- 1Oncology Department, Shandong Second Provincial General Hospital, Jinan, China
- 2Key Laboratory of Marine Drugs, Ministry of Education, School of Medicine and Pharmacy, Ocean University of China, Qingdao, China
Image-guided tumor ablation eliminates tumor cells by physical or chemical stimulation, which shows less invasive and more precise in local tumor treatment. Tumor ablation provides a treatment option for medically inoperable patients. Currently, clinical ablation techniques are widely used in clinical practice, including cryoablation, radiofrequency ablation (RFA), and microwave ablation (MWA). Previous clinical studies indicated that ablation treatment activated immune responses besides killing tumor cells directly, such as short-term anti-tumor response, immunosuppression reduction, specific and non-specific immune enhancement, and the reduction or disappearance of distant tumor foci. However, tumor ablation transiently induced immune response. The combination of ablation and immunotherapy is expected to achieve better therapeutic results in clinical application. In this paper, we provided a summary of the principle, clinical application status, and immune effects of tumor ablation technologies for tumor treatment. Moreover, we discussed the clinical application of different combination of ablation techniques with immunotherapy and proposed possible solutions for the challenges encountered by combined therapy. It is hoped to provide a new idea and reference for the clinical application of combinate treatment of tumor ablation and immunotherapy.
Introduction
The cancer incidence and mortality have been rising rapidly in China, with about 4.29 million new cases and 2.81 million cancer deaths in 2015 according to China National Cancer Center (NCC) statistics (1). Malignant tumor has become a major public health problem nowadays. Radiotherapy and chemotherapy is still the predominant treatment options for advanced malignant tumors after surgery nowadays (2). Most cancer patients often suffer recurrence and distant metastasis, eventually cancer-related death, although respond to initial radiotherapy and chemotherapy. With the deep research of tumor immunity, immunotherapy is a hotspot of medicine currently, represented by immune checkpoint inhibitors (ICIs), adoptive cell transfer therapy, tumor-specific vaccines and small molecule immune drugs (3). ICIs have been widely used in cancer treatment, such as anti-PD-1/PD-L1 and anti-CTLA-4 monoclonal antibodies (mAb), whereas limited therapeutic effects in those with poor PD-L1 expression, lymphocyte deficiency, immune effector cells failed to infiltrate into tumor microenvironment (TME), insufficient host recognition of tumor antigen and so on (4). A large percentage of cancer patients still benefit little form clinical treatment, with a single-agent effective rate of only 20%-40% (5). Therefore, urgent clinical needs to explore new therapeutic strategies have addressed the aforementioned therapeutic limitations.
Ablation technology induces tumor tissue coagulative necrosis and achieves local elimination of tumor with physical and chemical methods. The ablation techniques include cryoablation, chemical ablation, laser ablation, radiofrequency ablation (RFA), microwave ablation (MWA), high-intensity focused ultrasound (HIFU), and combined technology (6). Currently, the therapeutic application of ablation technology has been widely implemented in the clinical practice, which also achieved a broad consensus and guidelines on its specific administration plans and strategies (Figure 1). As a minimally invasive treatment technology (except for high-intensity focused ultrasound), ablation treatment can effectively promote the exposure and release of tumor-related antigens, enhance the antigenicity of tumors, and eventually trigger a systemic antitumor immune response, which may eliminate distant metastatic lesions (7). Ablation techniques provide an optional choice for the patients with tumor oligolesion, especially those cannot withstand surgery, while potentially increasing the therapeutic effects of immunotherapy in clinical practice.
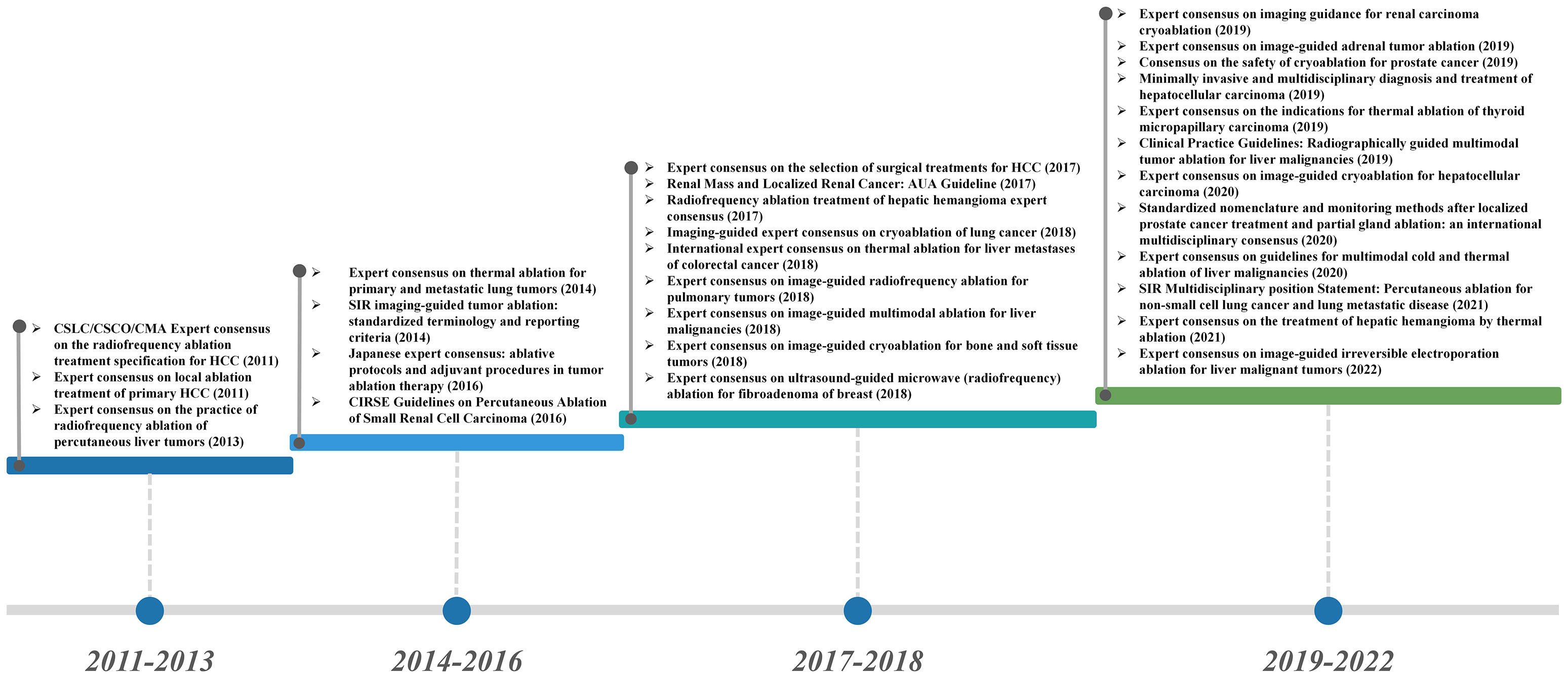
Figure 1 Guidelines and consensus for the application of ablation in the clinical treatment of cancer.
In this paper, we focused the three common used ablation technologies, including cryoablation, RFA and MWA. We reviewed the literature of ablation techniques and related immune activities, and the combination treatment of ablation and immunotherapy. We would like to provide new ideas and reference for the implementation of the combination of ablation techniques and immunotherapy.
The principle of the ablation techniques
Imaging-guided ablation is a precise and minimally invasive cancer treatment technique, which cures tumors by physical or chemical energy safely. Currently, cryoablation, RFA and MWA are widely used in clinical practice (Table 1).The characteristics of tumor ablation include: ① Ablation equipment can accurately target tumor tissue with small wound, avoiding wound infection and bleeding (8–10). ② Ablation treatment shows a clear therapeutic boundary, which is generally set near the edge of tumor tissue about 1 cm (11). This range can not only effectively kill local tumor tissues, but also avoid the excessive destroy in adjacent normal tissues (12–14). ③ Ablation usually induces different degrees of autoimmune responses to inhibit tumor recurrence and metastasis (15).
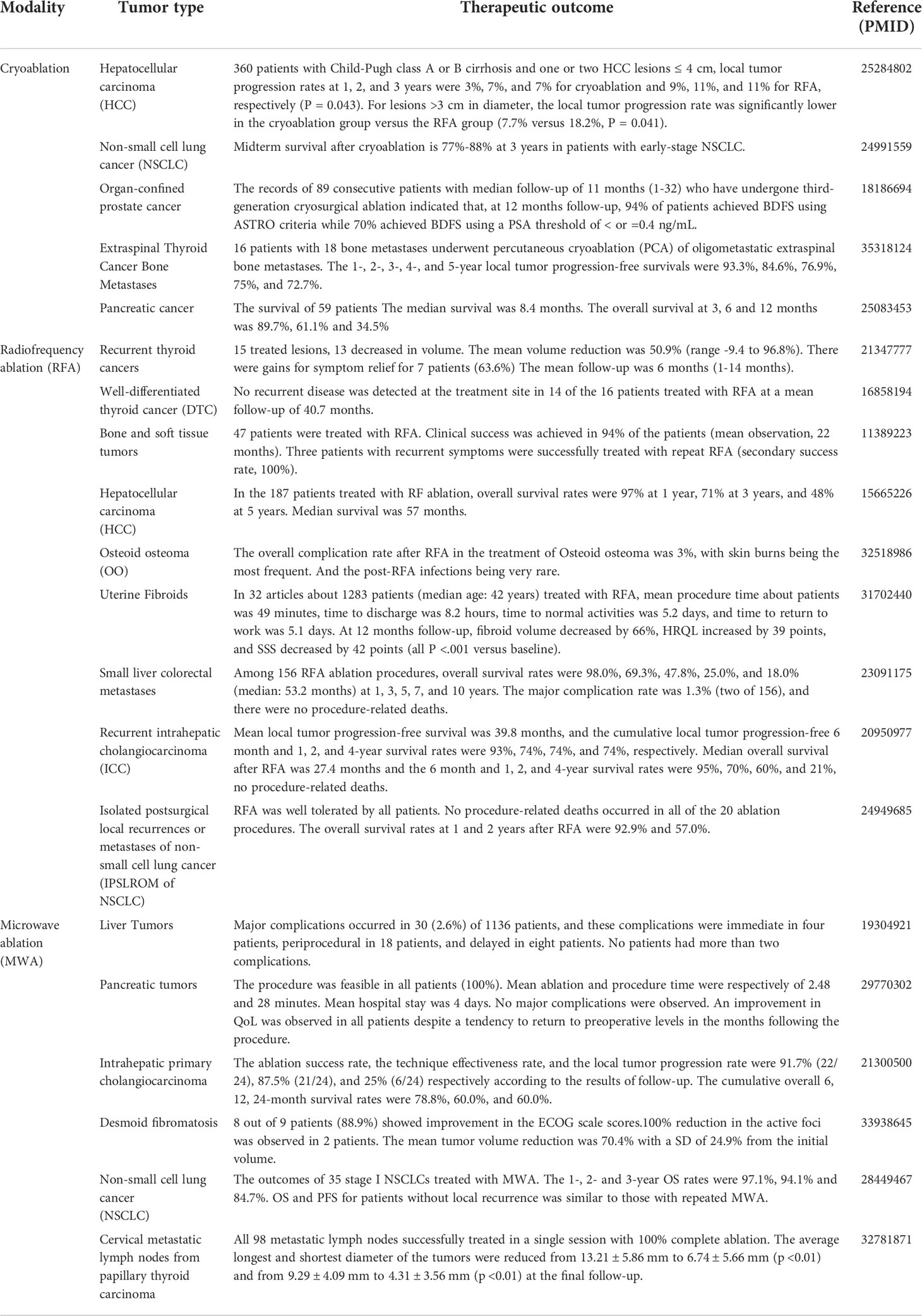
Table 1 Selected publications on the clinical application outcomes of Cryoablation, Radiofrequency ablation (RFA), and Microwave ablation (MWA).
Cryoablation
Cryoablation kills tumor cells with low temperature environment, which changes the osmotic pressure and causes tissue ischemia. The critical temperature for cell death is -20°C. Various factors influenced tumor death rate, such as freezing duration, freeze-thaw cycle and local blood flow changes (16). Cryoablation technology uses an ablation probe to introduce liquid nitrogen or argon into the tumor tissue, forming an “ice ball” near the cryoprobe, with the center temperature reaching -170°C and about 0°C in the periphery of “isotherm”. The high-pressure gas induces a freezing cycle process, in which the temperature rapidly decreases and then increases in the tumor tissue according to the Joule-Thomson principle (17). Due to the rapidly decreased temperature inside and outside cells, ice crystal leads to cell dehydration in adjacent tissues, inducing cell permeability damage and cell membrane irreversible damage, and ultimately tumor necrosis (18). Low temperature also damages the intima of microveins and arterioles in the tumor tissues. The damaged endothelial cells contact platelets to form thrombus after ice crystal thawing, which will further develop ischemia and hypoxia in the tumor tissues (19, 20).
The advantages of cryoablation are summarized as the following aspects: ① Ice crystal can be imaged by computed tomography (CT) to achieve accurate treatment area and intuitive monitoring and evaluation of the therapeutic effects during cryoablation (16). ② Cryoablation effectively reduces the recurrence rate of tumor. In SOLSTICE study, 128 post-cryoablation patients were followed up at the 12th and 24th month respectively. The local recurrence-free survival (RFS) rate after cryoablation was 85.1% and 77.2% after initial treatment. The local RFS rate of the recurrent tumor after the secondary cryoablation was even increased to 91.1% and 84.4% (21). ③Cryoablation shows significant pain alleviation effects. Previous studies indicated that cryoablation decreased the analgesics dosage in the treatment of localized tumor foci (≤4cm), which was better than RFA (22). Matthew R et al. summarized the most severe cancer pain assessment of 69 patients with bone metastasis before and after cryoablation, which found that the average score within 24 hours prior to cryoablation was 7.1/10, with a range of 4/10 to 10/10. The mean scores of 1, 4, 8, and 24 weeks after treatment decreased to 5.1/10 (p<0.001), 4.0/10 (p <0.001), 3.6/10 (p <0.001), and 1.4/10 (p <0.001) respectively. Cryoablation treatment provides sustain relief for bone metastatic pain (23). In a palliative treatment of bronchial cancer, cryoablation also effectively relieved the severe pain and hemoptysis which caused by bronchus blockage, and restored the bronchial ventilation again (24). Cryoablation has been widely used in the treatment of patients with early hepatocellular carcinoma (HCC), non-small cell lung cancer (NSCLC), pleural lesions, palliative treatment of lung metastatic foci, which achieved good clinical outcome (25, 26). A phase I clinical study enrolled 160 patients with NSCLC after cryoablation therapy. The average local tumor progression-free survival (PFS) interval was extended to 69 ± 2 months (27). Another study of cryoablation in recurrent pleural malignancy also showed a 3-year recurrence-free rate of local disease as 73.7% (28).
RFA
RFA is a physical ablation that can be performed with percutaneous puncture, laparotomy or laparoscopy (29). The radiofrequency applicator is inserted into a target lesion with imaging guidance, such as ultrasound or computed tomography (CT). The current induces ions agitation at a high frequency to generate frictional heat (90-120 ℃), which causes coagulation necrosis in tumor tissues (30). Meanwhile, RFA prevents blood supply and tumor metastasis with the blood vessels disruption. A large proportion of liver tumors are suitable for RFA treatment rather than surgery resection, because of multiple intrahepatic lesions and other extrahepatic diseases (31). RFA is an optional technique for treating primary HCCs, as well as intrahepatic metastatic foci of colorectal or gastric cancer (32).
RFA exhibits significant advantages of minimal invasion, short recovery period in clinical practice. HCC patients after RFA treatment show low recurrence and mortality rate, which achieves similar curative effect as surgery to some extent (33–36). Previous studies also supported low recurrence rate and only mild adverse reactions, including fever, neutrophils, local pain and minor thermal damage in peripheral organs (37–39).
MWA
MWA is another novel tumor ablation technology which developed from biologic thermal effects, which is mainly applied in the treatment of solid tumors in liver (40), lung (41), kidney (42), adrenal gland (43), spleen (44), thyroid (45) and breast (46). The therapeutic effects of MWA depends on the dipolar molecules (primarily water) to align and realign according to the variable electromagnetic field. The frictional heating induces tissue degeneration attributed to highly interdependent antenna–tissue interactions, which is different from RFA. So MWA is suitable for increased maximal local ablation deposition in clinical practice (47).
MWA treatment is safe and effective in local lesion treatment, which showed well tolerance for the patients. The incidence of serious complications after MWA is only 3.65%, mild complications as 8.03%, and the mortality rate is 0% according previous study (48). Both MWA and RFA are widely used in the treatment of liver tumors, while MWA is more appropriate for larger diameter liver malignancy than RFA. RFA treatment causes local tissue dehydration and carbonization to prevent heat conduction to the adjacent tumor tissues, while WMA oscillates water molecules to cause tissue heating, which expands the coagulation zone in tumor tissues (49). Medhat et al. evaluated 26 HCC patients with large lesions (5-7cm in diameter) with MWA treatment and found that 19/26 patients (73.1%) achieved complete ablation, while no ablation-related major complications or death (50).
Immune effects of different ablation technologies
Tumor ablation induces immune effects by releasing immunogenic components within the tumor. Due to different ways of ablation technologies, various types and quantities of damage-associated molecular patterns (DAMPs) are released to induce different immune effects (51). Here, the differences in immune response are reviewed among different technologies.
Immunology of cryoablation
The tumor-specific immune response caused by cryoablation is commonly known as “cryoimmune response” (52). Released tumor cell contents during ablation treatment stimulate autoimmune response, and eliminate immunosuppression effect to kill tumors. Meanwhile, the antigenicity of tumor tissue necrosis activity also stimulates immune response in vivo to cure distant tumor foci, which commonly known as “distal immune effect”. Previous studies have shown that tumor-specific immune responses contribute to spontaneous regression of distant metastases in patients received cryoablation treatment (53). Zhu et al. reported that cryoablation treatment causes DAMPs release in the center of tumor lesion by ultra-low temperature to induce tissue destruction (54), including DNA, heat shock protein (HSP), tumor antigens, cytokines and inflammatory factors (55). DAMPs phagocytosis activates NF-κB pathway to induce anti-tumor immune response of immature dendritic cells (DCs). Mature DCs translocate to lymph nodes with the help of MHC class I molecules, activating CD8+ T cells and costimulatory factor CD80/86 expression by presenting antigen. The immune system finally eliminates residual tumor lesions and distant metastases (56). Kato et al. analyzed the renal tumor samples after cryoablation treatment and found a significant increase in the proportion of T cell β receptor (TCRB) clones (defined as clones with a frequency ≥ 1% of total TCRB reads). The TCRB clones were from 27.3% ± 27.4% to 46.4% ± 31.0% after ablation (P = 0.024), the transcription levels of granase A and CD11c in CD4+ T cells were also significantly increased in tumor tissues (57). Other study also supported increased levels of interleukin-1 (IL-1), IL-6, NF-κB and tumor necrosis factor α (TNF-α) after cryoablation (30). Of note, IL-6 shows the most significant increase after cryoablation than RFA and MWA (58). The main reasons are summarized as follows: ① Thermal ablation causes protein denaturation in tumor tissues, and reduces the tumor antigen amounts. Meanwhile, tissue coagulation by thermal ablation reduces the release of intracellular content into circulatory system. ② Cryoablation treatment increases cell membrane permeability while maintains the intact cell structure. ③ Cryoablation increases the release of inflammatory intracellular fragments to activate immune cells, Systemic inflammatory response syndrome (SIRS) maybe observed in some serious cases, whereas rarely observed in thermal ablation technologies (30). Therefore, cryoablation showed a stronger immune response than the thermal ablation.
Immunology of RFA
RFA technology provides a high temperature in local tumor tissue lesions for therapeutic effects. The release of large amounts of DAMPs from necrotic tissue further affect tumor microenvironment, including RNA, DNA, HSP-70, HSP-90, High Mobility Group Protein B1 (HMGB1), C-reactive protein and uric acid, which is similar to cryoablation. Zerbini et al. found that cell fragments released from tumor tissues after RFA contributed to the maturation of antigen-presenting cells (APCs) and DCs in vivo, which further promoted the activation of the immune system (59). Increased plasma levels of IL-1, IL-6, IL-8 and TNF-α were also reported after RFA treatment (60). Dramatic morphological changes were observed in tumor tissues after RFA, which were characterized as four areas: application, central, transition, and reference tissue areas (61). High temperature from RFA induces cell necrosis and increased HSP-70 expression in the transition zone (61, 62). Elevated expression levels of HSP-70 and HSP-90 are also observed in animal tumor models after RFA treatment (63). HSP-70 plays a key role in the activation of innate and adaptive immune cells (64). Ryan Slovak et al. indicated that innate immune response in the early stage after RFA contributed a majority part in RFA related immune response, which was associated with recurrence rate (65). Increased HSP-70 expression in tumor peripheral tissues after RFA attracts NK cells infiltration and activation, constituting the innate immunity response induced by RFA (66).
The immunosuppressive microenvironment within the tumor tissue contributes to the poor prognosis of malignancy. Among them, regulatory T cells (Tregs) induce immunosuppressive effects and mitigate the function of cytotoxic T cells (CTLs) (67). RFA treatment improves the tumor immune microenvironment by increasing the infiltration of CD4+ and CD8+T cells and reducing Tregs proportion (68, 69). The proportions of Tregs in the tumor microenvironment and blood show significantly decrease in pancreatic ductal adenocarcinoma after RFA (70). RFA treatment attenuates the immunosuppressive effects in tumor microenvironment.
Immunology of MWA
Unlike cryoablation and RFA, no significant difference in HSP-70 expression after MWA treatment (51). However, the phenotype and number of T cells exhibit dramatic changes. Increased IL-12, IL-18 activates Inducible T Cell Co-stimulator (ICOS) pathway after MWA, which synergistically promotes Helper T cells (Th) differentiation into Th1 and increases IL-2 secretion, thus inducing the expansion of cytotoxic T cells (CTLs) related immune effect (71). Previous study showed increased percentage of T cells in peripheral blood at 1 week after MWA compared with surgery group (3.37 ± 30.31% vs. 19.42 ± 31.82%, p=0.033). All the studies support that WMA enhances the innate immune response (71).
The study of Zhang et al. observed decreased IL-4 and IL-10 levels after MWA, with IL-4 decreased from 0.13 ± 0.02 to 0.07 ± 0.01 pg/mL (p <0.05), and IL-10 decreased from 9.17 ± 2.97 to 3.93 ± 1.13 pg/mL (P <0.05) (72). So MWA inhibits Th2 cytokines secretion and reduces immunosuppressive factors in tumor microenvironment. Furthermore, ICOS expression on cell membrane after T cell activation also promotes antigen presentation of CD4 + T cells. Activated ICOS+ CD4+ T cells produce higher levels of interferon-γ (IFN-γ) (73). IFN-γ and other inflammatory factors contributes to the formation of Th1 type environment around tumor tissue, leading to immune response to eliminate tumor cells (74).
Combinate therapy of ablation with immunotherapy
Tumor ablation technology destroys tumor tissue with minimally invasion, which produces a large amount of tumor cell fragment within the tumor lesions. The released occult antigens activate immune system, including tumor-specific T cell response. The preclinical and clinical studies indicated a remarkable increase in T cell infiltration and activation after ablation therapy. However, the ablation-induced immune response appears to be insufficient to produce sustained antitumor effects. The combination of tumor ablation and immunotherapy has been further explored in clinical applications recently, which supports the combination strategies improved ablation-induced immune effect and achieved sustained anti-tumor immune effect (Table 2).
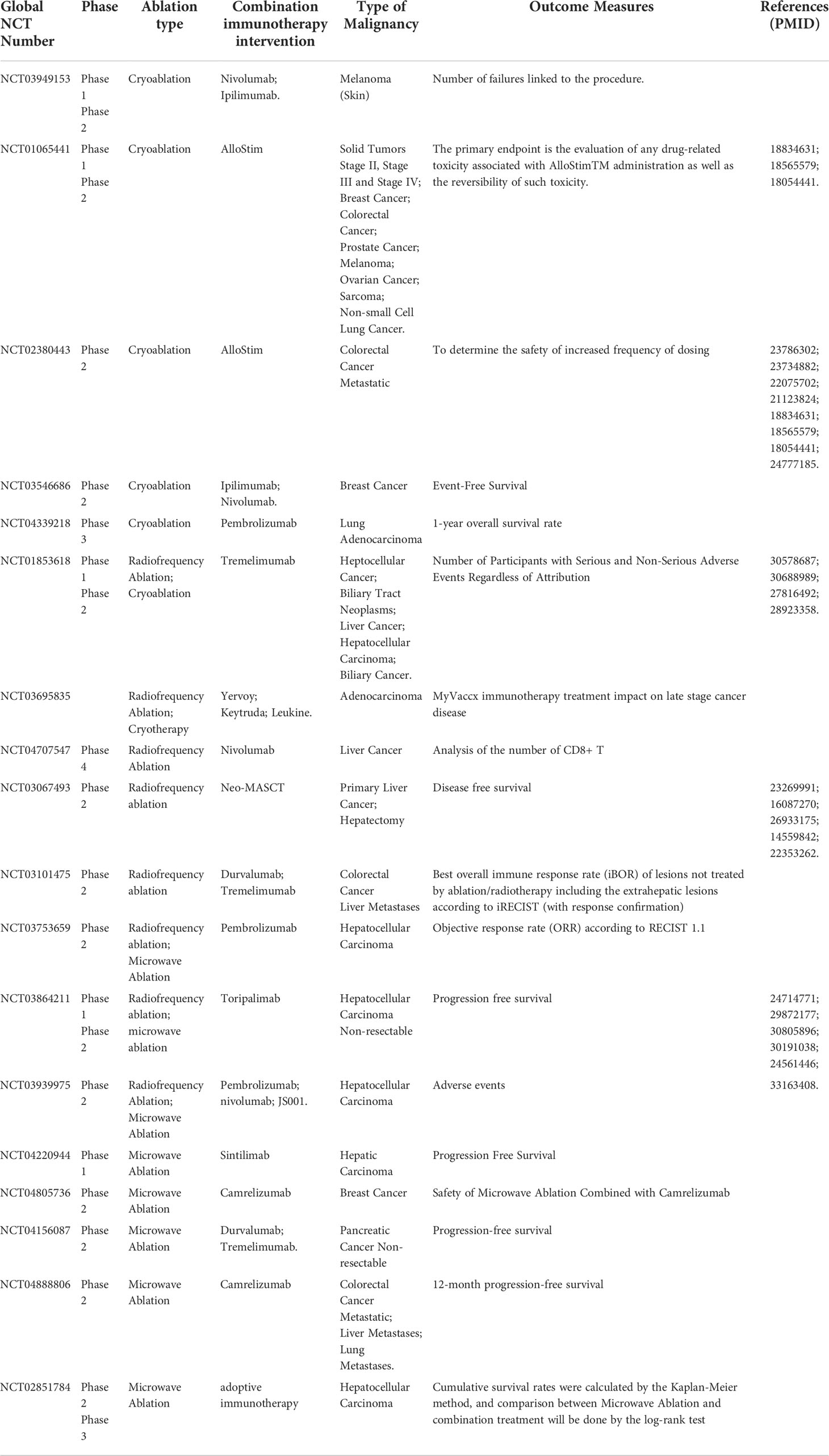
Table 2 Overview of clinical trials of cryoablation, radiofrequency ablation(RFA) and microwave ablation (MWA) combined with immunotherapy.
Cryoablation and immunotherapy
Immune checkpoint inhibitors (ICIs) achieves indelible mark in the field of tumor therapy, which exhibits remarkable safety and effects in clinical practice (75). The combination of ICIs and ablation aims to increase and prolong the antitumor immune effects. Present clinical studies usually selected the combination of anti-PD-1/PD-L1 or CTLA-4 mAb with ablation, which showed feasible safety and efficacy (76). McArthur et al. evaluated the safety of combinate treatment of cryoablation and Ipilimumab (CTLA-4 inhibitor) in 19 breast cancer patients. Only 1 patient suffered grade III toxicity symptoms (maculopapular rash unrelated to Ipilimumab treatment) (77). The combined treatment of cryoablation and Ipilimumab induced higher proportion of Ki67+ T cells and ICOShi T cells in peripheral blood than those only with cryoablation (p=0.05; p=0.005). The results suggest that combination therapy may bring on auto immune reaction and synergistic antitumor effect. A cervical cancer patient received Pembrolizumab after cryoablation, which showed a significant reduction of metastatic lesions (from 7.2 cm×6.8cm to 2.9 cm×1.5 cm) after two months treatment. Then the lesion was undetectable 3 months later and maintained complete response (CR) for 7 months (78). A retrospective study of cryotherapy combined with Pembrolizumab for liver metastatic melanoma, achieves superior efficacy to cryotherapy or immunotherapy alone. Cryotherapy improves the efficacy of anti-PD-1/PD-L1 mab in the 15 enrolled metastatic melanoma patients, with 1 patient (6.7%) achieved a complete response and 3 patients (20.0%) achieved a partial response. The objective response rate (ORR) of the combination regimen was 26.7% (95%CI 4.3-49.0%) and median PFS time was 4.0 months (95%CI 2.5-5.5) (79), which were significantly higher than only Pembrolizumab treatment (2.7 months) as Tumeh et al. reported (80). Moreover, grade 3-4 adverse events (AEs) were not observed in the combine treatment (79), while grade 3-5 AEs attributed to only Pembrolizumab treatment every two or three weeks occurred in 13.3%, 10.1% of advanced melanoma patients according to Caroline Robert et al. ‘s report (81). Cryoablation combined with Pembrolizumab is safe and effective than immunotherapy only.
DCs connects innate and acquired immune responses in tumor therapy. Toll-like receptor agonists activate and maturate DCs to uptake and present specific tumor antigens in microenvironment, which enabling acquired immune response (82). So combinate Toll-like receptor agonists with ablation technology means a promising strategy for enhancing anti-tumor immune response. Gaitanis et al. studied the combination treatment of cryoablation with Imiquimod (Toll-like receptor 7 agonist) for basal cell carcinoma, and only 1 patient (n = 21) suffered relapse after at least 18 months of follow-up with a cumulative efficacy of 95% (83).
Adoptive cell immunotherapy developed rapidly these years. The combination of tumor ablation and immune cell therapy is worth expecting. The metastatic pancreatic cancer patients treated with the combination of cryoablation and DCs immunotherapy showed significantly higher median survival time than those with cryoablation alone (13 vs. 7 months, p<0.001) (84). Lin et al. evaluated the clinical efficacy of cryoablation combined with allogeneic NK cell immunotherapy in 60 renal cell carcinoma patients. The combination therapy group showed significantly lower CT values of tumor lesion and higher response rate than cryoablation alone group at 3 months post-treatment (85).
RFA and immunotherapy
Preclinical studies indicated that RFA combined with ICIs significantly reduced the tumor burthen and prolong survival time in mice models (86). Further investigation also explored their clinical application. Duffy et al. evaluated Tremelimumab (CTLA-4 inhibitor) in combination with RFA in the patients with advanced hepatocellular carcinoma (87). Definite partial remission was achieved in 5 of 19 patients (26.3%; 95%CI:9.1-51.2%), and favorable PFS and OS estimation. The median viral load was decreased from 1275 x103 IU/ml to 351 x103 IU/ml in 14 patients with quantifiable data. Besides, a metastatic squamous cell lung cancer patient received Atezolizumab (PD-L1 inhibitor) treatment following RFA in left lower lung lesions. The left lung lesion showed a definite therapeutic response, while the right upper lung lesions without RFA treatment showed poor response to Atezolizumab (88). Thus, combined RFA therapy with ICIs maybe an optional regimen when the patient showed poor response to immunotherapy.
The combination of RFA and adoptive cellular immunotherapy also achieved remarkable clinical efficacy. RFA in combination with cell immunotherapy decreased the recurrence risk and disease progression estimation, as compared with RFA alone in HCC patients (recurrence rate: 72% vs. 33%). Notably, 2-year OS rates in the combination treatment and RFA alone group were 100% and 76.6%, respectively (p<0.05) (89). Another clinical study also found that a combination of RFA and RetroNectin activated killer (RAK) cells effectively reduced recurrent the risk of HCC, and no patients suffered recurrence at 7 months follow-up in the 7 HCC patients. The proportion of CD3+/CD8+ cells showed a continuous increase after combinate RFA and RAK treatment (36.08 ± 9.44% to 48.75 ± 9.44%, p=0.03), while the ratio of CD4+/CD8+ decreased (1.14 ± 0.42% to 0.65 ± 0.26%, p=0.02) (90). Increased IFN-γ concentration in peripheral blood was observed in 5 patients (4.73 ± 2.50 pg/ml~7.64 ± 1.10 pg/mL, p=0.02). Zhao et al. followed-up HCC patients who received CIK combined with RFA after transcatheter arterial chemoembolization (TACE). The combination treatment group showed lower recurrence rate than control group (12% vs. 25.8%). Meanwhile, most patients showed decreased HBV DNA content after combined treatment, whereas only one patient decreased from 1.1×105 to less than 103 in the control group (91). Thus, RFA combined with CIK therapy or TACE provides an option to reduce recurrence rate of HCC and anti-HBV effect.
MWA and immunotherapy
ICIs are still the most common option for combination treatment with MWA. A report evaluated MWA combined with Tremelimumab (CTLA-4 inhibitor) treatment in 16 refractory biliary tract cancer patients. Two patients achieved partial remission (12.5%), 5 stable disease (31.3%), and a maximum duration of 6.2 months (92). Notably, the median PFS was 3.4 months in this study, which was higher than second-line chemotherapy as previous report (2.8 months) (93). Another study of Camrelizumab (PD-1 inhibitor) with MWA for non-small cell lung cancer also achieved an ORR of 33.3% and a DCR of 61.9% (94).
The combination of adoptive cell therapy with MWA also demonstrates efficacy and safety in tumor treatment. A phase I clinical study evaluated the safety of percutaneous MWA combined with adoptive cell immunotherapy (DCs and CTLs) in HCC patients. CIK injection was performed at 5 days after MWA, DCs and CTL at 11 days after MWA. No class III/IV serious AEs was observed during the study. Decreased percentage of CD4+CD25high Treg cells was observed after 1 month of combination therapy (2.40 ± 0.61 to 1.67 ± 0.48, p<0.05) and CD8+CD28- effector cells increased significantly (11.41 ± 6.63 to 15.13 ± 7.16, p<0.05) (95).
Staphylococcal enterotoxin C (SEC) exhibits superantigen activity to stimulate the proliferation of peripheral blood monocytes (PBMCs) and release of cytokines, such as IL-2, IFN-γ and TNF-α in a dose-dependent manner (96). Previous study indicated that SEC1 promoted the differentiation of CD4+ and CD8+ T cells to inhibit tumor growth in mice models (97). So combinate SEC with ablation is speculated to enhance antitumor effects. A phase II clinical trial evaluated MWA combined SEC intratumoral injection treatment in HCC. Totally 2000 U SEC was administrated in the edge of the tumor area after different days after MWA (24 days, 28 days, 2 months, 5 months and 7 months, respectively). Compared with the MWA treatment alone group, combined treatment group showed prolonged OS estimation (p=0.032), while no serious AEs associated with SEC injection (98).
Challenges for combining ablation and immunotherapy
Accumulated clinical studies support that the combination of ablation and immunotherapy will achieve better clinical outcome than any treatment regimen alone. However, tumor ablation and immunotherapy still exhibit deficiencies and urgent problems to be solved as a new treatment strategy.
Evaluation system for combinate ablation therapy
No unified evaluation system was established to access for the safety and efficacy of different combination strategies, in consideration of different combinations of ablation and immunotherapies. For example, RFA is considered as an effective regimen for HCC lesion less than 3cm in diameter (99). Then various combinations of RFA with anti-CTLA-4, anti-PD-1 mAb agents and DC-cytokine-induced killer (CIK) further improved a variety of survival estimation related parameters. However, we cannot provide evaluation criteria to measure the efficacy of different combination, which limits further exploration of their clinical application value.
National Health and Family Planning Commission Expert Group on tumor ablation technology management of China established an evaluation criterion in 2017, “National restricted medical technology–Expert interpretation: Standardized management and clinical application’s quality control index of tumor ablation technology” (100), which was updated in 2021 and 2022. The evaluation criteria included a variety of operational accuracy and security index and related calculation formula, such as local lesion control after tumor ablation, serious complication rate within 30 days after tumor ablation. However, no evaluation item was provided to calculate tumor immunotherapy-related indicators, even which kinds of immune responses should be included in the efficacy evaluation system. Moreover, a reasonable evaluation system for immunotherapy efficacy should include reasonable evaluation time-point, and the correlation between immune response and clinical efficacy. Ablation-based combine immunotherapy is deficient in evaluation at present, because of a late start, few clinical studies and a lack of corresponding evaluation parameters. It is suggested to add corresponding immunotherapy evaluation parameters on the basis of the evaluation criteria in tumor ablation technology quality control. Perhaps including anti-tumor immune response indicators as other combination therapies into the efficacy evaluation system. In addition, it should be reminded that the safety assessment of ablation combination therapy is also necessary for clinical application, in view of the novel therapeutic effects and adverse reactions in clinical trials. Moreover, standardized technical and management training for the medical operators will be of great value to the combination therapy evaluation.
Exploration in ablation combine strategies
Personalized treatment plan for cancer patient should not be ignored in the research of ablation combinate therapy. The ablation efficiency is affected by tumor size, location, ablation equipment, ablation duration and frequency in clinical practice, while immunotherapy efficiency is also affected by various factors, such as different forms and dosages in administration. Therefore, the influencing factors for ablation combine therapy are more complex, including the optimal timing of immunotherapy, the choice of adjuvant immunotherapy, and application order of ablation and immunotherapy (101). Previous study indicated that ICIs treatment after MWA, PBMCs showed higher T-cell activity than that of pre-MWA treatment (102). Besides, Imiquimod treatment after cryoablation resulted in better tumor clearance (10/10 vs 3/7 tumors; p=0.0147) and overall treatment efficacy (9/10 vs 2/7 relapse-free tumors; p=0.0345) than immunotherapy before cryoablation in basal cell carcinoma according to Georgios Gaitanis et al. (103). The advanced HCC patients received intravenous NK cell therapy after cryoablation also achieved therapeutic effects (104). These results support immunotherapy after cryoablation exhibits superiority in eliminating tumors and reducing recurrence. However, more clinical trials are needed to explore the specific time and regimen of immunotherapy administration. It is necessary to continuously optimize and explore combined treatment strategies and develop accurate treatment plans.
Tumor tissue destruction caused by cryoablation depends on longer freezing time and slow thawing (105), with a maximum of 15 minutes for freezing and five minutes for thawing each cycle (106). The triple freeze-thaw protocol shows remarkable advantage in time consuming and tumor ablation zones than double freeze-thaw protocol in pulmonary ablation (107). In a study of cryoablation combinate with DC-CIK, the patients with recurrent or advanced HCC received repeated cryoablation showed longer median OS than those received single treatment (106). Treatment frequency is suggested to be an important factor for combination therapy efficiency.
Researchers also attempted to add chemotherapy and targeted therapy to the ablation combine therapy for further efficacy. Aquaporin (AQP) mediates adaptation effects associated with freezing injury, which was supported by increased AQP3 expression in tumor cells at 2 hours after freezing (108). AQP3 participates in the plasma membrane permeability and intracellular water retention, which increases the critical freezing temperature to maintain cell survival advantage with cryoablation treatment. AQP inhibitors (mercuric chloride) combinate with cryoablation significantly reverses resistance and improve treatment effects (109, 110), which supported AQP inhibitors in combination with cryoablation-immunotherapy in clinical practice. Combined cryoablation with Toripalimab (anti-PD-1) and Lenvatinib (anti-angiogenic agent) in a metastatic HCC patient also achieved CR at 7 months after treatment, and PFS as 24 months (111). Compared with cryoablation-chemotherapy and cryoablation-DC-CIK cell therapy, combinate treatment of cryoablation-immunotherapy-chemotherapy showed the longest median OS estimation in metastatic NSCLC (27 months, 95%CI, 26.6-37.0, P <0.001) (112). The combination of cryoablation, NK cell therapy and Herceptin significantly prolonged the PFS of patients with recurrent HER-2 positive breast cancer (113). Moreover, most reports of ablation combine with immunotherapy focus on cryoablation, while few studies choose MWA and RFA. Further clinical trials of MWA and RFA combination therapy are still needed to develop more accurate and efficient treatment plan.
Adverse events in ablation combine therapy and treatment
Ablation related complications and side effects still bring troubles to clinical application despite of its relatively mild and low incidence rate. Among them, cryoshock (114), immunosuppression and thrombocytopenia (115) are observed in cryoablation. Cryoshock is a cytokine release syndrome with a mortality rate of 40% (114), which was corelated with dysfunction of suppressor T cells (116). Cryoshock usually occurs in the patients received cryoablation with a large tumor volume or simultaneous treatment in multiple areas (117). However, the mechanism of cryoablation induced immunosuppressive response is still unclear. Some researchers suggest its relation with cytokines release of TNF-α, IL-1 and IL-6, as well as regulatory T cell proliferation (118). Thrombocytopenia is associated with double cryo-cycle therapy (119). Otto Kollmar et al. demonstrated that aprotinin prevented platelet capture induced by cryoablation, prolonged platelet survival time (3.3 ± 0.4 vs 2.4 ± 0.2 days), and significantly reduced platelet aggregation (local platelet activity: 14.0 ± 1.7% vs 1.9 ± 1.9%; P < 0.001) (120). Moreover, cryoablation may cause skin necrosis, fracture and other complications in the treatment of bone tumors (121). Clark Chen et al. irrigated the adjacent tissues of the lesion with warm saline and filled the defect after resection with bone graft substitute, which could reduce the complication rate from 25% to 2.34% (122). Therefore, improving the accuracy of cryoablation, reducing the range of cryoablation, selecting appropriate ablation points, adjusting the parameter design of freeze-thaw cycle and combining other adjuvant therapies may be effective strategies to reduce adverse reactions of ablation and improve the safety of combined immunotherapy.
Excessive RFA and ICIs are associated with impaired liver function and immune-related adverse events (IrAEs) in liver, which will cause a variety of adverse reactions, such as fever and fatigue (123, 124). High dose corticosteroids are used to alleviate the adverse reactions in clinical practice (125), which will also decrease immune response to influence the curative effect. It is suggested that short-term corticosteroid therapy may reduce the associated adverse effects during ablation combined with immunotherapy. Inadequate RFA (iRFA) is an inevitable defect in RFA treatment alone group, which will facilize tumor proliferation, migration, invasion, epithelial-mesenchymal transformation and angiogenesis by affecting the transcriptional and epigenetic regulation of residual tumor cells, ultimately leading to tumor recurrence and metastasis (126, 127). It is speculated that iRFA may be an important factor in affecting the efficacy of RFA combined immunotherapy. Previous studies have shown that XL888 (HSP90 inhibitor) combined with RFA significantly reduces STAT3 expression and phosphorylation in tumor cells, promotes tumor cell apoptosis, and ameliorates adverse effects of iRFA effectively (128). In addition, RFA may induce arterioportal fistula in the treatment of HCC cases, which limited the therapeutic outcome (129). Xu et al. embolized the fistula between the right hepatic artery and the right portal vein with transcatheter arterial embolization (TAE) in HCC patients. AFP (alpha fetoprotein) remained at normal levels within 3 months and intrahepatic bleeding area was reduced (130), which supported that TAE can be used as a complementary therapy in ablation combine strategies.
Previous studies have shown that thermal ablation induced DAMPs release activates tumor proliferation and development related signaling pathways in the periphery of ablation site (131, 132), which will increase the recurrence risk. Erik Velez et al. selected IL-6, vascular endothelial growth factor (VEGF), and hepatocyte growth factor (HGF) to assess the recurrence risk after ablation. VEGF levels were significantly reduced in 20-W MWA (5-W MWA: 5952 ± 1068 pg/mL, 20-W MWA: 3915 ± 881 pg/mL, p<0.05) (133). Therefore, high power and short term MWA treatment will contribute to a reduced recurrence risk. Optimizing the parameters of MWA will be a new way to reduce the occurrence of adverse reactions in ablation combination therapy.
Summary and outlook
As a new local minimally invasive, safe and effective treatment method, tumor ablation technology has become a reasonable choice in the treatment of solid tumors. Ablation therapy eliminates tumor cells with physical and chemical effects. Released tumor antigen enhances anti-tumor immune response for further therapeutic effect. However, the immune response is generally non-durable. Combining ablation with immunotherapy exhibits profound synergistic effect for the treatment of tumors. In this paper, the effects of cryoablation, RFA and MWA on the immune system were thoroughly summarized. The clinical application of ablation and common immunotherapy strategy was expatiated systematically, including ICIs, small molecule immunodrugs, and adoptive cellular immunotherapy etc. (Figure 2).
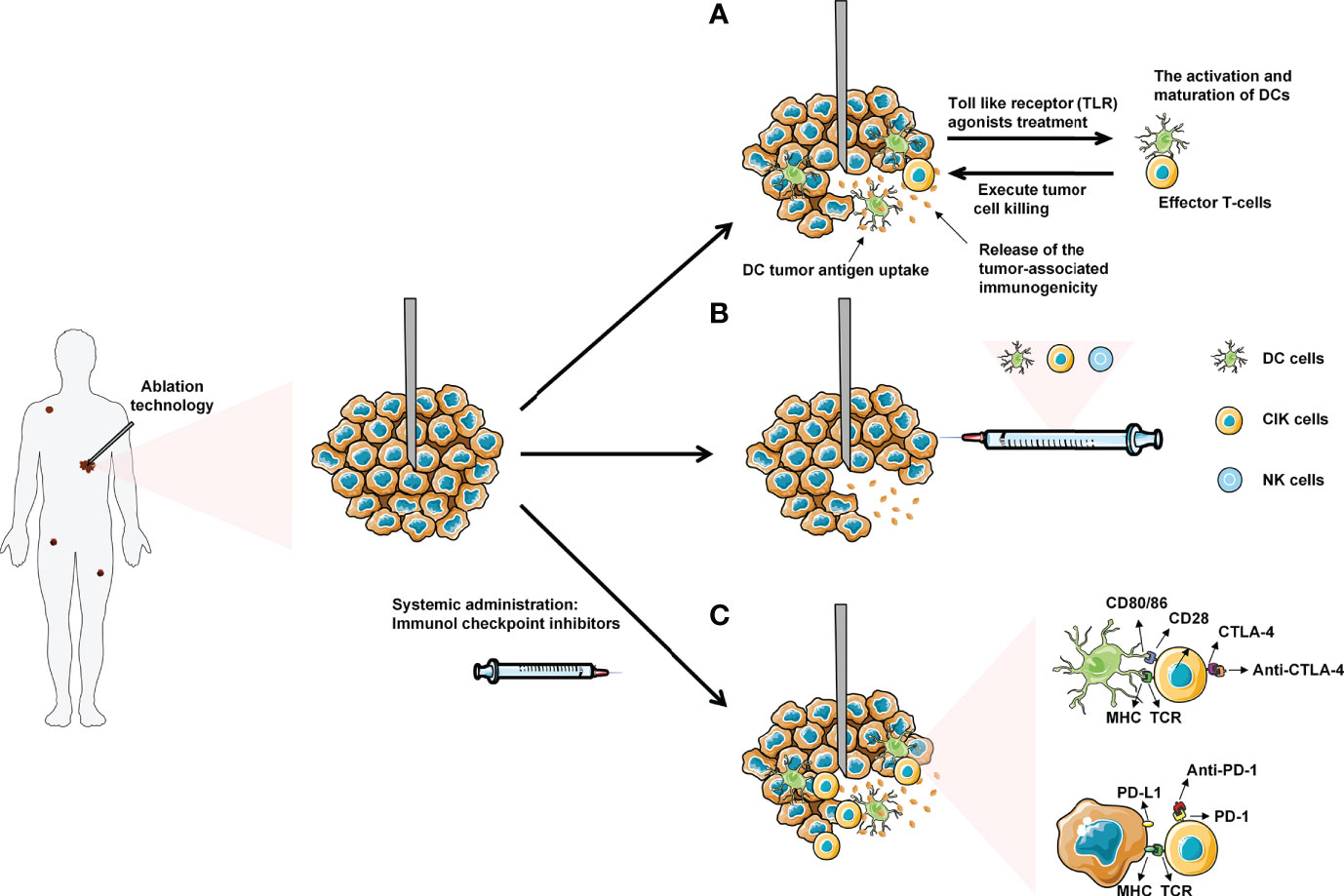
Figure 2 Ablation combined with systemic immunotherapies: (A) Administration of low dose Toll-like receptor (TLR) agonists will cause the activation and maturation of DCs. Ablation induces tumor necrosis and the release of tumor antigens into surrounding tumor microenvironment (TME), which are uptake by mature DCs in TME. The DCs present tumor antigens to naive T-cells, thereby enhancing activation and differentiation into effector T-cells to kill tumor cells. (B) Combined ablation with different immune cells for anti-tumor therapy. (C) Introduction of immune checkpoint inhibitors (anti-CTLA-4 and anti-PD-1/PDL-1) will allow the T-cells to execute tumor cell without being inhibited by the checkpoint signaling. Eventually, the effector T-cells with blocked checkpoint molecules will also migrate to the distant metastasized tumor sites, leading to the regression of metastases.
The safety and efficacy of ablation combined immunotherapy are gradually recognized with the accumulation of relevant clinical studies. However, only a few researchers focus on the standardization of the combination regimens, resulting in the lack of a systematic analysis of literature data for therapeutic evaluation, which limits its clinical application. Therefore, it is necessary to establish a long-term evaluation system to evaluate the safety and efficacy of different combination strategies.
The combination of ablation and immunotherapy is a milestone in the history of cancer treatment. However, it is necessary to develop personalized treatment plan of combination therapy according to clinical experiences and best evidences. We should invest basic research heavily to ablation combination therapy for improved efficacy.
Author contributions
LY and QW drafted the original manuscript. X-YL, L-LZ, G-LC, ZX, Q-QW and J-WB helped to revise the manuscript. LY and QW were responsible for leading this work and revising the manuscript. All authors listed have made a substantial, direct, and intellectual contribution to the work and approved it for publication. All authors have read and approved the final manuscript.
Funding
This research was supported by grants from National Natural Science Foundation of China (81972793, 81803400, 81502283), Natural Science Foundation of Shandong province (ZR2020MH226, ZR2018BH045), Shandong province Medical Health Science and Technology Project (2018WS447), and Qingdao Outstanding Health Professional Development Fund.
Acknowledgments
We apologize to those authors whose deserving research was not cited in this manuscript.
Conflict of interest
The authors declare that the research was conducted in the absence of any commercial or financial relationships that could be construed as a potential conflict of interest.
The reviewer XY declared a shared parent affiliation with the authors LY, LZ, GC, ZX, QW, JB to the handling editor at the time of review.
Publisher’s note
All claims expressed in this article are solely those of the authors and do not necessarily represent those of their affiliated organizations, or those of the publisher, the editors and the reviewers. Any product that may be evaluated in this article, or claim that may be made by its manufacturer, is not guaranteed or endorsed by the publisher.
References
1. Chen W, Zheng R, Baade PD, Zhang S, Zeng H, Bray F, et al. Cancer statistics in China, 2015. CA Cancer J Clin (2016) 66(2):115–32. doi: 10.3322/caac.21338
2. Baskar R, Lee KA, Yeo R, Yeoh KW. Cancer and radiation therapy: current advances and future directions. Int J Med Sci (2012) 9(3):193–9. doi: 10.7150/ijms.3635
3. Datta M, Coussens LM, Nishikawa H, Hodi FS, Jain RK. Reprogramming the tumor microenvironment to improve immunotherapy: Emerging strategies and combination therapies. Am Soc Clin Oncol Educ Book (2019) 39:165–74. doi: 10.1200/EDBK_237987
4. Mooradian MJ, Sullivan RJ. Immunomodulatory effects of current cancer treatment and the consequences for follow-up immunotherapeutics. Future Oncol (2017) 13(18):1649–63. doi: 10.2217/fon-2017-0117
5. Elias R, Hartshorn K, Rahma O, Lin N, Snyder-Cappione JE. Aging,immune senescence,and immunotherapy:A comprehensive review. Semin Oncol (2018) 45(4):187–200. doi: 10.1053/j.seminoncol.2018.08.006
6. Luo W, Zhang Y, He G, Yu M, Zheng M, Liu L, et al. Effects of radiofrequency ablation versus other ablating techniques on hepatocellular carcinomas: a systematic review and meta-analysis. World J Surg Oncol (2017) 15(1):126. doi: 10.1186/s12957-017-1196-2
7. van den Bijgaart RJ, Eikelenboom DC, Hoogenboom M, Futterer JJ, den Brok MH, Adema GJ. Thermal and mechanical high-intensity focused ultrasound: perspectives on tumor ablation, immune effects and combination strategies. Cancer Immunol Immunother (2017) 66(2):247–58. doi: 10.1007/s00262-016-1891-9
8. Gandhi NS, Dupuy DE. Image-guided radiofrequency ablation as a new treatment option for patients with lung cancer. Semin Roentgenol (2005) 40(2):171–81. doi: 10.1053/j.ro.2005.01.009
9. Liang P, Wang Y. Microwave ablation of hepatocellular carcinoma. Oncology(2007) (72) Suppl1:124–31. doi: 10.1159/000111718
10. Hoffmann R, Rempp H, Schmidt D, Pereira PL, Claussen CD, Clasen S. Prolonged antibiotic prophylaxis in patients with bilioenteric anastomosis undergoing percutaneous radiofrequency ablation. J Vasc Interv Radiol (2012) 23(4):545–51. doi: 10.1016/j.jvir.2011.12.025
11. Knull E, Oto A, Eggener S, Tessier D, Guneyli S, Chatterjee A, et al. Evaluation of tumor coverage after MR-guided prostate focal laser ablation therapy. Med Phys (2019) 46(2):800–10. doi: 10.1002/mp.13292
12. Sebek J, Taeprasartsit P, Wibowo H, Beard WL, Bortel R, Prakash P. Microwave ablation of lung tumors: A probabilistic approach for simulation-based treatment planning. Med Phys (2021) 48(7):3991–4003. doi: 10.1002/mp.14923
13. Alexander ES, Xiong L, Baird GL, Fernando H, Dupuy DE. CT densitometry and morphology of radiofrequency-ablated stage IA non-small cell lung cancer: Results from the American college of surgeons oncology group Z4033 (Alliance) trial. J Vasc Interv Radiol (2020) 31(2):286–93. doi: 10.1016/j.jvir.2019.09.010
14. Mala T, Samset E, Aurdal L, Gladhaug I, Edwin B, Soreide O. Magnetic resonance imaging-estimated three-dimensional temperature distribution in liver cryolesions: a study of cryolesion characteristics assumed necessary for tumor ablation. Cryobiology (2001) 43(3):268–75. doi: 10.1006/cryo.2001.2351
15. Wu F. Heat-based tumor ablation: Role of the immune response. Adv Exp Med Biol (2016) 880:131–53. doi: 10.1007/978-3-319-22536-4_8
16. Abtin F, De Baere T, Dupuy DE, Genshaft S, Healey T, Khan S, et al. Updates on current role and practice of lung ablation. J Thorac Imaging (2019) 34(4):266–77. doi: 10.1097/RTI.0000000000000417
17. Abtin F, Suh RD, Nasehi L, Han SX, Hsu W, Quirk M, et al. Percutaneous cryoablation for the treatment of recurrent thymoma: preliminary safety and efficacy. J Vasc Interv Radiol (2015) 26(5):709–14. doi: 10.1016/j.jvir.2014.12.024
18. Mazur P. Freezing of living cells: mechanisms and implications. Am J Physiol (1984) 247(3 Pt 1):C125–42. doi: 10.1152/ajpcell.1984.247.3.C125
19. Mahnken AH, Konig AM, Figiel JH. Current technique and application of percutaneous cryotherapy. Rofo (2018) 190(9):836–46. doi: 10.1055/a-0598-5134
20. Hu KQ. Advances in clinical application of cryoablation therapy for hepatocellular carcinoma and metastatic liver tumor. J Clin Gastroenterol (2014) 48(10):830–6. doi: 10.1097/MCG.0000000000000201
21. Callstrom MR, Woodrum DA, Nichols FC, Palussiere J, Buy X, Suh RD, et al. Multicenter study of metastatic lung tumors targeted by interventional cryoablation evaluation (SOLSTICE). J Thorac Oncol (2020) 15(7):1200–9. doi: 10.1016/j.jtho.2020.02.022
22. Whitworth PW, Rewcastle JC. Cryoablation and cryolocalization in the management of breast disease. J Surg Oncol (2005) 90(1):1–9. doi: 10.1002/jso.20201
23. Callstrom MR, Dupuy DE, Solomon SB, Beres RA, Littrup PJ, Davis KW, et al. Percutaneous image-guided cryoablation of painful metastases involving bone: multicenter trial. Cancer (2013) 119(5):1033–41. doi: 10.1002/cncr.27793
24. Maiwand MO. The role of cryosurgery in palliation of tracheo-bronchial carcinoma. Eur J Cardiothorac Surg (1999) 15(6):764–8. doi: 10.1016/s1010-7940(99)00121-9
25. Alzubaidi SJ, Liou H, Saini G, Segaran N, Scott Kriegshauser J, Naidu SG, et al. Percutaneous image-guided ablation of lung tumors. J Clin Med (2021) 10(24):5783. doi: 10.3390/jcm10245783
26. Ambrogi MC, Fanucchi O, Cioni R, Dini P, De Liperi A, Cappelli C, et al. Long-term results of radiofrequency ablation treatment of stage I non-small cell lung cancer: a prospective intention-to-treat study. J Thorac Oncol (2011) 6(12):2044–51. doi: 10.1097/JTO.0b013e31822d538d
27. Yamauchi Y, Izumi Y, Hashimoto K, Yashiro H, Inoue M, Nakatsuka S, et al. Percutaneous cryoablation for the treatment of medically inoperable stage I non-small cell lung cancer. PloS One (2012) 7(3):e33223. doi: 10.1371/journal.pone.0033223
28. Cao C, Tian D, Manganas C, Matthews P, Yan TD. Systematic review of trimodality therapy for patients with malignant pleural mesothelioma. Ann Cardiothorac Surg (2012) 1(4):428–37. doi: 10.3978/j.issn.2225-319X.2012.11.07
29. Izzo F, Granata V, Grassi R, Fusco R, Palaia R, Delrio P, et al. Radiofrequency ablation and microwave ablation in liver tumors: An update. Oncologist (2019) 24(10):e990–e1005. doi: 10.1634/theoncologist.2018-0337
30. Mehta A, Oklu R, Sheth RA. Thermal ablative therapies and immune checkpoint modulation: Can locoregional approaches effect a systemic response? Gastroenterol Res Pract(2016) (2016):9251375. doi: 10.1155/2016/9251375
31. Vogl TJ, Farshid P, Naguib NN, Darvishi A, Bazrafshan B, Mbalisike E, et al. Thermal ablation of liver metastases from colorectal cancer: radiofrequency, microwave and laser ablation therapies. Radiol Med (2014) 119(7):451–61. doi: 10.1007/s11547-014-0415-y
32. Frich L. [Local ablation of colorectal liver metastases–a systematic review]. Tidsskr Nor Laegeforen (2008) 128(1):54–6.
33. Kan X, Wang Y, Han P, Yao Q, Qian K, Xiong B, et al. Combined ultrasound/computed tomography guidance in percutaneous radiofrequency ablation after transarterial chemoembolization for hepatocellular carcinoma in the hepatic dome. Cancer Manag Res (2019) 11:7751–7. doi: 10.2147/CMAR.S212127
34. Lai C, Jin RA, Liang X, Cai XJ. Comparison of laparoscopic hepatectomy, percutaneous radiofrequency ablation and open hepatectomy in the treatment of small hepatocellular carcinoma. J Zhejiang Univ Sci B (2016) 17(3):236–46. doi: 10.1631/jzus.B1500322
35. Wong J, Lee KF, Yu SC, Lee PS, Cheung YS, Chong CN, et al. Percutaneous radiofrequency ablation versus surgical radiofrequency ablation for malignant liver tumours: the long-term results. HPB (Oxford) (2013) 15(8):595–601. doi: 10.1111/hpb.12014
36. Ng KK, Poon RT, Lam CM, Yuen J, Tso WK, Fan ST. Efficacy and safety of radiofrequency ablation for perivascular hepatocellular carcinoma without hepatic inflow occlusion. Br J Surg (2006) 93(4):440–7. doi: 10.1002/bjs.5267
37. Wong J, Lee KF, Lee PS, Ho SS, Yu SC, Ng WW, et al. Radiofrequency ablation for 110 malignant liver tumours: preliminary results on percutaneous and surgical approaches. Asian J Surg (2009) 32(1):13–20. doi: 10.1016/S1015-9584(09)60003-8
38. Weis S, Franke A, Mossner J, Jakobsen JC, Schoppmeyer K. Radiofrequency (thermal) ablation versus no intervention or other interventions for hepatocellular carcinoma. Cochrane Database Syst Rev (2013) 12):CD003046. doi: 10.1002/14651858.CD003046.pub3
39. Spiliotis AE, Gabelein G, Hollander S, Scherber PR, Glanemann M, Patel B. Microwave ablation compared with radiofrequency ablation for the treatment of liver cancer: a systematic review and meta-analysis. Radiol Oncol (2021) 55(3):247–58. doi: 10.2478/raon-2021-0030
40. Kuang M, Lu MD, Xie XY, Xu HX, Mo LQ, Liu GJ, et al. Liver cancer: increased microwave delivery to ablation zone with cooled-shaft antenna–experimental and clinical studies. Radiology (2007) 242(3):914–24. doi: 10.1148/radiol.2423052028
41. Ni Y, Ye X, Wan C, Ni Q, Yang X, Huang G, et al. Percutaneous microwave ablation (MWA) increased the serum levels of VEGF and MMP-9 in stage I non-small cell lung cancer (NSCLC). Int J Hyperthermia (2017) 33(4):435–9. doi: 10.1080/02656736.2017.1284350
42. Hou Q, Yu X, Cheng Z, Han Z, Liu F, Dou J, et al. Acute kidney injury after nephron sparing surgery and microwave ablation: focus on incidence, survival impact and prediction. Int J Hyperthermia (2020) 37(1):470–8. doi: 10.1080/02656736.2020.1752944
43. Espinosa De Ycaza AE, Welch TL, Ospina NS, Rodriguez-Gutierrez R, Atwell TD, Erickson D, et al. Image-guided thermal ablation of adrenal metastases: Hemodynamic and endocrine outcomes. Endocr Pract (2017) 23(2):132–40. doi: 10.4158/EP161498.OR
44. Beermann M, Delle M, Magnusson M, Casswall T. [Microwave ablation of the spleen: An easy and gentle method for treatment of secondary splenomegaly in children. a case report]. Lakartidningen (2021) 118:21093.
45. Lu C, Li X, Chu X, Li R, Li J, Wang J, et al. Clinical effects of microwave ablation in the treatment of low-risk papillary thyroid microcarcinomas and related histopathological changes. Front Endocrinol (Lausanne) (2021) 12:751213. doi: 10.3389/fendo.2021.751213
46. Xu J, Wu H, Han Z, Zhang J, Li Q, Dou J, et al. Microwave ablation of benign breast tumors: a prospective study with minimum 12 months follow-up. Int J Hyperthermia (2018) 35(1):253–61. doi: 10.1080/02656736.2018.1494340
47. Simo KA, Tsirline VB, Sindram D, McMillan MT, Thompson KJ, Swan RZ, et al. Microwave ablation using 915-MHz and 2.45-GHz systems: what are the differences? HPB (Oxford) (2013) 15(12):991–6. doi: 10.1111/hpb.12081
48. Qin S, Liu GJ, Huang M, Huang J, Luo Y, Wen Y, et al. The local efficacy and influencing factors of ultrasound-guided percutaneous microwave ablation in colorectal liver metastases: a review of a 4-year experience at a single center. Int J Hyperthermia (2019) 36(1):36–43. doi: 10.1080/02656736.2018.1528511
49. Kovacs A, Bischoff P, Haddad H, Zhou W, Temming S, Schafer A, et al. Long-term comparative study on the local tumour control of different ablation technologies in primary and secondary liver malignancies. J Pers Med (2022) 12(3):430. doi: 10.3390/jpm12030430
50. Medhat E, Abdel Aziz A, Nabeel M, Elbaz T, Zakaria Z, Shousha H, et al. Value of microwave ablation in treatment of large lesions of hepatocellular carcinoma. J Dig Dis (2015) 16(8):456–63. doi: 10.1111/1751-2980.12259
51. Ahmad F, Gravante G, Bhardwaj N, Strickland A, Basit R, West K, et al. Renal effects of microwave ablation compared with radiofrequency, cryotherapy and surgical resection at different volumes of the liver treated. Liver Int (2010) 30(9):1305–14. doi: 10.1111/j.1478-3231.2010.02290.x
52. Yakkala C, Chiang CL, Kandalaft L, Denys A, Duran R. Cryoablation and immunotherapy: An enthralling synergy to confront the tumors. Front Immunol (2019) 10:2283. doi: 10.3389/fimmu.2019.02283
53. Waitz R, Solomon SB, Petre EN, Trumble AE, Fasso M, Norton L, et al. Potent induction of tumor immunity by combining tumor cryoablation with anti-CTLA-4 therapy. Cancer Res (2012) 72(2):430–9. doi: 10.1158/0008-5472.CAN-11-1782
54. Zhu C, Lin S, Liang J, Zhu Y. PD-1 blockade enhances the anti-tumor immune response induced by cryoablation in a murine model of renal cell carcinoma. Cryobiology (2019) 87:86–90. doi: 10.1016/j.cryobiol.2019.01.015
55. Baust JG, Snyder KK, Santucci KL, Robilotto AT, Van Buskirk RG, Baust JM. Cryoablation: physical and molecular basis with putative immunological consequences. Int J Hyperthermia (2019) 36(sup1):10–6. doi: 10.1080/02656736.2019.1647355
56. Keisari Y. Tumor abolition and antitumor immunostimulation by physico-chemical tumor ablation. Front Biosci (2017) 22(2):310–47. doi: 10.2741/4487
57. Kato T, Iwasaki T, Uemura M, Nagahara A, Higashihara H, Osuga K, et al. Characterization of the cryoablation-induced immune response in kidney cancer patients. Oncoimmunology (2017) 6(7):e1326441. doi: 10.1080/2162402X.2017.1326441
58. Erinjeri JP, Thomas CT, Samoilia A, Fleisher M, Gonen M, Sofocleous CT, et al. Image-guided thermal ablation of tumors increases the plasma level of interleukin-6 and interleukin-10. J Vasc Interv Radiol (2013) 24(8):1105–12. doi: 10.1016/j.jvir.2013.02.015
59. Zerbini A, Pilli M, Fagnoni F, Pelosi G, Pizzi MG, Schivazappa S, et al. Increased immunostimulatory activity conferred to antigen-presenting cells by exposure to antigen extract from hepatocellular carcinoma after radiofrequency thermal ablation. J Immunother (2008) 31(3):271–82. doi: 10.1097/CJI.0b013e318160ff1c
60. Fietta AM, Morosini M, Passadore I, Cascina A, Draghi P, Dore R, et al. Systemic inflammatory response and downmodulation of peripheral CD25+Foxp3+ T-regulatory cells in patients undergoing radiofrequency thermal ablation for lung cancer. Hum Immunol (2009) 70(7):477–86. doi: 10.1016/j.humimm.2009.03.012
61. Nikfarjam M, Muralidharan V, Christophi C. Mechanisms of focal heat destruction of liver tumors. J Surg Res (2005) 127(2):208–23. doi: 10.1016/j.jss.2005.02.009
62. Bhardwaj N, Dormer J, Ahmad F, Strickland AD, Gravante G, Beckingham I, et al. Heat shock protein 70 expression following hepatic radiofrequency ablation is affected by adjacent vasculature. J Surg Res (2012) 173(2):249–57. doi: 10.1016/j.jss.2010.09.040
63. Schueller G, Kettenbach J, Sedivy R, Bergmeister H, Stift A, Fried J, et al. Expression of heat shock proteins in human hepatocellular carcinoma after radiofrequency ablation in an animal model. Oncol Rep (2004) 12(3):495–9.
64. Haen SP, Gouttefangeas C, Schmidt D, Boss A, Clasen S, von Herbay A, et al. Elevated serum levels of heat shock protein 70 can be detected after radiofrequency ablation. Cell Stress Chaperones (2011) 16(5):495–504. doi: 10.1007/s12192-011-0261-y
65. Rochigneux P, Nault JC, Mallet F, Chretien AS, Barget N, Garcia AJ, et al. Dynamic of systemic immunity and its impact on tumor recurrence after radiofrequency ablation of hepatocellular carcinoma. Oncoimmunology (2019) 8(8):1615818. doi: 10.1080/2162402X.2019.1615818
66. Todorova VK, Klimberg VS, Hennings L, Kieber-Emmons T, Pashov A. Immunomodulatory effects of radiofrequency ablation in a breast cancer model. Immunol Invest (2010) 39(1):74–92. doi: 10.3109/08820130903428291
67. Uzunparmak B, Sahin IH. Pancreatic cancer microenvironment: a current dilemma. Clin Transl Med (2019) 8(1):2. doi: 10.1186/s40169-019-0221-1
68. Widenmeyer M, Shebzukhov Y, Haen SP, Schmidt D, Clasen S, Boss A, et al. Analysis of tumor antigen-specific T cells and antibodies in cancer patients treated with radiofrequency ablation. Int J Cancer (2011) 128(11):2653–62. doi: 10.1002/ijc.25601
69. Chu KF, Dupuy DE. Thermal ablation of tumours: biological mechanisms and advances in therapy. Nat Rev Cancer (2014) 14(3):199–208. doi: 10.1038/nrc3672
70. Gao S, Pu N, Yin H, Li J, Chen Q, Yang M, et al. Radiofrequency ablation in combination with an mTOR inhibitor restrains pancreatic cancer growth induced by intrinsic HSP70. Ther Adv Med Oncol (2020) 12:1758835920953728. doi: 10.1177/1758835920953728
71. Zhou W, Yu M, Pan H, Qiu W, Wang H, Qian M, et al. Microwave ablation induces Th1-type immune response with activation of ICOS pathway in early-stage breast cancer. J Immunother Cancer (2021) 9(4):e002343. doi: 10.1136/jitc-2021-002343
72. Zhang H, Hou X, Cai H, Zhuang X. Effects of microwave ablation on T-cell subsets and cytokines of patients with hepatocellular carcinoma0. Minim Invasive Ther Allied Technol (2017) 26(4):207–11. doi: 10.1080/13645706.2017.1286356
73. Simpson TR, Quezada SA, Allison JP. Regulation of CD4 T cell activation and effector function by inducible costimulator (ICOS). Curr Opin Immunol (2010) 22(3):326–32. doi: 10.1016/j.coi.2010.01.001
74. Emens LA. Breast cancer immunotherapy: Facts and hopes. Clin Cancer Res (2018) 24(3):511–20. doi: 10.1158/1078-0432.CCR-16-3001
75. Bagchi S, Yuan R, Engleman EG. Immune checkpoint inhibitors for the treatment of cancer: Clinical impact and mechanisms of response and resistance. Annu Rev Pathol (2021) 16:223–49. doi: 10.1146/annurev-pathol-042020-042741
76. Hack SP, Spahn J, Chen M, Cheng AL, Kaseb A, Kudo M, et al. IMbrave 050: a phase III trial of atezolizumab plus bevacizumab in high-risk hepatocellular carcinoma after curative resection or ablation. Future Oncol (2020) 16(15):975–89. doi: 10.2217/fon-2020-0162
77. McArthur HL, Diab A, Page DB, Yuan J, Solomon SB, Sacchini V, et al. A pilot study of preoperative single-dose ipilimumab and/or cryoablation in women with early-stage breast cancer with comprehensive immune profiling. Clin Cancer Res (2016) 22(23):5729–37. doi: 10.1158/1078-0432.CCR-16-0190
78. Zhu B, Liu Y, Li J, Diao L, Shao L, Han-Zhang H, et al. Exceptional response of cryoablation followed by pembrolizumab in a patient with metastatic cervical carcinosarcoma with high tumor mutational burden: a case report. Oncologist (2020) 25(1):15–8. doi: 10.1634/theoncologist.2019-0739
79. Shen L, Qi H, Chen S, Cao F, Xie L, Wu Y, et al. Cryoablation combined with transarterial infusion of pembrolizumab (CATAP) for liver metastases of melanoma: an ambispective, proof-of-concept cohort study. Cancer Immunol Immunother (2020) 69(9):1713–24. doi: 10.1007/s00262-020-02566-z
80. Tumeh PC, Hellmann MD, Hamid O, Tsai KK, Loo KL, Gubens MA, et al. Liver metastasis and treatment outcome with anti-PD-1 monoclonal antibody in patients with melanoma and NSCLC. Cancer Immunol Res (2017) 5(5):417–24. doi: 10.1158/2326-6066.CIR-16-0325
81. Robert C, Schachter J, Long GV, Arance A, Grob JJ, Mortier L, et al. Pembrolizumab versus ipilimumab in advanced melanoma. N Engl J Med (2015) 372(26):2521–32. doi: 10.1056/NEJMoa1503093
82. Dowling DJ. Recent advances in the discovery and delivery of TLR7/8 agonists as vaccine adjuvants. Immunohorizons (2018) 2(6):185–97. doi: 10.4049/immunohorizons.1700063
83. Gaitanis G, Nomikos K, Vava E, Alexopoulos EC, Bassukas ID. Immunocryosurgery for basal cell carcinoma: results of a pilot, prospective, open-label study of cryosurgery during continued imiquimod application. J Eur Acad Dermatol Venereol (2009) 23(12):1427–31. doi: 10.1111/j.1468-3083.2009.03224.x
84. Niu L, Chen J, He L, Liao M, Yuan Y, Zeng J, et al. Combination treatment with comprehensive cryoablation and immunotherapy in metastatic pancreatic cancer. Pancreas (2013) 42(7):1143–9. doi: 10.1097/MPA.0b013e3182965dde
85. Lin M, Xu K, Liang S, Wang X, Liang Y, Zhang M, et al. Prospective study of percutaneous cryoablation combined with allogenic NK cell immunotherapy for advanced renal cell cancer. Immunol Lett (2017) 184:98–104. doi: 10.1016/j.imlet.2017.03.004
86. Shi L, Chen L, Wu C, Zhu Y, Xu B, Zheng X, et al. PD-1 blockade boosts radiofrequency ablation-elicited adaptive immune responses against tumor. Clin Cancer Res (2016) 22(5):1173–84. doi: 10.1158/1078-0432.CCR-15-1352
87. Duffy AG, Ulahannan SV, Makorova-Rusher O, Rahma O, Wedemeyer H, Pratt D, et al. Tremelimumab in combination with ablation in patients with advanced hepatocellular carcinoma. J Hepatol (2017) 66(3):545–51. doi: 10.1016/j.jhep.2016.10.029
88. Yin J, Dong J, Gao W, Wang Y. A case report of remarkable response to association of radiofrequency ablation with subsequent atezolizumab in stage IV nonsmall cell lung cancer. Med (Baltimore) (2018) 97(44):e13112. doi: 10.1097/MD.0000000000013112
89. Cui J, Wang N, Zhao H, Jin H, Wang G, Niu C, et al. Combination of radiofrequency ablation and sequential cellular immunotherapy improves progression-free survival for patients with hepatocellular carcinoma. Int J Cancer (2014) 134(2):342–51. doi: 10.1002/ijc.28372
90. Ma H, Zhang Y, Wang Q, Li Y, He J, Wang H, et al. Therapeutic safety and effects of adjuvant autologous RetroNectin activated killer cell immunotherapy for patients with primary hepatocellular carcinoma after radiofrequency ablation. Cancer Biol Ther (2010) 9(11):903–7. doi: 10.4161/cbt.9.11.11697
91. Zhao M, Wu PH, Zeng YX, Xia JC, Zhang FJ, Xian LJ, et al. Cytokine-induced killer cell fusion to lower recurrence of hepatocellular carcinoma after transcatheter arterial chemoembolization sequentially combined with radiofrequency ablation: a randomized trial. Zhonghua Yi Xue Za Zhi (2006) 86(26):1823–8.
92. Xie C, Duffy AG, Mabry-Hrones D, Wood B, Levy E, Krishnasamy V, et al. Tremelimumab in combination with microwave ablation in patients with refractory biliary tract cancer. Hepatology (2019) 69(5):2048–60. doi: 10.1002/hep.30482
93. Walter T, Horgan AM, McNamara M, McKeever L, Min T, Hedley D, et al. Feasibility and benefits of second-line chemotherapy in advanced biliary tract cancer: a large retrospective study. Eur J Cancer (2013) 49(2):329–35. doi: 10.1016/j.ejca.2012.08.003
94. Wei Z, Yang X, Ye X, Huang G, Li W, Han X, et al. Camrelizumab combined with microwave ablation improves the objective response rate in advanced non-small cell lung cancer. J Cancer Res Ther (2019) 15(7):1629–34. doi: 10.4103/jcrt.JCRT_990_19
95. Zhou P, Liang P, Dong B, Yu X, Han Z, Xu Y. Phase clinical study of combination therapy with microwave ablation and cellular immunotherapy in hepatocellular carcinoma. Cancer Biol Ther (2011) 11(5):450–6. doi: 10.4161/cbt.11.5.14669
96. Liu T, Li L, Yin L, Yu H, Jing H, Liu Y, et al. Superantigen staphylococcal enterotoxin C1 inhibits the growth of bladder cancer. Biosci Biotechnol Biochem (2017) 81(9):1741–6. doi: 10.1080/09168451.2017.1350564
97. Jie KG, Jiang H, Sun L, Wang HR, Zheng YL, Li Y, et al. The pilot study of anti-tumor effects versus immunosuppression of staphylococcal enterotoxin c. Cancer Biol Ther (2007) 6(10):1584–91. doi: 10.4161/cbt.6.10.4724
98. Zhou P, Liang P, Dong B, Yu X, Han X, Wang Y, et al. Long-term results of a phase II clinical trial of superantigen therapy with staphylococcal enterotoxin c after microwave ablation in hepatocellular carcinoma. Int J Hyperthermia (2011) 27(2):132–9. doi: 10.3109/02656736.2010.506670
99. Xu Z, Xie H, Zhou L, Chen X, Zheng S. The combination strategy of transarterial chemoembolization and radiofrequency ablation or microwave ablation against hepatocellular carcinoma. Anal Cell Pathol (Amst) (2019) 2019:8619096. doi: 10.1155/2019/8619096
100. Fan WJ, Han Y. [National restricted medical technology–expert interpretation: Standardized management and clinical application's quality control index of tumor ablation technology]. Zhonghua Yi Xue Za Zhi (2017) 97(31):2404–6. doi: 10.3760/cma.j.issn.0376-2491.2017.31.002
101. Wang K, Wang C, Jiang H, Zhang Y, Lin W, Mo J, et al. Combination of ablation and immunotherapy for hepatocellular carcinoma: Where we are and where to go. Front Immunol (2021) 12:792781. doi: 10.3389/fimmu.2021.792781
102. Zhou W, Yu M, Mao X, Pan H, Tang X, Wang J, et al. Landscape of the peripheral immune response induced by local microwave ablation in patients with breast cancer. Adv Sci (2022) 9(17):e2200033. doi: 10.1002/advs.202200033
103. Gaitanis G, Alexopoulos EC, Bassukas ID. Cryosurgery is more effective in the treatment of primary, non-superficial basal cell carcinomas when applied during and not prior to a five week imiquimod course: a randomized, prospective, open-label study. Eur J Dermatol (2011) 21(6):952–8. doi: 10.1684/ejd.2011.1524
104. Lin M, Liang S, Wang X, Liang Y, Zhang M, Chen J, et al. Cryoablation combined with allogenic natural killer cell immunotherapy improves the curative effect in patients with advanced hepatocellular cancer. Oncotarget (2017) 8(47):81967–77. doi: 10.18632/oncotarget.17804
105. Gage AA, Guest K, Montes M, Caruana JA, Whalen DA Jr. Effect of varying freezing and thawing rates in experimental cryosurgery. Cryobiology (1985) 22(2):175–82. doi: 10.1016/0011-2240(85)90172-5
106. Niu LZ, Li JL, Zeng JY, Mu F, Liao MT, Yao F, et al. Combination treatment with comprehensive cryoablation and immunotherapy in metastatic hepatocellular cancer. World J Gastroenterol (2013) 19(22):3473–80. doi: 10.3748/wjg.v19.i22.3473
107. Hinshaw JL, Littrup PJ, Durick N, Leung W, Lee FT Jr., Sampson L, et al. Optimizing the protocol for pulmonary cryoablation: a comparison of a dual- and triple-freeze protocol. Cardiovasc Intervent Radiol (2010) 33(6):1180–5. doi: 10.1007/s00270-010-9868-0
108. Ismail M, Bokaee S, Morgan R, Davies J, Harrington KJ, Pandha H. Inhibition of the aquaporin 3 water channel increases the sensitivity of prostate cancer cells to cryotherapy. Br J Cancer (2009) 100(12):1889–95. doi: 10.1038/sj.bjc.6605093
109. Alkhalifa H, Mohammed F, Taurin S, Greish K, Taha S, Fredericks S. Inhibition of aquaporins as a potential adjunct to breast cancer cryotherapy. Oncol Lett (2021) 21(6):458. doi: 10.3892/ol.2021.12719
110. Tomita Y, Dorward H, Yool AJ, Smith E, Townsend AR, Price TJ, et al. Role of aquaporin 1 signalling in cancer development and progression. Int J Mol Sci (2017) 18(2):299. doi: 10.3390/ijms18020299
111. Li X, Xu J, Gu X, Chen L, Wu Q, Li H, et al. Case report: Antiangiogenic therapy plus immune checkpoint inhibitors combined with intratumoral cryoablation for hepatocellular carcinoma. Front Immunol (2021) 12:740790. doi: 10.3389/fimmu.2021.740790
112. Yuanying Y, Lizhi N, Feng M, Xiaohua W, Jianying Z, Fei Y, et al. Therapeutic outcomes of combining cryotherapy, chemotherapy and DC-CIK immunotherapy in the treatment of metastatic non-small cell lung cancer. Cryobiology (2013) 67(2):235–40. doi: 10.1016/j.cryobiol.2013.08.001
113. Liang S, Niu L, Xu K, Wang X, Liang Y, Zhang M, et al. Tumor cryoablation in combination with natural killer cells therapy and herceptin in patients with HER2-overexpressing recurrent breast cancer. Mol Immunol (2017) 92:45–53. doi: 10.1016/j.molimm.2017.10.003
114. Ni Eochagain A. Cryoshock following cryoablation for hepatocellular carcinoma. J Clin Anesth (2022) 77:110641. doi: 10.1016/j.jclinane.2021.110641
115. Shi J, Niu L, Huang Z, Mu F, Chen J, Li J, et al. Diagnosis and treatment of coagulopathy following percutaneous cryoablation of liver tumors: experience in 372 patients. Cryobiology (2013) 67(2):146–50. doi: 10.1016/j.cryobiol.2013.06.004
116. Shibata T, Suzuki K, Yamashita T, Takeichi N, Mark M, Hosokawa M, et al. Immunological analysis of enhanced spontaneous metastasis in WKA rats following cryosurgery. Anticancer Res (1998) 18(4A):2483–6.
117. Haen SP, Pereira PL, Salih HR, Rammensee HG, Gouttefangeas C. More than just tumor destruction: immunomodulation by thermal ablation of cancer. Clin Dev Immunol (2011) 2011:160250. doi: 10.1155/2011/160250
118. Pistorius GA, Alexander C, Krisch CM, Feifel G, Schilling MK, Menger MD. Local platelet trapping as the cause of thrombocytopenia after hepatic cryotherapy. World J Surg (2005) 29(5):657–60. doi: 10.1007/s00268-005-7543-4
119. Ferreira CA, Vicente WV, Evora PR, Rodrigues AJ, Klamt JG, Carlotti AP, et al. Does aprotinin preserve platelets in children with acyanogenic congenital heart disease undergone surgery with cardiopulmonary bypass? Rev Bras Cir Cardiovasc (2009) 24(3):373–81. doi: 10.1590/s0102-76382009000400018
120. Kollmar O, Richter S, Czyborra J, Menger MD, Dietrich S, Schilling MK, et al. Aprotinin inhibits local platelet trapping and improves tissue destruction in hepatic cryosurgery. Surgery (2004) 136(3):624–32. doi: 10.1016/j.surg.2004.02.004
121. Marcove RC, Weis LD, Vaghaiwalla MR, Pearson R, Huvos AG. Cryosurgery in the treatment of giant cell tumors of bone. a report of 52 consecutive cases. Cancer (1978) 41(3):957–69. doi: 10.1002/1097-0142(197803)41:3<957::aid-cncr2820410325>3.0.co;2-y
122. Chen C, Garlich J, Vincent K, Brien E. Postoperative complications with cryotherapy in bone tumors. J Bone Oncol (2017) 7:13–7. doi: 10.1016/j.jbo.2017.04.002
123. Fonseca AZ, Santin S, Gomes LG, Waisberg J, Ribeiro MA Jr. Complications of radiofrequency ablation of hepatic tumors: Frequency and risk factors. World J Hepatol (2014) 6(3):107–13. doi: 10.4254/wjh.v6.i3.107
124. Dougan M, Blidner AG, Choi J, Cooksley T, Glezerman I, Ginex P, et al. Multinational association of supportive care in cancer (MASCC) 2020 clinical practice recommendations for the management of severe gastrointestinal and hepatic toxicities from checkpoint inhibitors. Support Care Cancer (2020) 28(12):6129–43. doi: 10.1007/s00520-020-05707-3
125. Riveiro-Barciela M, Barreira-Diaz A, Vidal-Gonzalez J, Munoz-Couselo E, Martinez-Valle F, Viladomiu L, et al. Immune-related hepatitis related to checkpoint inhibitors: Clinical and prognostic factors. Liver Int (2020) 40(8):1906–16. doi: 10.1111/liv.14489
126. Dong S, Kong J, Kong F, Kong J, Gao J, Ke S, et al. Insufficient radiofrequency ablation promotes epithelial-mesenchymal transition of hepatocellular carcinoma cells through akt and ERK signaling pathways. J Transl Med (2013) 11:273. doi: 10.1186/1479-5876-11-273
127. Kong J, Kong J, Pan B, Ke S, Dong S, Li X, et al. Insufficient radiofrequency ablation promotes angiogenesis of residual hepatocellular carcinoma via HIF-1alpha/VEGFA. PloS One (2012) 7(5):e37266. doi: 10.1371/journal.pone.0037266
128. Sun C, Bai M, Ke W, Wang X, Zhao X, Lu Z. The HSP90 inhibitor, XL888, enhanced cell apoptosis via downregulating STAT3 after insufficient radiofrequency ablation in hepatocellular carcinoma. Life Sci (2021) 282:119762. doi: 10.1016/j.lfs.2021.119762
129. Choi JW, Lee JM, Kim HC, Lee M, Hur S, Jae HJ, et al. Iatrogenic arterioportal fistula caused by radiofrequency ablation of hepatocellular carcinoma: Clinical course and treatment outcomes. J Vasc Interv Radiol (2020) 31(5):728–36. doi: 10.1016/j.jvir.2019.10.020
130. Xu L, Ke S, Gao J, Sun WB. Intrahepatic hemorrhage and recurrence caused by arterioportal fistula after radiofrequency ablation for hepatocellular carcinoma: A thought-provoking ablation-related complication. Asian J Surg (2021) 44(7):996–9. doi: 10.1016/j.asjsur.2021.04.030
131. Kumar G, Goldberg SN, Gourevitch S, Levchenko T, Torchilin V, Galun E, et al. Targeting STAT3 to suppress systemic pro-oncogenic effects from hepatic radiofrequency ablation. Radiology (2018) 286(2):524–36. doi: 10.1148/radiol.2017162943
132. Ahmed M, Kumar G, Moussa M, Wang Y, Rozenblum N, Galun E, et al. Hepatic radiofrequency ablation-induced stimulation of distant tumor growth is suppressed by c-met inhibition. Radiology (2016) 279(1):103–17. doi: 10.1148/radiol.2015150080
Keywords: cryoablation, radiofrequency ablation, microwave ablation, immunotherapy, tumor treatment
Citation: Yin L, Li X-y, Zhu L-l, Chen G-l, Xiang Z, Wang Q-q, Bi J-w and Wang Q (2022) Clinical application status and prospect of the combined anti-tumor strategy of ablation and immunotherapy. Front. Immunol. 13:965120. doi: 10.3389/fimmu.2022.965120
Received: 09 June 2022; Accepted: 17 August 2022;
Published: 05 September 2022.
Edited by:
Nikolai Korpan, International Institute of Cryosurgery, AustriaCopyright © 2022 Yin, Li, Zhu, Chen, Xiang, Wang, Bi and Wang. This is an open-access article distributed under the terms of the Creative Commons Attribution License (CC BY). The use, distribution or reproduction in other forums is permitted, provided the original author(s) and the copyright owner(s) are credited and that the original publication in this journal is cited, in accordance with accepted academic practice. No use, distribution or reproduction is permitted which does not comply with these terms.
*Correspondence: Qiang Wang, wangqiang401@gmail.com
†These authors have contributed equally to this work