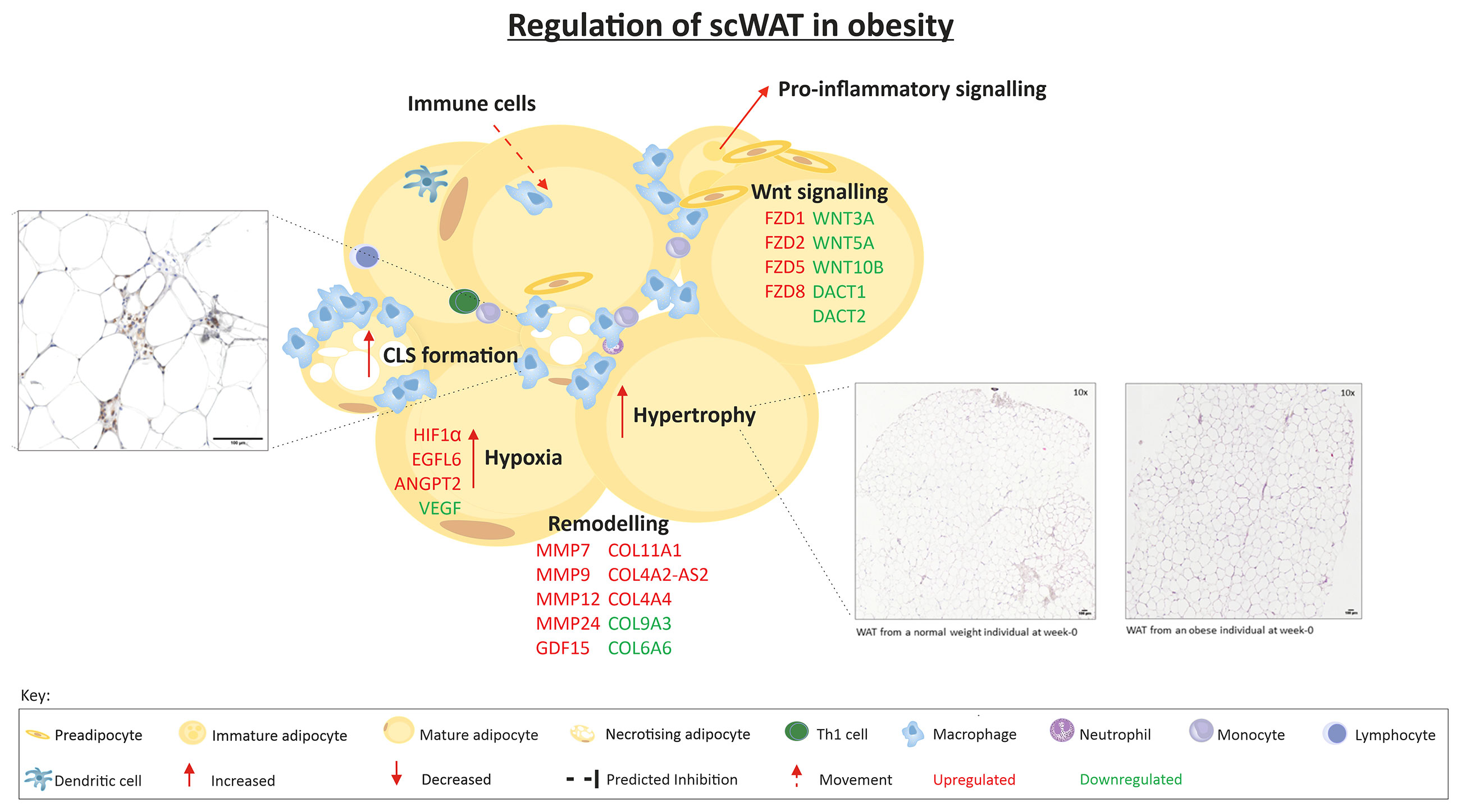
Figure 8 The regulation of scWAT in obesity in which metabolic complications are yet to manifest. Indication of upregulation or downregulation of mRNA expression is the expression in individuals living with obesity in comparison to that of normal weight individuals at week-0.
Chronic Supplementation With LC n-3 PUFA Modulates Expression of scWAT Genes but Does Not Alter Tissue Morphology
We previously reported that 12-weeks of LC n-3 PUFAs increased scWAT proportions of EPA, DPA, and DHA to a similar extent in both BMI groups but this was only significant in normal weight individuals (36). EPA, DPA and DHA also increased in erythrocytes following 12-week LC n-3 PUFA intervention in both normal weight individuals and those living with obesity (Table 5). 12-week EPA+DHA significantly modulated the expression of several genes involved in tissue remodelling and expansion processes (Table 6). These genes are associated with the upregulation of blood vessel remodelling, actin filament binding, cell differentiation, and apoptotic cell clearance in normal weight individuals, and with anatomical structure morphogenesis and the negative regulation of cell proliferation in individuals living with obesity. In addition, LC n-3 PUFAs downregulated genes associated with angiogenesis, inflammatory response and circadian rhythm in normal weight individuals, and downregulated genes associated with cell differentiation, negative regulation of cell adhesion, and Wnt signalling in individuals living with obesity. A summary of the effects of LC n-3 PUFAs on the regulation of scWAT in normal weight individuals and individuals living with obesity in which metabolic complications are yet to manifest is depicted in Figure 9.
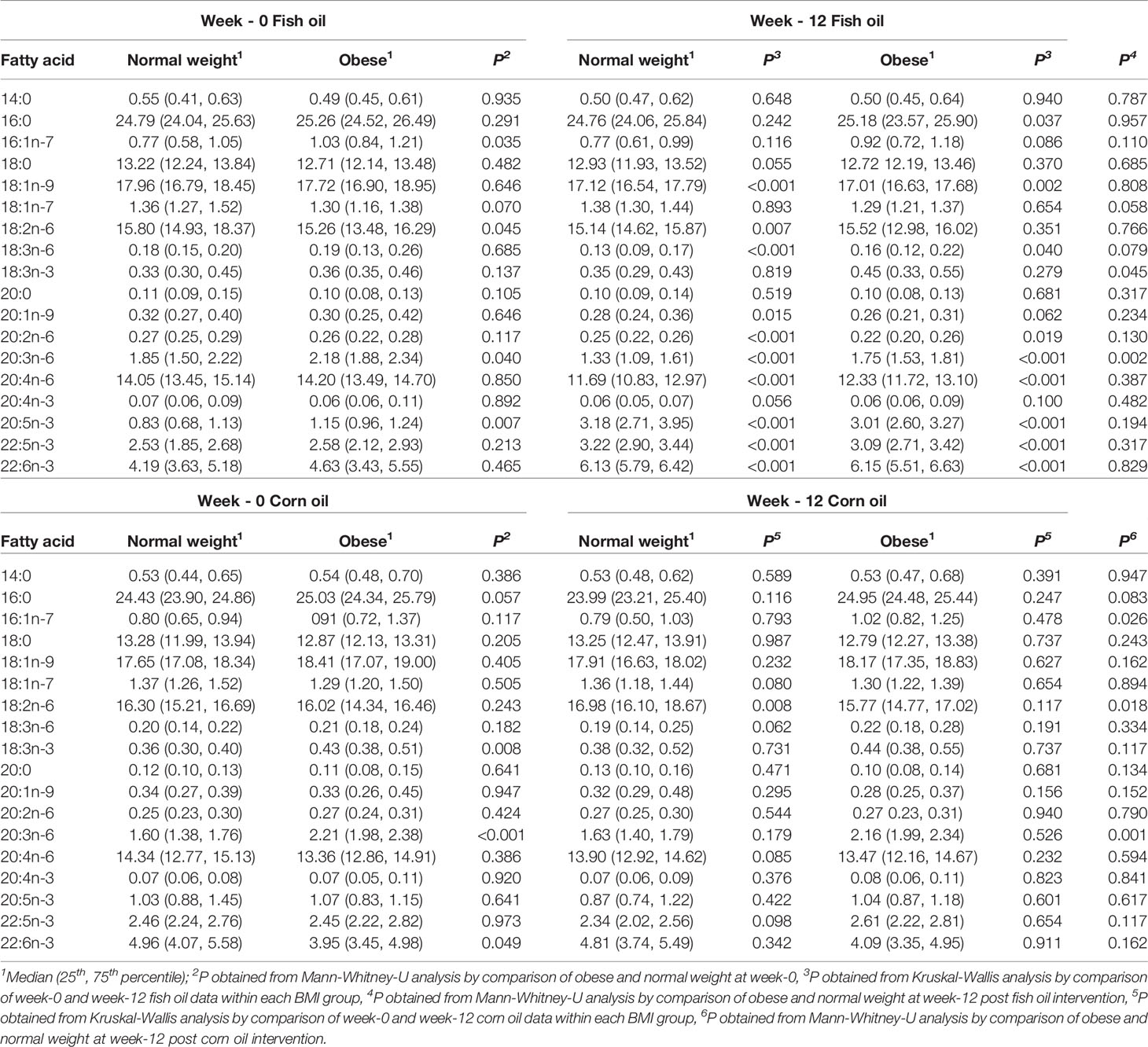
Table 5 Erythrocyte fatty acids at study entry and following 12-week intervention with LC n-3 PUFAs or corn oil in normal weight and individuals living with obesity.
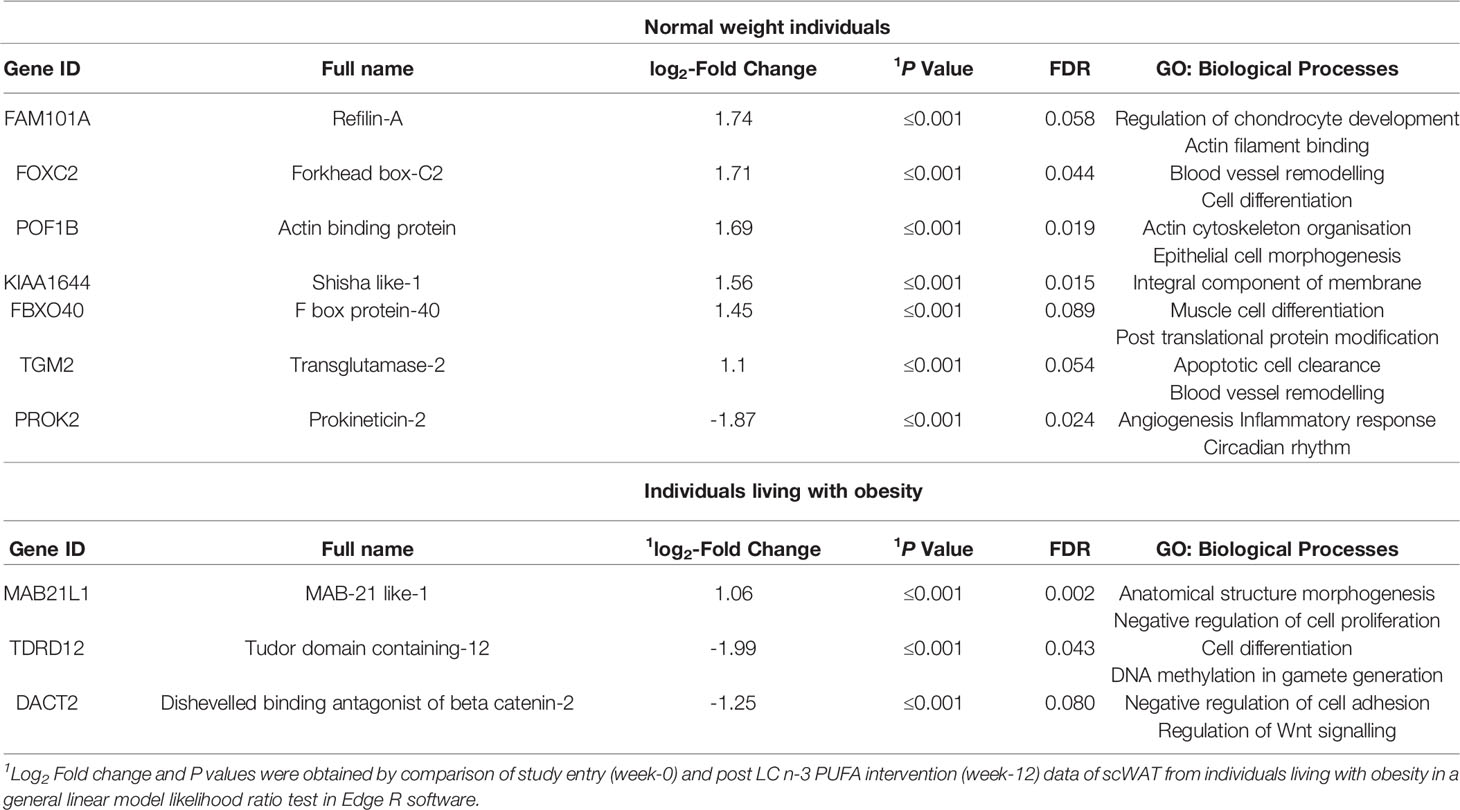
Table 6 Remodelling associated genes significantly modulated by 12-weeks intervention with LC n-3 PUFAs in normal weight and individuals living with obesity.
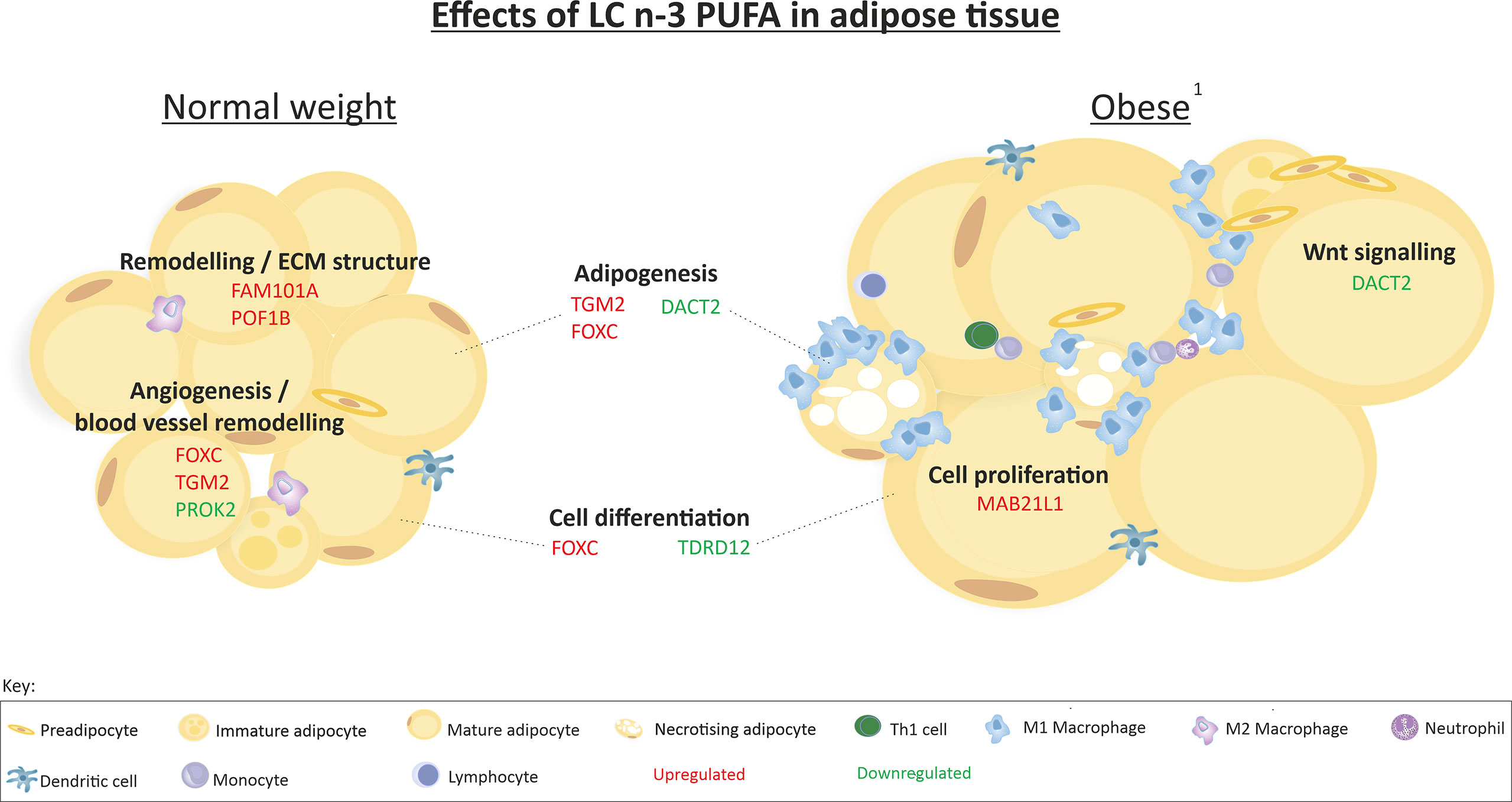
Figure 9 Summary of the effects of 12-week LC n-3 PUFA intervention on scWAT in normal weight individuals and individuals living with obesity. 1 Obesity in which metabolic complications are yet to manifest. Indication of upregulation or downregulation of mRNA expression is the expression at week-12 in comparison to week-0 in each group of individuals.
Discussion
Dysregulation of Adipogenesis and Tissue Expansion in Obesity - The Role of Wnt Signalling
We report altered expression of several genes involved in scWAT adipogenesis and tissue expansion suggesting an upregulation of these processes in the early stages of obesity. We confirmed obesity-associated hypertrophy in which we observed significantly enlarged adipocytes in individuals living with obesity even before significant metabolic perturbations are obvious.
A group of proteins that have a role in controlling adipose growth and expansion in response to nutritional cues are encoded by the Wnt genes. Wnt signalling proteins bind to cell surface receptors composed of frizzleds and LRPs -5 and -6. This binding activates DACTs resulting in the inhibition of glycogen synthease 3-β kinase (GSK3) and of β-catenin phosphorylation (Figure 4) (41). Non-phosphorylated β-catenin translocates to the nucleus to regulate target gene expression. Wnt signalling proteins are anti-adipogenic and are inhibited by DACT proteins, so when there is high expression of DACTs, Wnt signalling is inhibited and adipogenesis occurs. Therefore, during WAT expansion, the expression of Wnts including WNT3A and WNT10B is decreased, often seen in conjunction with increased expression of DACT genes (16, 17). Our data support this and provide evidence for dysregulated mRNA expression of several key Wnt signalling components in human scWAT.
We observe downregulation of WNT3A, WNT5A, and WNT10B which is consistent with observation of enhanced TCF/LEF gene expression which represses target gene expression when Wnt signals are absent (42). Wnt effectors such as TCF7L2 may be potential targets for therapeutic treatment of Wnt-related metabolic disease (43). TCF7L2 mRNA expression is reported to be the strongest type-2 diabetes candidate gene and inactivation of TCF7L2 leads to hepatic insulin resistance and decreased whole body glucose tolerance (43). In the current study, conversely, TCF7L2 mRNA expression was negatively correlated with HOMA2-IR scores (ρ = -0.526, P = 0.017, data not shown). Non-canonical signalling of WNT5A is reported to contribute to obesity-induced inflammation and systemic insulin resistance in obese mice independent of tissue expansion (44). In contrast to previous reports of WNT5A positively correlating with IL-6 and insulin resistance (44, 45), data from the current study in which metabolic complications are yet to manifest, identifies negative correlations between WNT5A mRNA expression and HOMA2-IR (ρ = -0.480, P = 0.032) and plasma IL-6 concentrations (ρ = -0.445, P = 0.049). These data suggest that there is decreased WNT5A expression with declining metabolic health and increasing systemic inflammation. This is not consistent with previous reports but may reflect hijacking of the Wnt system by inflammatory cytokines such as IL-6 (46). Individuals living with obesity in the current study exhibited higher circulating concentrations of IL-6 and higher HOMA2-IR scores in conjunction with downregulation of scWAT WNT5A mRNA expression.
We also observed downregulated DACT2 mRNA expression, which is conflicting with the inhibition of Wnt gene expression. DACT1 has a well-defined role in inhibiting Wnt gene expression but the action of DACT2 in human scWAT has yet to be defined (47). It may be that DACT2 is involved in non-canonical Wnt signalling in human scWAT, or that there is dysregulation of this system in obesity which is consistent with reports that DACT expression increases only to the point where the expansion limit of the scWAT is reached (16). Obesity beyond this point results in loss of adipose function and in this scenario, loss of DACT expression in conjunction with loss of Wnt signalling is observed (16). In addition to adipogenesis, it has been reported that activation of Wnt/β-catenin signalling strongly inhibits the expression of collagen genes and stimulates the expression of matrix protease genes resulting in loss of matrix in chondrocytes and cartilage (48) so this signalling pathway may have a role in ECM regulation in obesity.
Upregulation of Tissue Remodelling in Obesity – The Role of Hypoxia
We report altered expression of several genes involved in tissue remodelling as well as hypoxia and angiogenesis pathways in scWAT from individuals living with obesity. Data from the current study advances on evidence from a small cohort (n=25) of monozygotic BMI-discordant twins from the Finn-Twin study which exhibit differential expression of scWAT genes indicating upregulation of ECM remodelling associated genes including collagens, ECM glycoproteins, and proteogylcans (49–51). Data from the current study in a larger cohort of non-related adults with obesity, supports this limited evidence of upregulated collagen gene expression prior to metabolic complication but provides additional data describing lack of alteration to collagen deposition in the tissue itself despite altered regulation of a range of collagen genes.
We report dysregulation of collagen expression in individuals living with obesity observing both up- and down-regulated expression of individual collagens but we did not observe enhanced scWAT fibrosis in obesity. Collagen deposition in the tissue as measured by Picro Sirius red staining was positively correlated with insulin sensitivity, indicating association between tissue remodelling and insulin resistance, suggesting fibrosis may occur in the later stages of obesity accompanied by metabolic complications. The expression of GDF-15 mRNA was upregulated in individuals living with obesity; serum GDF-15 concentration has recently been reported as a predictor of liver fibrosis in patients with NAFLD and was involved in the association between insulin resistance and liver fibrosis (52). The expression of GDF-15 was not significantly associated with scWAT fibrosis in the current study but was positively associated with HOMA2-IR and leptin concentrations (P = 0.005, ρ = 0.599 and P = 0.007, ρ = 0.580 respectively), and negatively associated with adiponectin concentrations (P = 0.002, ρ = 0.657) suggesting association with metabolic health in scWAT.
In addition to fibrosis signalling, upregulated genes were associated with HIF-1α signalling, VEGF signalling, actin cytoskeleton, integrin and MMP signalling. Of note, MMP-7 and MMP-9 were highly upregulated by 42.5 and 16.3 fold in obesity, respectively. MMP-7 hydrolyses human plasminogen which results in angiogenic factors promoting blood vessel formation (53). It also releases TNF-α from the cell surface which has a role in inflammatory signalling but the role of MMP-7 in human obesity has not been defined (53). The current study is the first to report upregulation of MMP7 mRNA expression which contrasts with previous reports of decreased expression in murine obesity (54) and decreased circulating concentrations in human obesity (55). MMP-9 is secreted from pericytes, fibroblasts and macrophages and may be reflective of increased inflammatory macrophage presence in scWAT in obesity (56). These data suggest scWAT from individuals living with obesity exhibits signs of hypoxia and an attempt to reconnect the vasculature with upregulation of blood vessel formation and remodelling as well as ECM remodelling.
Enhanced scWAT Inflammatory Macrophage Infiltration in Obesity – Hypertrophy, Inflammation and Insulin Resistance
In obesity with accompanied insulin resistance, there is interaction between the fibrotic state of the scWAT and immune cell infiltration, thought to be due to the cascade of events occurring from adipocyte hypertrophy and consequential fibrosis. Given these interactions, it is suggested that scWAT infiltration of macrophages is correlated with fibrosis and insulin resistance (57); however, data from the current study do not support this. Neither total nor pericellular fibrosis was correlated with number of macrophages, number of CLS in the tissue, or HOMA2-IR. However, both number of macrophages and CLS were positively correlated with IL-6, and number of CLS was negatively correlated with adiponectin which plays a role in insulin sensitivity. This highlights the complexity of the relationship between fibrosis, immune cell recruitment, and insulin sensitivity.
Adipose dysfunction may manifest as a higher frequency of hypertrophic adipocytes in association with stress signals, necrosis, deposition of ECM components, and immune cell recruitment attributed to the pathological enlargement of adipocytes. Destabilisation of the ECM may lead to a reduction in mechanical stress on the expanding adipocytes and environment, and inflammation including macrophage infiltration only persists at the later stages of adipose dysfunction in response to an increasingly fibrotic ECM.
We report scWAT hypertrophy and increased CLS formation independent of tissue fibrosis which may suggest the balance between ECM breakdown and deposition is maintained in obesity in which metabolic complications have not yet manifested. However, the current study observes associations between number of CLS and pro-inflammatory cytokines, adiponectin and HOMA2-IR scores suggesting a more pro-inflammatory environment is associated with insulin signalling even in the absence of enhanced fibrosis and metabolic syndrome.
LC n-3 PUFA Modulation of scWAT Transcriptome: Adipogenesis, Morphology and Remodelling
LC n-3 PUFA intervention significantly modulated genes involved in adipogenesis (DACT2, TGM2 and FOXC2); however, the biological effects of this are uncertain as there may be an increase in adipogenesis via enhanced Wnt signalling due to the downregulation of its inhibitor DACT2 with LC n-3 PUFA intervention (16, 58–60). As discussed, the actions of DACT2 in adipose tissue are not defined; furthermore, the dysregulation of Wnt signalling at study entry in individuals living with obesity may interfere with physiological DACT2 signalling. These data pave the way to further understand this signalling pathway by identifying the alteration of DACT2 in human obesity and its modulation by dietary fatty acids. Elucidation of the role of DACT2 in adipogenesis may further our understanding of non-canonical Wnt signalling in obesity and how modulation by LC n-3 PUFAs affects adipogenesis in scWAT.
In addition to modulation of Wnt signalling, LC n-3 PUFAs upregulated the expression of genes associated with negative regulation of cell proliferation and cytokine mediated signalling in normal weight individuals, suggesting inhibition of inflammation. However, in individuals living with obesity, LC n-3 PUFAs upregulated the expression of genes associated with promoting cell differentiation and blood vessel remodelling suggesting intervention with these lipids may help improve the scWAT environment under circumstances of excess lipid accumulation and ECM restriction.
LC n-3 PUFA intervention did not alter scWAT morphological parameters. Changes to adipocyte size were not expected as there was no fat loss (data not shown) following the intervention period. In contrast to previous reports in scWAT of non-diabetic individuals with impaired glucose tolerance (61) and in visceral adipose tissue (62), the current study did not observe a reduction in scWAT macrophages in response to LC n-3 PUFAs. Despite this, we previously reported the downregulation of inflammatory and immune signalling in both groups of individuals with LC n-3 PUFA intervention (30).
Strength and Limitations
The current study has several strengths including its sample size, compliance to the intervention which was >90%, and the careful phenotyping of the individuals. We have shown that 12 weeks of 1.9 g of EPA + DHA daily was adequate to increase EPA and DHA in human scWAT and to alter transcriptome profiles in both normal weight individuals and individuals living with obesity and that this has a novel influence on Wnt signalling. This dose of EPA + DHA could be achieved amongst the general population by diet (e.g. several servings of fatty fish weekly) or a combination of diet and supplementation.
A limitation of this study is that the study may be underpowered. A target group size of 40 normal weight individuals and 40 individuals living with obesity was required for the study to be appropriately powered to detect changes in circulating cytokines (using IL-6 as the primary outcome) at > 80% power and a 5% level of significance. There was no formal power calculation for the outcomes described in the current paper, and so it may be possible that the number of scWAT samples analysed from normal weight individuals was a limitation for some of the outcomes reported herein.
Conclusion
In summary, the current study provides novel evidence for an altered transcriptome profile and tissue morphology in scWAT in obesity prior to manifestation of metabolic syndrome indicative of tissue hypertrophy, hypoxia, inflammatory signalling and macrophage infiltration, and remodelling, and for altered responses to LC n-3 PUFA according to adiposity. We report correlations between HOMA2-IR and scWAT fibrosis and WNT mRNA expression, as well as correlations between circulating IL-6 concentrations, macrophage infiltration and WNT mRNA expression suggesting an important role for metabolic health in addition to obesity. We highlight the dysregulation of scWAT Wnt signalling in human obesity and provide novel insights into the dysregulation of both canonical and non-canonical signalling. In addition, we report for the first time modulation of DACT2 mRNA expression by LC n-3 PUFAs which may have beneficial effects on dysregulated Wnt signalling in individuals living with obesity.
We provide novel evidence that scWAT is responsive to dietary manipulation with LC n-3 PUFAs and that potential beneficial changes to the tissue environment through the modulation of inflammatory and remodelling pathways can be achieved within 12-weeks. Furthermore, we provide novel evidence for altered responses to LC n-3 PUFAs in human obesity. Higher doses of LC n-3 PUFAs and/or longer duration of the intervention period may result in morphological changes such as a decrease in the number of CLS and adipocyte size, which would be beneficial to individuals living with obesity.
Data Availability Statement
The datasets presented in this study can be found in online repositories. The names of the repository/repositories and accession number(s) can be found below: https://www.ncbi.nlm.nih.gov/geo/, GSE162653.
Ethics Statement
The studies involving human participants were reviewed and approved by National Research Ethics Service South Central–Berkshire Research Ethics Committee (submission no. 11/SC/0384). The patients/participants provided their written informed consent to participate in this study.
Author Contributions
HF: data curation, formal analysis, investigation, methodology, writing - original draft, and writing - review and editing. CC: formal analysis, project administration, and writing - review and editing. EM: conceptualisation and writing - review and editing. RA: formal analysis and writing - review and editing. PN: formal analysis, project administration, and writing - review and editing. CP-C: investigation, project administration, and writing - review and editing. EA: data curation and writing - review and editing. KL: data curation and writing - review and editing. PC: conceptualisation, funding acquisition, supervision, and writing - review and editing. All authors contributed to the article and approved the submitted version.
Funding
European Commission, Seventh Framework Programme (Grant Number 244995).
Conflict of Interest
PCC undertakes unpaid voluntary work as the current President of the Federation of European Nutrition Societies (FENS) and as Past President of ILSI Europe.
The remaining authors declare that the research was conducted in the absence of any commercial or financial relationships that could be construed as a potential conflict of interest.
Publisher’s Note
All claims expressed in this article are solely those of the authors and do not necessarily represent those of their affiliated organizations, or those of the publisher, the editors and the reviewers. Any product that may be evaluated in this article, or claim that may be made by its manufacturer, is not guaranteed or endorsed by the publisher.
Acknowledgments
We acknowledge the European Commission for providing funding through its Seventh Framework Programme (grant number 244995) and EPAX, Norway, for the supply of the fish oil and corn oil capsules used in the study. We acknowledge Dr Jaswinder Sethi at the University of Southampton for her intellectual input, Dr Susan Wilson and Jenny Norman at the Histochemistry Research Unit at Southampton General Hospital for their support with sample preservation and histological technique training, and Dr David Johnston and Dr David Chatelet at the Biomedical Imaging Unit at Southampton General Hospital for their support with image analysis training.
Abbreviations
Dishevelled binding antagonist of beta catenin, (DACT, alias DAPPER1); triglyceride, (TG); Hypoxia inducible factor-1α, (HIF-1α); Extracellular matrix, (ECM); Subcutaneous WAT, (scWAT); Matrix metalloproteinases, (MMPs); Interleukin-6, (IL-6); Eicosapentaenoic acid, (EPA); Long chain omega-3 polyunsaturated fatty acids, (LC n-3 PUFAs); Peroxisome proliferator activated receptors, (PPARs); High density lipoprotein cholesterol, (HDL-C); Low density lipoprotein cholesterol, (LDL-C); Homeostasis model assessment 2 of insulin resistance, (HOMA2-IR); Fatty acid methyl esters, (FAMEs); RNA integrity, (RIN); Counts per million, (CPM); Interquartile range, (IQR); Ingenuity pathway analysis, (IPA); Vascular endothelial growth factor, (VEGF); EGF like domain multiple 6, (EGFL6); Growth/differentiation factor-15, (GDF15); Wnt family member-3A, (WNT3A); Wnt family member-10B, (WNT10B); Crown like structures, (CLS).
References
1. Daryabor G, Kabelitz D, Kalantar K. An Update on Immune Dysregulation in Obesity-Related Insulin Resistance. Scand J Immunol (2019) 89:e12747. doi: 10.1111/sji.12747
2. Salans LB, Cushman SW, Weismann RE. Studies of Human Adipose Tissue, Adipose Cell Size and Number in Nonobese and Obese Patients. J Clin Invest (1973) 52:929–41. doi: 10.1172/JCI107258
3. Drolet R, Richard C, Sniderman AD, Mailloux J, Fortier M, Huot C, et al. Hypertrophy and Hyperplasia of Abdominal Adipose Tissues in Women. Int J Obes (Lond) (2008) 32:283–91. doi: 10.1038/sj.ijo.0803708
4. Verboven K, Wouters K, Gaens K, Hansen D, Bijnen M, Wetzels S, et al. Abdominal Subcutaneous and Visceral Adipocyte Size, Lipolysis and Inflammation Relate to Insulin Resistance in Male Obese Humans. Sci Rep (2018) 8:4677. doi: 10.1038/s41598-018-22962-x
5. Belligoli A, Compagnin C, Sanna M, Favaretto F, Fabris R, Busetto L, et al. Characterization of Subcutaneous and Omental Adipose Tissue in Patients With Obesity and With Different Degrees of Glucose Impairment. Sci Rep (2019) 9:11333. doi: 10.1038/s41598-019-47719-y
6. Lee MJ, Wu Y, Fried SK. Adipose Tissue Remodeling in Pathophysiology of Obesity. Curr Opin Clin Nutr Metab Care (2010) 13:371–6. doi: 10.1097/MCO.0b013e32833aabef
7. Halberg N, Khan T, Trujillo ME, Wernstedt-Asterholm I, Attie AD, Sherwani S, et al. Hypoxia-Inducible Factor 1alpha Induces Fibrosis and Insulin Resistance in White Adipose Tissue. Mol Cell Biol (2009) 29:4467–83. doi: 10.1128/MCB.00192-09
8. Pasarica M, Sereda OR, Redman LM, Albarado DC, Hymel DT, Roan LE, et al. Reduced Adipose Tissue Oxygenation in Human Obesity: Evidence for Rarefaction, Macrophage Chemotaxis, and Inflammation Without an Angiogenic Response. Diabetes (2009) 58:718–25. doi: 10.2337/db08-1098
9. Marcelin G, Gautier EL, Clement K. Adipose Tissue Fibrosis in Obesity: Etiology and Challenges. Annu Rev Physiol (2022) 84:135–55. doi: 10.1146/annurev-physiol-060721-092930
10. Johnson A, Milner J, Makowski L. The Inflammation Highway: Metabolism Accelerates Inflammatory Traffic in Obesity. Immunol Rev (2012) 249:218–38. doi: 10.1111/j.1600-065X.2012.01151.x
11. Sun K, Tordjman J, Clement K, Scherer PE. Fibrosis and Adipose Tissue Dysfunction. Cell Metab (2013) 18:470–7. doi: 10.1016/j.cmet.2013.06.016
12. Chen N, Zhou L, Zhang Z, Xu J, Wan Z, Qin L. Resistin Induces Lipolysis and Suppresses Adiponectin Secretion in Cultured Human Visceral Adipose Tissue. Regul Peptides (2014) 194-195:49–54. doi: 10.1016/j.regpep.2014.10.001
13. Masoodi M, Kuda O, Rossmeisl M, Flachs P, Kopecky J. Lipid Signaling in Adipose Tissue: Connecting Inflammation & Metabolism. Biochim Biophys Acta (2014) 1851:503–18. doi: 10.1016/j.bbalip.2014.09.023
14. Bessesen DH, Cox-York KA, Hernandez TL, Erickson CB, Wang H, Jackman MR, et al. Postprandial Triglycerides and Adipose Tissue Storage of Dietary Fatty Acids: Impact of Menopause and Estradiol. Obesity (2015) 23:145–53. doi: 10.1002/oby.20935
15. Jo J, Gavrilova O, Pack S, Jou W, Mullen S, Sumner AE, et al. Hypertrophy and/or Hyperplasia: Dynamics of Adipose Tissue Growth. PLoS Comput Biol (2009) 5:e1000324. doi: 10.1371/journal.pcbi.1000324
16. Sethi JK, Vidal-Puig A. Wnt Signalling and the Control of Cellular Metabolism. Biochem J (2010) 427:1–17. doi: 10.1042/BJ20091866
17. Bennett CN, Ross SE, Longo KA, Bajnok L, Hemati N, Johnson KW, et al. Regulation of Wnt Signaling During Adipogenesis. J Biol Chem (2002) 277:30998–1004. doi: 10.1074/jbc.M204527200
18. Chen N, Wang J. Wnt/beta-Catenin Signaling and Obesity. Front Physiol (2018) 9:792. doi: 10.3389/fphys.2018.00792
19. Bagchi DP, Nishii A, Li Z, Delproposto JB, Corsa CA, Mori H, et al. Wnt/beta-Catenin Signaling Regulates Adipose Tissue Lipogenesis and Adipocyte-Specific Loss is Rigorously Defended by Neighboring Stromal-Vascular Cells. Mol Metab (2020) 42:101078. doi: 10.1016/j.molmet.2020.101078
20. Ruiz-Ojeda FJ, Mendez-Gutierrez A, Aguilera CM, Plaza-Diaz J. Extracellular Matrix Remodeling of Adipose Tissue in Obesity and Metabolic Diseases. Int J Mol Sci (2019) 20:4888. doi: 10.3390/ijms20194888
21. Hosogai N, Fukuhara A, Oshima K, Miyata Y, Tanaka S, Segawa K, et al. Adipose Tissue Hypoxia in Obesity and Its Impact on Adipocytokine Dysregulation. Diabetes (2007) 56:901–11. doi: 10.2337/db06-0911
22. Wang B, Wood IS, Trayhurn P. Dysregulation of the Expression and Secretion of Inflammation-Related Adipokines by Hypoxia in Human Adipocytes. Pflugers Arch (2007) 455:479–92. doi: 10.1007/s00424-007-0301-8
23. Tahergorabi Z, Khazaei M. The Relationship Between Inflammatory Markers, Angiogenesis, and Obesity. ARYA Atheroslet (2013) 9:247–53.
24. Pasarica M, Gowronska-Kozak B, Burk D, Remedios I, Hymel D, Gimble J, et al. Adipose Tissue Collagen VI in Obesity. J Clin Endocrinol Metab (2009) 94:5155–62. doi: 10.1210/jc.2009-0947
25. Henegar C, Tordjman J, Achard V, Lacasa D, Cremer I, Guerre-Millo M, et al. Adipose Tissue Transcriptomic Signature Highlights the Pathological Relevance of Extracellular Matrix in Human Obesity. Genome Biol (2008) 9:R14. doi: 10.1186/gb-2008-9-1-r14
26. Mutch DM, Tordjman J, Pelloux V, Hanczar B, Henegar C, Poitou C, et al. Needle and Surgical Biopsy Techniques Differentially Affect Adipose Tissue Gene Expression Profiles. Am J Clin Nutr (2009) 89:51–7. doi: 10.3945/ajcn.2008.26802
27. Debari MK, Abbott RD. Adipose Tissue Fibrosis: Mechanisms, Models, and Importance. Int J Mol Sci (2020) 21:6030. doi: 10.3390/ijms21176030
28. Calder PC. Very Long Chain Omega-3 (N-3) Fatty Acids and Human Health. Eur J Lipid Sci Technol (2014) 116:1280–300. doi: 10.1002/ejlt.201400025
29. Calder PC. Marine Omega-3 Fatty Acids and Inflammatory Processes: Effects, Mechanisms and Clinical Relevance. Biochim Biophys Acta (2015) 1851:469–84. doi: 10.1016/j.bbalip.2014.08.010
30. Fisk HL, Childs CE, Miles EA, Ayres R, Noakes PS, Paras-Chavez C, et al. Modification of Subcutaneous White Adipose Tissue Inflammation by Omega-3 Fatty Acids is Limited in Human Obesity-a Double Blind, Randomised Clinical Trial. EBioMedicine (2022) 77:103909. doi: 10.1016/j.ebiom.2022.103909
31. Mejia-Barradas CM, Del-Rio-Navarro BE, Dominguez-Lopez A, Campos-Rodriguez R, Martinez-Godinez M, Rojas-Hernandez S, et al. The Consumption of N-3 Polyunsaturated Fatty Acids Differentially Modulates Gene Expression of Peroxisome Proliferator-Activated Receptor Alpha and Gamma and Hypoxia-Inducible Factor 1 Alpha in Subcutaneous Adipose Tissue of Obese Adolescents. Endocrine (2014) 45:98–105. doi: 10.1007/s12020-013-9941-y
32. Larsen TM, Toubro S, Astrup A. PPARgamma Agonists in the Treatment of Type II Diabetes: Is Increased Fatness Commensurate With Long-Term Efficacy? Int J Obes Relat Metab Disord (2003) 27:147–61. doi: 10.1038/sj.ijo.802223
33. Zhang K, Chang Y, Shi Z, Han X, Han Y, Yao Q, et al. Omega-3 PUFAs Ameliorate Liver Fibrosis and Inhibit Hepatic Stellate Cells Proliferation and Activation by Promoting YAP/TAZ Degradation. Sci Rep (2016) 6:30029. doi: 10.1038/srep30029
34. Tanaka N, Sano K, Horiuchi A, Tanaka E, Kiyosawa K, Aoyama T. Highly Purified Eicosapentaenoic Acid Treatment Improves Nonalcoholic Steatohepatitis. J Clin Gastroenterol (2008) 42:413–8. doi: 10.1097/MCG.0b013e31815591aa
35. Burger B, Kuhl CMC, Candreva T, Cardoso RDS, Silva JR, Castelucci BG, et al. Oral Administration of EPA-Rich Oil Impairs Collagen Reorganization Due to Elevated Production of IL-10 During Skin Wound Healing in Mice. Sci Rep (2019) 9.9119 doi: 10.1038/s41598-019-45508-1
36. Fisk HL, Childs CE, Miles EA, Ayres R, Noakes PS, Paras-Chavez C, et al. Dysregulation of Endocannabinoid Concentrations in Human Subcutaneous Adipose Tissue in Obesity and Modulation by Omega-3 Polyunsaturated Fatty Acids. Clin Sci (Lond) (2021) 135:185–200. doi: 10.1042/CS20201060
37. Huang DW, Sherman BT, Lempicki RA. Systematic and Integrative Analysis of Large Gene Lists Using DAVID Bioinformatics Resources. Nat Protoc (2009) 4:44–57. doi: 10.1038/nprot.2008.211
38. Sherman B, Hao M, Qiu J, Jiao X, Baseler M, Lane H, et al. DAVID: A Web Server for Functional Enrichment Analysis and Functional Annotation of Gene Lists. Nucleic Acids Res (2022). doi: 10.1093/nar/gkac194
39. Subramanian A, Tamayo P, Mootha V, Mukherjee S, Ebert B, Gillette M, et al. Gene Set Enrichment Analysis: A Knowledge-Based Approach for Interpreting Genome-Wide Expression Profiles. PNAS (2005) 102:15545–50. doi: 10.1073/pnas.0506580102
40. Mootha VK, Lindgren CM, Karl-Fredrik E, Aravind S, Smita S, Joseph L, et al. PGC-1α-Responsive Genes Involved in Oxidative Phosphorylation are Coordinately Downregulated in Human Diabetes. Nat Genet (2003) 34:267–73. doi: 10.1038/ng1180
41. Yasuhara R, Yuasa T, Williams JA, Byers SW, Shah S, Pacifici M, et al. Wnt/β-Catenin and Retinoic Acid Receptor Signaling Pathways Interact to Regulate Chondrocyte Function and Matrix Turnover*. J Biol Chem (2010) 285:317–27. doi: 10.1074/jbc.M109.053926
42. Cadigan KM, Waterman ML. TCF/LEFs and Wnt Signaling in the Nucleus. Cold Spring Harb Perspect Biol (2012) 4:a007906. doi: 10.1101/cshperspect.a007906
43. Del Bosque-Plata L, Martínez-Martínez E, Espinoza-Camacho MÁ, Gragnoli C. The Role of TCF7L2 in Type 2 Diabetes. Diabetes (2021) 70:1220–8. doi: 10.2337/db20-0573
44. Fuster JJ, Zuriaga MA, Ngo DT, Farb MG, Aprahamian T, Yamaguchi TP, et al. Noncanonical Wnt Signaling Promotes Obesity-Induced Adipose Tissue Inflammation and Metabolic Dysfunction Independent of Adipose Tissue Expansion. Diabetes (2015) 64:1235–48. doi: 10.2337/db14-1164
45. Relling I, Akcay G, Fangmann D, Knappe C, Schulte DM, Hartmann K, et al. Role of Wnt5a in Metabolic Inflammation in Humans. J Clin Endocrinol Metab (2018) 103:4253–64. doi: 10.1210/jc.2018-01007
46. Cawthorn WP, Heyd F, Hegyi K, Sethi JK. Tumour Necrosis Factor-Alpha Inhibits Adipogenesis via a Beta-Catenin/TCF4(TCF7L2)-Dependent Pathway. Cell Death Differ (2007) 14:1361–73. doi: 10.1038/sj.cdd.4402127
47. Christodoulides C, Lagathu C, Sethi JK, Vidal-Puig A. Adipogenesis and WNT Signalling. Trends Endocrinol Metab (2009) 20:16–24. doi: 10.1016/j.tem.2008.09.002
48. de Winter TJJ, Nusse R. Running Against the Wnt: How Wnt/β-Catenin Suppresses Adipogenesis. Front Cell Dev Biol (2021) 9. doi: 10.3389/fcell.2021.627429
49. Heinonen S, Muniandy M, Buzkova J, Mardinoglu A, Rodriguez A, Fruhbeck G, et al. Mitochondria-Related Transcriptional Signature is Downregulated in Adipocytes in Obesity: A Study of Young Healthy MZ Twins. Diabetologia (2017) 60:169–81. doi: 10.1007/s00125-016-4121-2
50. van der Kolk BW, Saari S, Lovric A, Arif M, Alvarez M, Ko A, et al. Molecular Pathways Behind Acquired Obesity: Adipose Tissue and Skeletal Muscle Multiomics in Monozygotic Twin Pairs Discordant for BMI. Cell Rep Med (2021) 2:100226. doi: 10.1016/j.xcrm.2021.100226
51. Kaartinen MT, Hang A, Barry A, Arora M, Heinonen S, Lundbom J, et al. Matrisome Alterations in Obesity - Adipose Tissue Transcriptome Study on Monozygotic Weight-Discordant Twins. Matrix Biol (2022) 108:1–19. doi: 10.1016/j.matbio.2022.02.005
52. Bilson J, Scorletti E, Bindels LB, Afolabi PR, Targher G, Calder PC, et al. Growth Differentiation Factor-15 and the Association Between Type 2 Diabetes and Liver Fibrosis in NAFLD. Nutr Diabetes (2021) 11:32. doi: 10.1038/s41387-021-00170-3
53. Rundhaug JE. Matrix Metalloproteinases and Angiogenesis. J Cell Mol Med (2005) 9:267–85. doi: 10.1111/j.1582-4934.2005.tb00355.x
54. Maquoi E, Munaut C, Colige A, Collen D, Lijnen HR. Modulation of Adipose Tissue Expression of Murine Matrix Metalloproteinases and Their Tissue Inhibitors With Obesity. Diabetes (2002) 51:1093–101. doi: 10.2337/diabetes.51.4.1093
55. Ress C, Tschoner A, Ciardi C, Laimer MW, Engl JW, Sturm W, et al. Influence of Significant Weight Loss on Serum Matrix Metalloproteinase (MMP)-7 Levels. Eur Cytokine Netw (2010) 21:65–70. doi: 10.1684/ecn.2009.0177
56. Yabluchanskiy A, Ma Y, Iyer RP, Hall ME, Lindsey ML. Matrix Metalloproteinase-9: Many Shades of Function in Cardiovascular Disease. Physiol (Bethesda) (2013) 28:391–403. doi: 10.1152/physiol.00029.2013
57. Spencer M. Adipose Tissue Macrophages in Insulin-Resistant Subjects are Associated With Collagen VI and Fibrosis and Demonstrate Alternative Activation. Am J Physiol - Endocrinol Metab (2010) 299:E1016-27. doi: 10.1152/ajpendo.00329.2010
58. Davis KE, Moldes M, Farmer SR. The Forkhead Transcription Factor FoxC2 Inhibits White Adipocyte Differentiation. J Biol Chem (2004) 279:42453–61. doi: 10.1074/jbc.M402197200
59. Myneni VD, Melino G, Kaartinen MT. Transglutaminase 2–a Novel Inhibitor of Adipogenesis. Cell Death Dis (2015) 6:e1868. doi: 10.1038/cddis.2015.238
60. Suryawanshi A, Tadagavadi RK, Swafford D, Manicassamy S. Modulation of Inflammatory Responses by Wnt/beta-Catenin Signaling in Dendritic Cells: A Novel Immunotherapy Target for Autoimmunity and Cancer. Front Immunol (2016) 7:460. doi: 10.3389/fimmu.2016.00460
61. Spencer M, Finlin BS, Unal R, Zhu B, Morris AJ, Shipp LR, et al. Omega-3 Fatty Acids Reduce Adipose Tissue Macrophages in Human Subjects With Insulin Resistance. Diabetes (2013) 62:1709–17. doi: 10.2337/db12-1042
Keywords: obesity, LC n-3 PUFA, adipose tissue, inflammation, tissue remodelling, Wnt signalling
Citation: Fisk HL, Childs CE, Miles EA, Ayres R, Noakes PS, Paras-Chavez C, Antoun E, Lillycrop KA and Calder PC (2022) Dysregulation of Subcutaneous White Adipose Tissue Inflammatory Environment Modelling in Non-Insulin Resistant Obesity and Responses to Omega-3 Fatty Acids – A Double Blind, Randomised Clinical Trial. Front. Immunol. 13:922654. doi: 10.3389/fimmu.2022.922654
Received: 18 April 2022; Accepted: 20 June 2022;
Published: 25 July 2022.
Edited by:
Amiram Ariel, University of Haifa, IsraelReviewed by:
Joan Clària, Hospital Clinic of Barcelona, SpainPaula Longhi, Queen Mary University of London, United Kingdom
Copyright © 2022 Fisk, Childs, Miles, Ayres, Noakes, Paras-Chavez, Antoun, Lillycrop and Calder. This is an open-access article distributed under the terms of the Creative Commons Attribution License (CC BY). The use, distribution or reproduction in other forums is permitted, provided the original author(s) and the copyright owner(s) are credited and that the original publication in this journal is cited, in accordance with accepted academic practice. No use, distribution or reproduction is permitted which does not comply with these terms.
*Correspondence: Helena L. Fisk, h.fisk@soton.ac.uk