Defining Cost-Effective Solutions in Designing Marine Protected Areas, Using Systematic Conservation Planning
- 1AZTI, Marine Research Division, Basque Research and Technology Alliance (BRTA), Pasaia, Spain
- 2Faculty of Marine Sciences, King Abdulaziz University, Jeddah, Saudi Arabia
Environmental conservation is currently one of the main objectives of marine management. It is agreed that effective management requires evaluating the tradeoffs between protection and economic costs for negatively impacted maritime activities. For these reasons, integrated approaches combining ecological and socio-economic aspects are needed to achieve nature conservation and sustainability targets. Here, we present an approach to identify cost-effective priority marine areas for protection through a Systematic Conservation Planning method, adopting the Basque Country as case study (SE Bay of Biscay). Eight protection scenarios were defined, targeting a combination of protection features: benthic habitats, biological value of cetaceans, birds, macroalgae, and macroinvertebrates, potential provision of ecosystem services, and habitat sensitivity to human activities. In turn, the total fishing pressure produced by artisanal fisheries was adopted as a measure of the socio-economic costs of protection (assuming, for this research, that fishing would be banned in the protected areas). The results indicated that existing marine protected areas (MPAs) were very close to achieving prescribed protection targets, while these targets could be achieved by increasing the size of the existing MPAs. Higher costs were associated with the declaration of areas that were targeting a larger number of protection features. Nevertheless, cost/effectiveness was higher in these cases, with the environmental benefits outweighing a comparatively smaller increase in cost. However, the most cost-effective scenarios were those that included the extension of already existing MPAs. The method implemented can assist managers and decision makers in identifying conservation gaps and ecosystem components that require special attention. In addition, the approach can be used to develop management strategies that may be adopted under different protection scenarios. Thus, the approach proposed here could be used to inform ecosystem-based marine spatial planning.
Introduction
Anthropogenic activities and associated pressures seriously threaten ocean health (Halpern et al., 2008; Dailianis et al., 2018). Subsequently, protection of marine habitats and biodiversity is one of the most important objectives of environmental management (Laurila-Pant et al., 2015; Mazaris et al., 2019). However, effective management requires ecological and socio-economic aspects to be considered in order to achieve nature protection and maritime sector sustainability targets (Pouso et al., 2020). Evaluating the tradeoffs of alternative management strategies requires quantitative estimates of the costs and benefits of their outcomes, including the value of biodiversity, either actual or lost (Laurila-Pant et al., 2015). In addition, healthy marine ecosystems provide important benefits for humans, including health (Borja et al., 2020). Thus, marine protection is also of strategic interest for humans, as marine and coastal ecosystems are among the most productive environments in the world, and their natural capital offer a bundle of vital ecosystem services (Costanza et al., 1997; Costanza et al., 2014; Vassallo et al., 2017).
Amongst the range of possible protection measures, the utility of Marine Protected Areas (MPAs) has been demonstrated in numerous cases (Diz et al., 2018; Cheng et al., 2019). MPAs are specific areas of the sea which are reserved to protect the natural or cultural features (Kelleher and Kenchington, 1992). In addition, MPAs are an important tool for maintaining marine ecosystem functionality and health, ensuring the continued flow of ecosystem services that support human well-being (Rees et al., 2015; Rasheed, 2020). Marine Protected Areas, by protecting biodiversity, make a significant contribution to achieving healthy and functional marine ecosystems and, consequently, to providing ecosystem services that humans benefit from Borja et al. (2015), Geange et al. (2019), Hummel et al. (2019). Unfortunately, there is currently no widely accepted approach in protection planning and conservation (Katsanevakis et al., 2020).
Protecting biodiversity is a global challenge. Many Sustainable Development Goals (SDGs) cannot be met without achieving SDG 14 for ocean conservation and sustainable use. The UN’s 10% target appears insufficient to protect biodiversity, preserve ecosystem services, and achieve socio-economic priorities (O’Leary et al., 2016). In particular, the EU Biodiversity Strategy for 2030 (European Commission [EC], 2020) requires the legal protection of a minimum of 30% of the EU’s marine area, of which 10% should be strictly protected. Moreover, it is acknowledged that meeting the conservation targets requires adopting an area-based management for further development of ecological corridors, the consideration of all sectors and activities at sea (including the environmental risks that they might pose), and the consideration of MPAs within marine spatial planning (MSP) (Katsanevakis et al., 2020). Currently, when the majority of protected area designations are made opportunistically instead of systematically (Baldi et al., 2017), vague objectives often emerge from hasty planning processes (Agardy, 2017). Moreover, management measures should be adopted in a way that minimizes the potential incompatibilities between economic growth and the conservation objectives (Geange et al., 2017). Thus, accounting for the value of an area to resource users is a critical component of spatial management planning, when attempting to devise measures that will allow both biodiversity protection and sustainable resource exploitation (Pomeroy and Douvere, 2008). In this context, the achievement of conservation goals requires spatial planning strategies, which, in turn, requires tools to identify areas devoted to socio-economic development and conservation, while supporting their management in a cost-effective way (Margules and Pressey, 2000). An effective approach is the so-called systematic conservation planning (SCP) (Álvarez-Romero et al., 2018a; Kirkman et al., 2019), which avoids being overly prescriptive but has two basic principles (Smith et al., 2009). First, numerical conservation targets have to be established for each important biodiversity element that should be protected (Stratoudakis et al., 2019), setting explicit protection targets, contributing to a more transparent planning process, and giving options for consultation with stakeholders (Cowling et al., 2003). Second, it can be used for selecting new areas to be conserved, or as a complementarity-based method for selecting sites, based on how much they would add to a single existing MPA network (Margules and Pressey, 2000).
In this context, we present an approach to identify priority areas for protection using a SCP method, looking for the most cost-effective protection scenario. The SCP method was implemented by considering a combination of publicly available environmental information on benthic habitats, biological value, ecosystem services provision and habitat sensitivity to human activities, which taken together are considered as the benefits of protection. In turn, the total fishing pressure, produced by artisanal fisheries, is considered a measure of the socio-economic costs of the protection (assuming, for this research, that fishing would be banned in the protected areas) (Schmiing et al., 2014; Adame et al., 2015; Baker-Médard et al., 2019). The identification of priority areas for protection was achieved by (i) defining the protection target scenarios, (ii) identifying areas to be conserved, according to predefined scenarios, and by comparison with existing protected areas, and (iii) identifying the most robust result, in terms of cost-effectiveness.
This study contributes to current management needs in relation to the adoption of spatial protection measures under ecosystem-based MSP, by implementing a working framework to integrate the existing environmental and human activity data, and analyzing the consequences of different management options.
Materials and Methods
Case Study
The geographical area in which the approach was implemented is located in the southeast of the Bay of Biscay, in the Basque continental shelf (Figure 1). Water depth angels from coastline up to 200 m depth, with a total area of 2,500 km2 (Figure 1). The area supports high diversity and intensity of maritime activities (i.e., fishing, renewable energy, shipping, recreation, aquaculture). The management of activities is based on a combination of specific international, national and local laws that regulate the uses and environmental status (Pınarbaşı et al., 2020). MSP Directive (Directive 2014/89/EU and Boletín Oficial del Estado [BOE] (2017)) is in development stage. MPAs are still scarce, needing to be planned and made compatible with other uses.
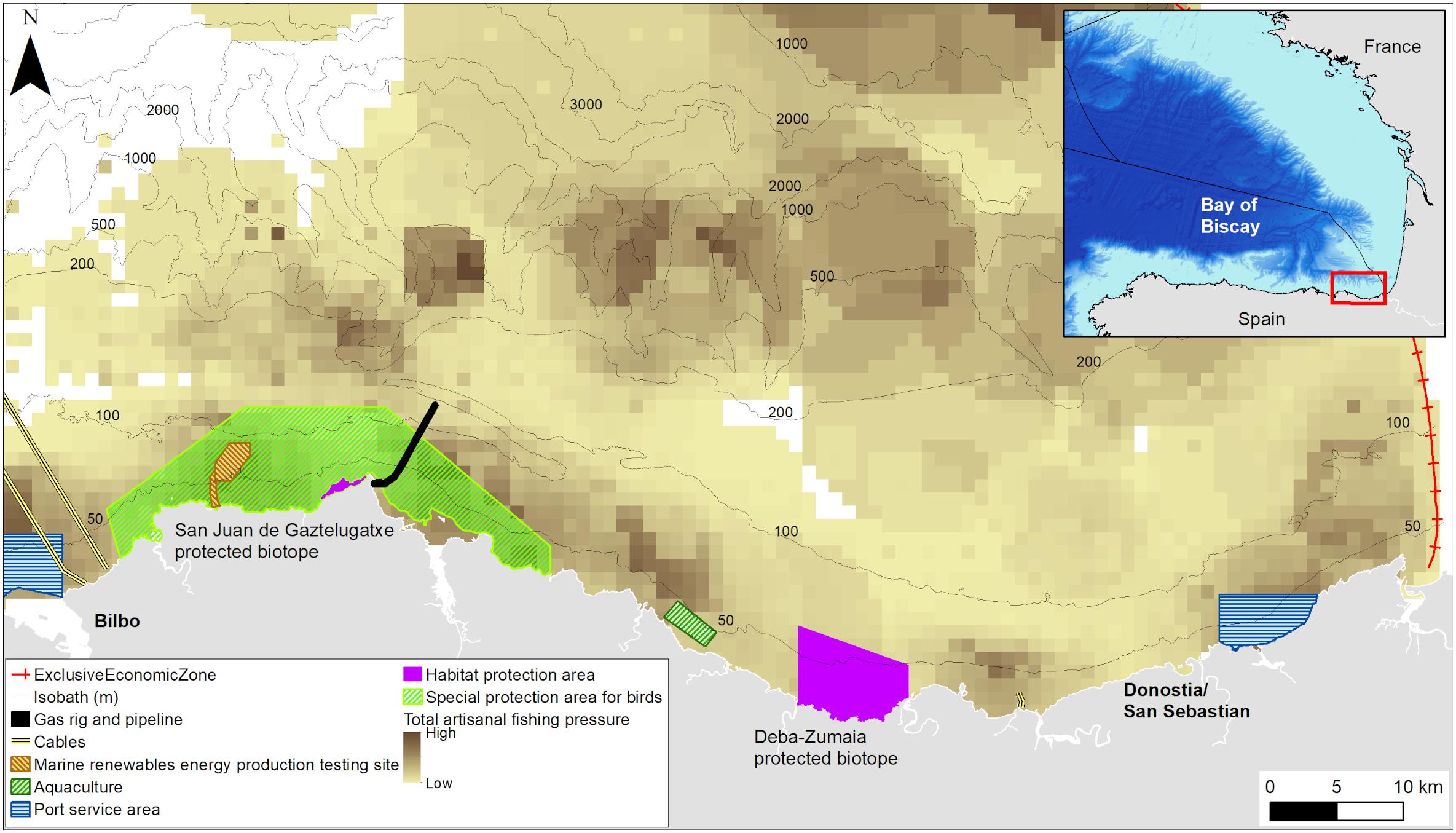
Figure 1. Geographical location of the Basque Country within the Bay of Biscay in the northeastern Atlantic Ocean. Spatial distribution of the main marine uses is shown (i.e., subsoil gas storage rig and pipeline, marine renewable energy production testing site, longline aquaculture production site, port service area for vessel waiting zone, and in background, the spatial distribution of total fishing pressure of artisanal fishery). Protected areas are divided into special protection area for birds and areas declared for benthic habitats protection.
Systematic Conservation Planning
A SCP algorithm, Marxan (Ball and Possingham, 2000; Ball et al., 2009), was used to identify candidate marine areas for protection. Marxan is a conservation site selection software that builds upon an optimization algorithm that incorporates key principles of SCP (Margules and Pressey, 2000), which include comprehensiveness (i.e., reaching multiple targets), cost-effectiveness (i.e., finding solutions at the least possible cost), and compactness [which implies a low edge to area ratio (Wilson et al., 2010)]. Marxan works with a heuristic optimization algorithm with the help of simulated annealing (Ball et al., 2009). The software aims to minimize an objective function containing the sum of opportunity costs of protection, represented by the costs of selected Planning Units (PUs), and the boundary length of the protected areas system (Schröter and Remme, 2016). Cost can be defined in economic terms or by another proxy that represents the effect of the closure of the area to an activity that used to carried out there. The objective function contains penalties for not meeting protection targets as well as for breaching a given cost threshold (Game and Grantham, 2008). Protection targets are set as a proportion of the total amount of each feature in a study area. Marxan highlights the areas to be conserved, determined by the location, the size or the shape of the areas. Thus, SCP aims to achieve a set of protection objectives whilst attempting to minimize the total cost and impact on social and economic activities (McDonnell et al., 2002). The method was selected because it enables the integration of large and diverse datasets of ecological relevance, which are used to establish the protection targets, and to be able to perform the analysis under multiple scenarios (Makino et al., 2013; Carvalho et al., 2016).
Input Data
The main criterion for the selection of the information layers was that they had already been published in scientific papers. Vectorial layers were the ones related to benthic habitats, biological value, sensitivity of benthic habitats to human activities, and ecosystem service provision capacity, which were used to define the protection targets. Artisanal fishing activity data was a 500 m raster layer, which was used as the cost to identify the areas to be protected. All the information layers were formatted and edited using ArcGIS (10.3.1) and QGIS (2.18.2) and resampled to a 1 km resolution reference grid.
The spatial distribution of benthic habitats was adopted from Galparsoro et al. (2015). The map was based on EUNIS habitat types (Davies et al., 2004), determined according to depth (biological zone), exposure to wave energy and seafloor type. In total, information regarding the spatial distribution of 12 benthic habitat types was considered (Supplementary Figure 1). In addition, biological value maps were obtained from Pascual et al. (2011). Biological value maps provide an integrated view of nature’s intrinsic non-anthropogenic value, and how this varies within a study area (Derous et al., 2007). The biological value estimation performed by Pascual et al. (2011) includes all available biological data (zooplankton, macroalgae, macrobenthos, demersal fish, seabirds, and cetaceans), from 2003 to 2010. The collated map represents the spatial distribution of the biological value according to five categories: Very High, High, Medium, Low, or Very Low (Supplementary Figure 2), following the approach by Derous et al. (2007). The same biological categories as provided by Pascual et al. (2011) were adopted to define the protection targets used as input in Marxan. A detailed description of the approach used to assess and map the biological value can be consulted in Pascual et al. (2011).
Maps of the total capacity of providing ecosystem services by benthic habitats were obtained from Galparsoro et al. (2014) (see Supplementary Figure 3 for the spatial distribution). The collated map represents the provision of potential ecosystem services according to three major evaluation classes: High, Low, Negligible/Irrelevant/Unknown (Salomidi et al., 2012). The same ecosystem service provision capacity classes as provided by Galparsoro et al. (2014) were adopted to define the protection targets used as input in Marxan.
In addition, the sensitivity of the benthic habitats to human activities was obtained from Alkiza et al. (2016) (Supplementary Figure 4). Sensitivity is the degree to which certain environmental features (in this case, benthic habitats) respond to the stress caused by the environmental conditions or human pressures (Okey et al., 2015). Sensitivity of each benthic habitat type was represented as: Negligible, Low, Medium, and High. The same benthic habitats sensitivity classes as provided by Alkiza et al. (2016) were adopted to define the protection targets used as input in Marxan.
Spatial distribution of fishing activity was obtained from Pascual et al. (2013), in which the detailed methodology used for fishing pressure calculation can be consulted. These authors calculated the total fishing pressure (measured as area covered per sampling effort) per métier unit by year (2009 and 2010), and aggregated, using data collected from 10,185 fishing trip events, questionnaires and other non-official logbooks. Fishing activity was selected because it might show greater conflicts with the declaration of a MPA (Supplementary Figure 5).
Regarding MPAs in place, two protected areas are present: Deba-Zumaia (on the eastern side with 33 km2) and San Juan de Gaztelugatxe (on the western side with 17.2 km2). A total of 50.2 km2 are under protection (2% of the study area) (Figure 1). MPAs were used to describe the current extent to which protection objectives are achieved and to be considered in Marxan runs.
PUs represent the analysis unit, and were defined as a 1-km sided square (i.e., 1 km2) (Supplementary Figure 6). The size was decided according to the spatial resolution of the available information layers, and by using a minimum size that was meaningful for management purposes. The area of each protection feature, and the total fishing pressure was calculated for each PU. Additionally, the number of protection features in each PU was calculated (Supplementary Figures 7–14).
Definition of the Protection Targets and Effectiveness of the Protection
Eight scenarios were defined to analyze the tradeoffs of the different protection options. Note that same weighting was given to each conservation feature considered in the scenarios. The definition of each scenario is given below.
• Scenario 1. This is the legally binding scenario. It targets the protection of Priority Habitats according to the European Habitats Directive (Directive 92/43/EEC). According to the Directive definition, reefs could be understood as any sublittoral hard substratum and associated benthic communities. According to the definition, the substratum is not necessarily of biological origin. In the study area, the “Reefs” (1170) priority habitat is present. For this scenario, the protection target to protect 10% of the area occupied by reef habitat.
• Scenario 2. This adopts the same target as Scenario 1 but it also includes 10% protection of the area of highest biological value for all ecosystem components.
• Scenario 3. 10% protection of the area of each benthic habitat type.
• Scenario 4. 10% protection of the area of each benthic habitat type together with the areas of highest biological value. This is the scenario targeting highest biodiversity, and not just the priority habitats cited in the legislation.
• Scenario 5. 10% protection of the area hosting the highest level of ecosystem service provision capacity.
• Scenario 6. 10% protection of the area with the most sensitive benthic habitats to human activities.
• Scenario 7. 10% protection of the area with each benthic habitat type, in combination with 10% of the area with the highest biological value, the greatest potential of providing ecosystem services, and benthic habitats with high sensitivity to human activities.
• Scenario 8. 10% protection of the area with priority habitats, the highest biological value, the greatest potential of providing ecosystem services, and 10% of the area of high sensitivity benthic habitats to human activities.
A synthesis of the combination of protection features considered in each of the scenarios is given in Table 1.
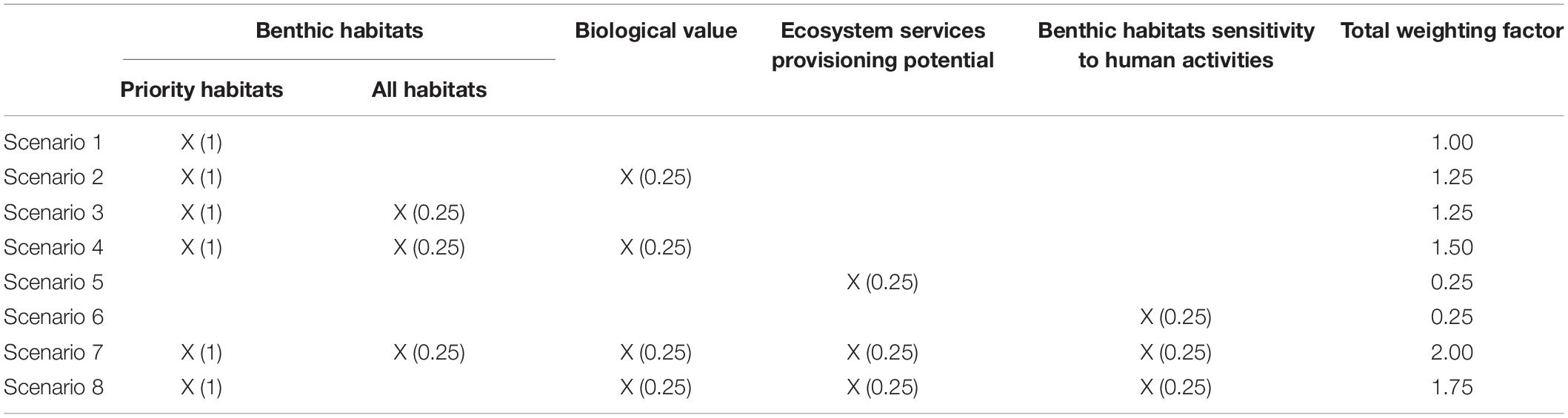
Table 1. Conservation features considered in each of the defined scenarios, including the weighting factors.
The result of each scenario was the location, the shape and the area to be declared to achieve the protection target, as well as the associated cost either as an extension of existing protected areas or independently from them. The final area obtained was weighted to determine the effectiveness of the protection at each scenario. We established a weighting value of “1” for the legally binding protection target (i.e., the priority habitats), and a value of “0.25” for each of the other features considered (Table 1). This means that Scenario 1, targeting only the legally binding protection, will not be modified in the final value of effectiveness. In turn, those scenarios with more features would be considered as having more effective protection (maximum, Scenario 7, with a weighting factor of 2), whereas scenarios not considering legally binding protection targets would be rated as less effective (minimum, Scenarios 5 and 6, with a weighting factor of 0.25) (Table 1).
Runs and Calibration
Marxan was run using CLUZ (2016.2.3) (Smith, 2019) add-in in QGIS. SCP algorithm was run twice for each scenario. First, to identify new areas of the currently declared MPAs to be independently protected, and second, to identify areas where the existing MPAs could be expanded to achieve protection targets. Each scenario was run with 100 restarts, each with one million iterations. the number of restarts did not result in significant improvements in the results, so it was kept at 100 restarts to improve processing speed.
The PUs overlapping with existing MPAs were “blocked-in” to force their selection when running Marxan. In this way, the algorithm calculated the contribution of the existing MPAs to the protection targets, and added new PUs to achieve the protection targets. For all scenarios, Marxan was run with and without the already conserved areas (present scenario). This was performed (i) to estimate the degree of achievement of protection targets according to already protected areas, (ii) to analyze the degree of overlap between the areas highlighted by Marxan with the existing protected areas, and (iii) to expand the existing protected areas to achieve the protection targets. In all cases, the cost (i.e., fishing pressure) layer was the same. This allowed the analysis of the tradeoffs between extending the existing protected areas or declaring new ones.
Marxan was set to select adjacent PUs preferentially, rather than unconnected units that might be less ecologically viable and more difficult to manage (Ball and Possingham, 2000). Reducing fragmentation levels inevitably results in more PUs being added to the portfolio, so this tradeoff was adjusted by weighting the importance of minimizing the combined external edge of the selected patches by setting a boundary-length-modifier (BLM) value (Ball and Possingham, 2000). The BLM parameter was used to clump together the PUs, to obtain results with continuous protection areas. The penalty factor was set at 10 for all protection features to give the same amount of emphasis to all features in the final solution. The selected solution was the one with the lowest BLM and penalty factor values, where protection objectives were met and costs were minimal (Martín-García et al., 2015).
The irreplaceability of each site was calculated as the proportion of solutions where this occurs for each of the scenarios (Carwardine et al., 2006; Popescu et al., 2013). The frequency of selection for each PU was averaged across all scenarios, and the standard deviation was calculated. For the effectiveness of protection estimation, the area calculated for each scenario, was multiplied by the weighting factor included in Table 1. The cost was considered as the total sum of the fishing pressure associated to each protection scenario. Dividing the cost by the effectiveness, a ratio of cost/effectiveness for each scenario, was obtained. The closest to zero, the cost/effectiveness was considered to be better in terms of protection, since with lower cost, the benefit (effectiveness) is maximized.
Results
Present Protection Status and Representativeness
Differences in the degree to which each ecosystem component is included within the existing MPA network was highlighted. As the existing MPAs were located adjacent to the coastline, the infralittoral habitats were the ones that showed the highest protected area, both in terms of sedimentary and rocky habitats (see Supplementary Table 1 for detailed information about the protection level of each ecosystem component). Meanwhile, circalittoral habitats had a lower percentage of area under protection. In three out of the four ecosystem components analyzed, no highest biological value area was under protection, and only for seabirds did the highest biological value areas show a good level of protection. The number of high biological value areas for birds was small, but it was well represented in the already protected areas. In contrast, cetaceans receive little protection within their high biological value areas because the protected areas were located very close to the coastline, and did not include the offshore zones of their highest biological value.
The number of different benthic habitat types per PU varied from one to four with highest benthic habitat diversity in areas shallower than 50 m (Supplementary Figure 7). When considering the number of benthic habitat types and priority habitats together with the zones showing high and very high biological values, the number of features varied from zero to six per PU (Supplementary Figures 9, 10), with the highest number of features also located in the shallower zones. In addition, the number of benthic habitats with capacity to provide medium and high ecosystem services capacity varied from zero to two habitats per PU (Supplementary Figure 11), being distributed in shallow water depth and around 75 m depth. It was observed that habitats showing medium to high sensitivity to human activities were mainly distributed in very shallow water depths (Supplementary Figure 12). Finally, the number of benthic habitats and priority habitats, together with very high and high biological value, medium and high capacity to provide ecosystem services, and medium and high sensitivity to human pressures, were highest in shallow water depths up to 50 m. A smaller number was found in waters up to 100 m water depth (Supplementary Figures 13, 14).
Protection Scenarios
The results for each scenario are presented as Supplementary Material (Supplementary Figures 16–21), except for Scenario 8. These results are presented here to illustrate the outcomes obtained (Figure 2).
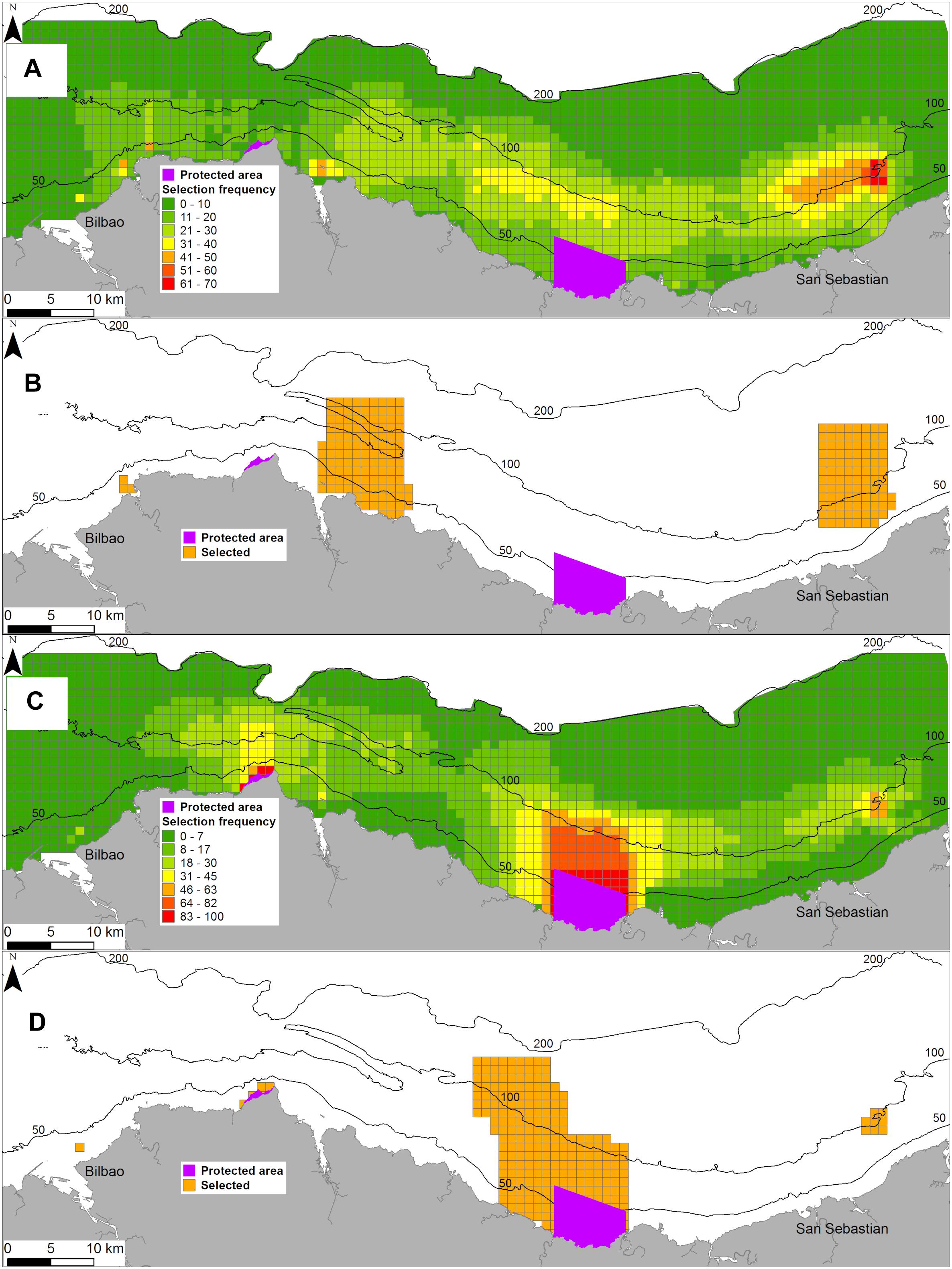
Figure 2. Frequency of selection of Planning Units (PUs) (A) and best solution (B) when the target is to conserve 10% of priority habitat types, very high biological value for ecosystem components, most sensitive benthic habitats to human activities, and the areas with the highest potential of delivering provisioning, regulating and cultural ecosystem services (Scenario 8; see section “Runs and calibration”). Frequency of selection of PUs (C) and best solution (D) for the same conservation targets but when the analysis is run considering the existing Marine Protected Areas.
The differences in protected area size, when considering existing MPAs or when the algorithm selected for a new area does not have this condition, are shown in Figure 3A. For Scenario 1, a small difference was found when the priority habitats were targeted. This means that the existing protected areas were very close to achieving the target and that the protection targets could be achieved by increasing the size of the existing MPAs by 8.7 km2 (i.e., an increase of 6.8% in the extension of the currently protected area). When the protection of highly biological value was added to the priority habitats protection, the existing protected areas should be increased by 4.3 km2, which was 3% of the currently protected area. This indicated a spatial coincidence between priority habitats and the highest biological value areas, and that they were in the surrounding areas of existing MPAs.
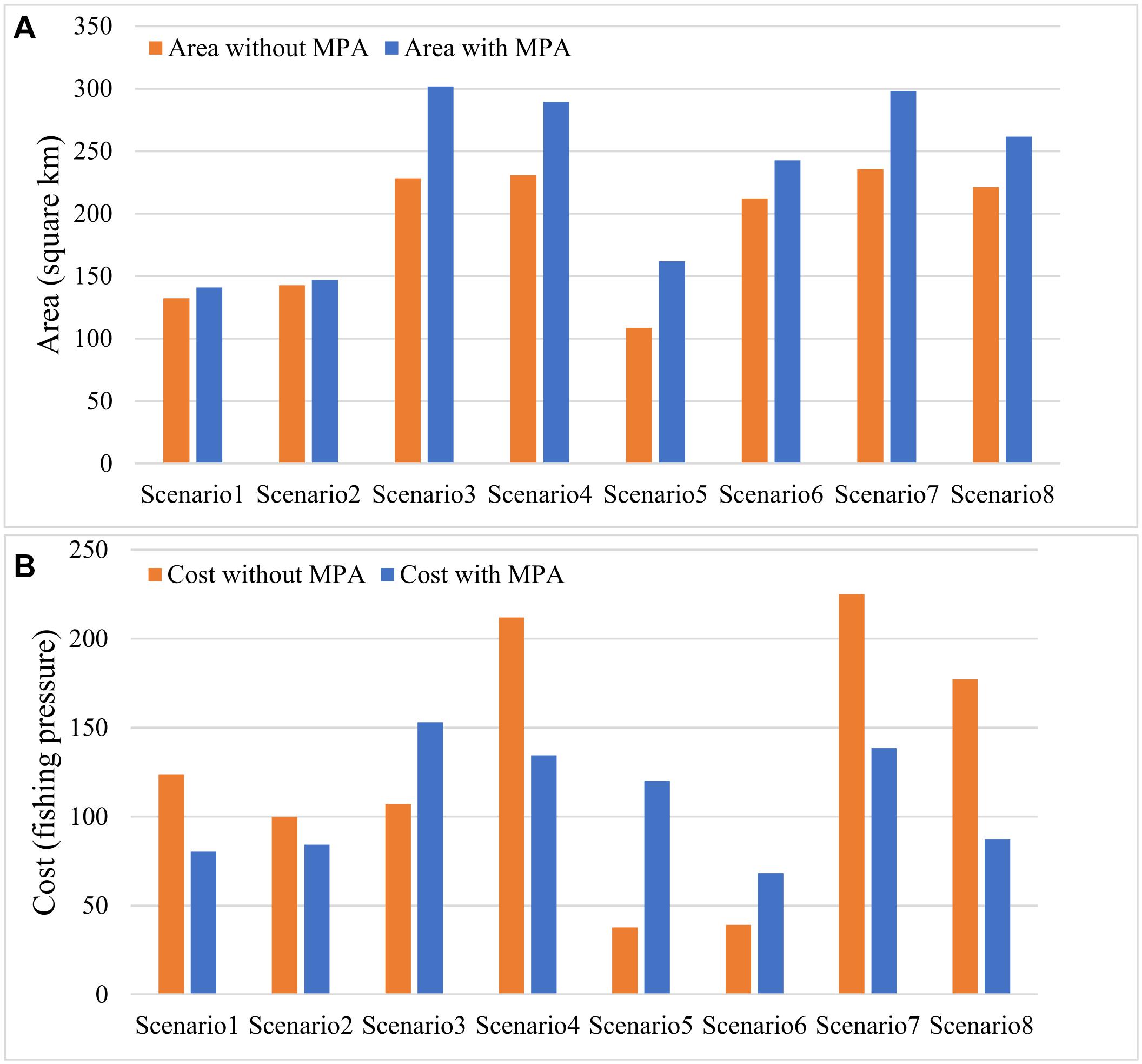
Figure 3. Total area needed to achieve the conservation targets in the eight scenarios analyzed (A); and total cost in terms of artisanal fishing pressure displacement associated with the achievement of the conservation targets (B). MPA, Marine Protected Area.
When the target was the protection of areas providing higher levels of ecosystem services (Scenario 5), the results indicated that the area to be protected, not considering already protected areas, would be smaller in comparison with the rest of scenarios (i.e., 108.6 km2) (Figure 3A). This means that, in the study area, there were hotspots of ecosystem service provision capacity and that, with a minimum size of protected area, the protection objective could be achieved. Nevertheless, when the algorithm was forced to achieve the same target considering the existing MPAs, the extension of the protected area increased up to 161.9 km2 (Figure 3A). This indicated that the protected areas and the surrounding areas were not the ones that provided the highest levels of ecosystem services, and that to achieving their protection, a greater surface area should be declared (almost 50% more should be added to the existing protected area extension).
Scenarios 7, 4, 3, 8, and 6 were the ones requiring largest MPAs (i.e., 236; 231; 228; 221, and 212 km2; respectively, when existing MPAs were not considered, and 298; 289; 302; 262, and 243 km2 when declared MPAs were considered) (Figure 3A). The results of Scenario 6 indicated an even distribution of benthic habitats of high sensitivity to human activities. In turn, Scenarios 3 and 4 shared the protection target of protecting all types of benthic habitats. Thus, both scenarios were defined to protect the highest diversity of benthic habitats, and consequently that required larger areas to cover different seafloor types and depth gradients. It must also be noted that, in Scenario 3, only the benthic habitats were considered, while in Scenario 4 the target was the protection of all benthic habitats together with the areas hosting the highest biological value areas. For both scenarios, the area to be protected, with or without considering currently protected areas, were similar (Figure 3A), indicating that the areas showing higher diversity of benthic habitats also coincided with the areas presenting higher biological values for other ecosystem components.
Scenarios 7 and 8 were defined for the protection of high biological value, highest ecosystem service provision capacity, and highest sensitivity to human activities. The difference between them was that Scenario 8 was targeting priority habitats, while Scenario 7 was targeting the protection of all habitat types. According to the outcomes and adopting the results of Scenario 8 as an example, the total area to be protected should be extended from the 50.2 km2 that is currently protected, up to 262 km2 (Figure 3A). This increases the percentage of total conserved area from the current 2% to 10.4%.
The costs associated with each of the scenarios are shown in Figure 3B. The results indicate that in five of the scenarios, the cost was lower when the algorithm was forced to select areas surrounding the already declared areas (i.e., Scenarios 1, 2, 4, 7, and 8). Thus indicating that, in absolute values, the extension of the existing protected areas would have a lower impact on fishing activity. The lowest absolute costs were found for Scenarios 5 and 6, when existing MPAs were not considered, and when the areas with the highest ecosystem services provision capacity and the highest sensitivity to human activities were targeted (i.e., 37.8 and 39.1 cost units, respectively) (Figure 3B). In contrast, when the protection target was achieved by extending the currently declared areas, the costs increased to 120 and 68.1, respectively. It must be highlighted here that, in Scenario 5, the cost was practically multiplied by three (Figure 3B), indicating that the areas surrounding the currently protected areas show the highest ecosystem services provisioning capacity, coinciding with the areas with the highest fishing activity.
In Scenario 3, the protection target was the protection of 10% the surface of all the benthic habitat types present in the study area. The results indicated that 73.5 km2 should be added to the currently protected areas (Figure 3A), and the associated cost in terms of fishing activity, would be 1.82 times higher than for Scenarios 1 and 2.
The estimation of the irreplaceability of PUs, when all scenarios were considered, was calculated based on the average number of times it was selected across all scenarios and the standard deviation (Figure 4). The largest number of times a PU was selected means that a larger number of PUs were selected considering the protection targets defined in each scenario, while the standard deviation shows the differences in PU selection across scenarios and protection targets. In both cases (i.e., considering the existing MPAs or not), the most frequently selected PUs did not coincide with the already protected areas.
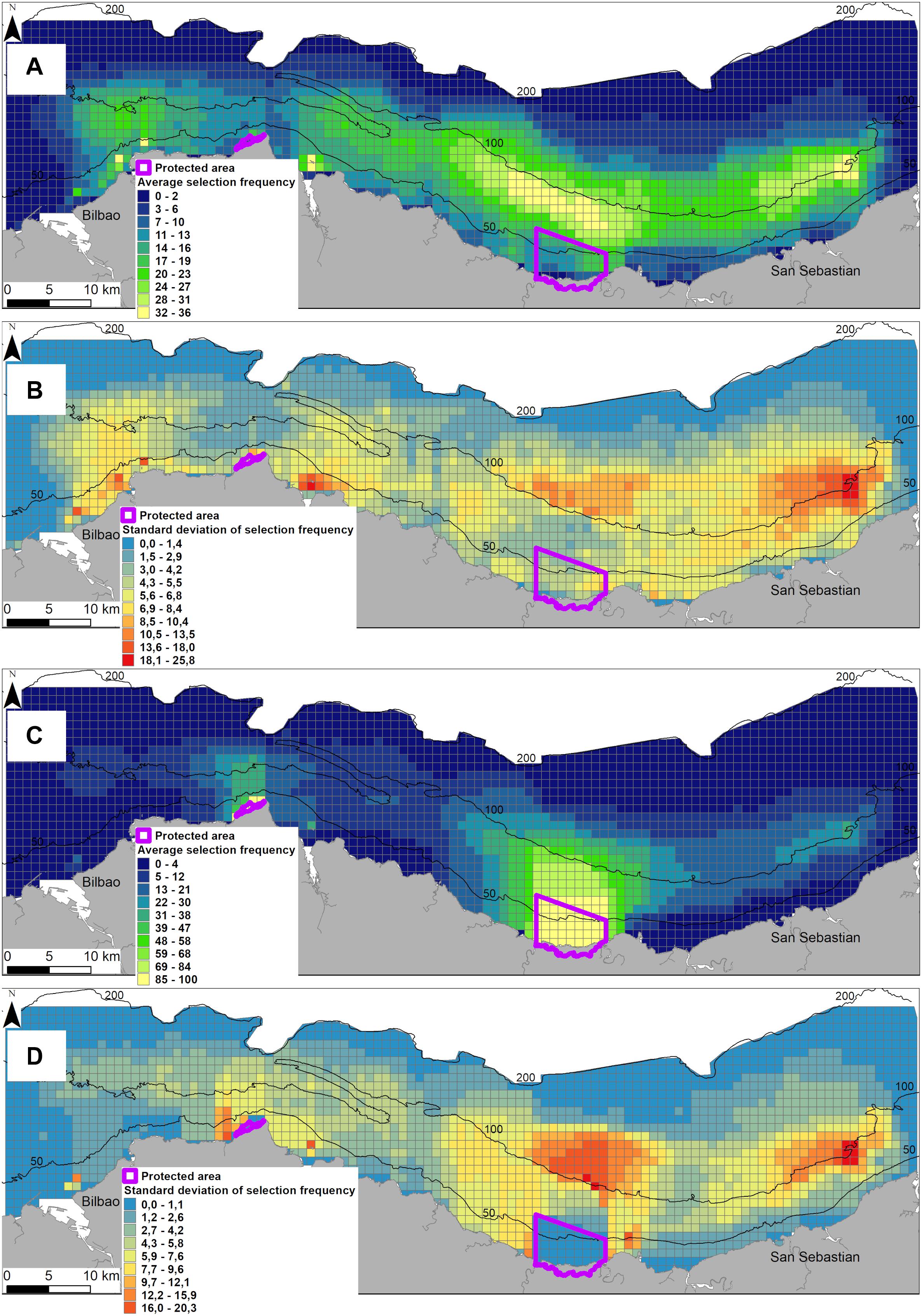
Figure 4. Average and standard deviation of the frequency of selection Planning Units for all scenarios without considering existing Marine Protected Areas (A,B) and considering the existing Marine Protected Areas (C,D).
Cost/Effectiveness
The cost-effectiveness of each scenario, and the weighting or not with the factors proposed in Table 1, is presented in Figure 5. In general, the most cost-effective solutions were those including the existing MPAs, since, for the same scenario, the ratios in those cases tended to be lower than when the MPAs were not included (except for Scenarios 3, 5, and 6; Figure 5A). This means that, for a given cost, the area protected was higher in those scenarios. When weighting the effectiveness, the ratios were still lower when including MPAs, but the scenarios can be ordered across a regression line (Figure 5B). In this case, the most effective for the cost assigned were Scenarios 8, 7 and 3, in that order and considering both with and without existing MPAs. The least effective was Scenario 5 (Figure 5B). It is interesting to note that, despite this cost-effectiveness, Scenarios 8 and 7 were protecting many more features than Scenario 3, which only included priority and all habitat types.
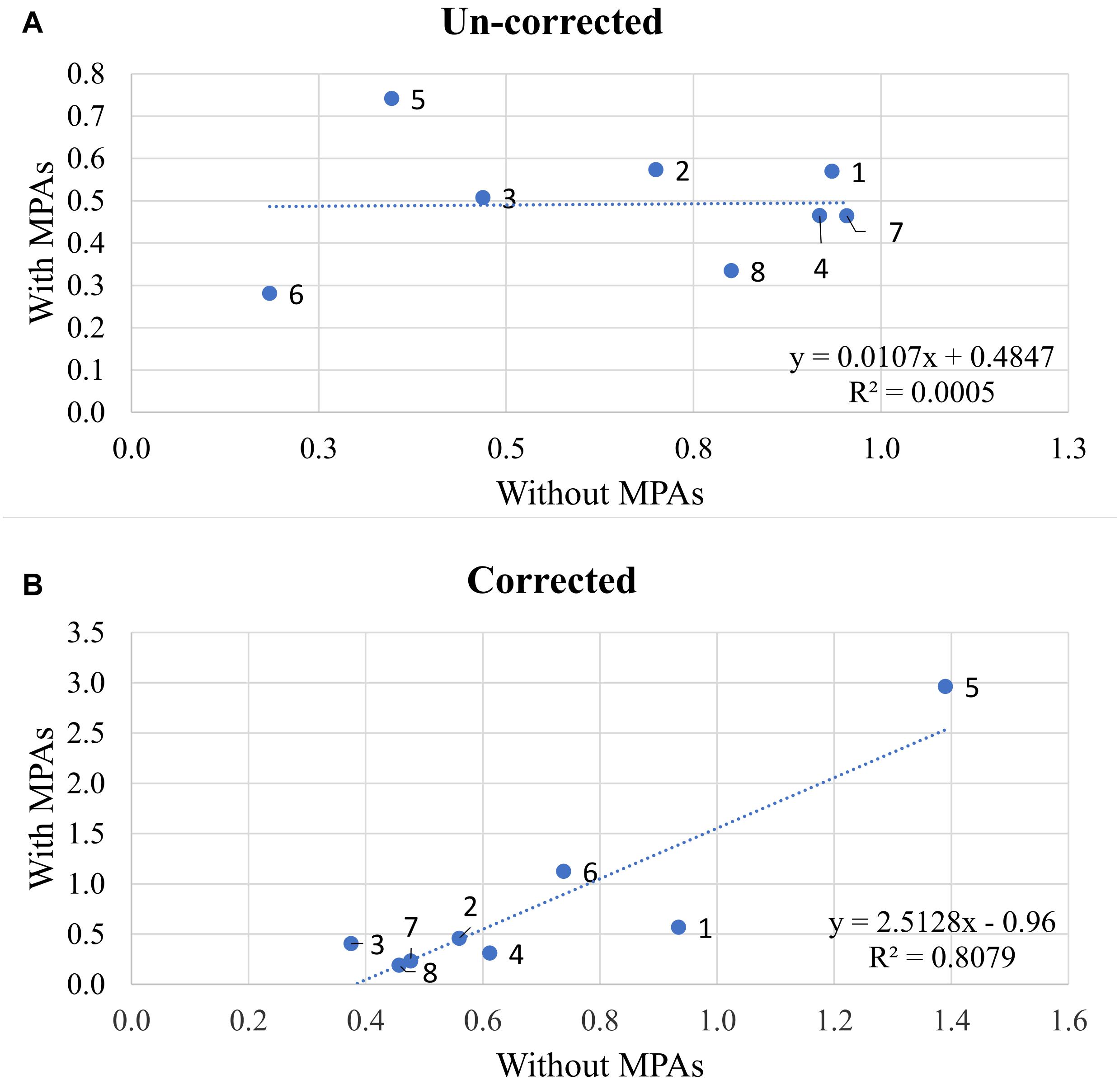
Figure 5. Cost/effectiveness ratio for each of the eight scenarios studied, with and without current Marine Protected Areas (MPAs), without correcting (A) and correcting (B) with the weighting factors proposed in Table 1.
Discussion
Selecting appropriate sites for MPAs should ensure that they cover the key habitats (benthic and/or pelagic) and ecosystem components without unnecessarily excluding other legitimate users of the marine environment (Tognelli et al., 2009; Peckett et al., 2014). In that sense, SCP provides useful functionalities for identification and provision of advice on potential areas to be protected (Álvarez-Romero et al., 2018b) in the framework of MSP. The main strength is that it allows a spatially explicit delineation of the area to be protected, in terms of minimum size and shape, while guaranteeing the fulfilment of the protection targets (including those legally binding) incurring the minimum cost, and not oversizing the area to be protected.
In this research, we present a framework that considers the integration of information of different natures and sources; from ecosystem components characteristics, such as biological value, benthic habitat distribution and their sensitivity, to human activities and the ecosystem services they provide; as well as the spatial intensity of artisanal fishery, which was previously identified as the maritime activity that would be impacted by the establishment of a no-take area (Pascual et al., 2013). Studies focusing on individual ecosystem components (i.e., benthic habitats or cetaceans) in isolation could be risky as they can underestimate the levels of biodiversity required to maintain multifunctional ecosystems (Hector and Bagchi, 2007). In particular, to the authors’ knowledge, few studies have explicitly included marine ecosystem services in SCP (Adame et al., 2015), even though there is growing support for considering ecosystem services in protection management, as they also contribute to human well-being (Bennett et al., 2015; Borja et al., 2020; Rasheed, 2020). It is acknowledged that, by explicitly valuing the costs and benefits associated with services, we may be able to achieve meaningful biodiversity protection at a lower cost and with greater co-benefits (Chan et al., 2011). Despite increasing attention to the human dimension of protection projects, a rigorous, systematic methodology for planning for ecosystem services has not been developed (Culhane et al., 2020; Farella et al., 2020). The reason is in part because flows of ecosystem services remain poorly characterized at local-to-regional scales (Wei et al., 2017), and their protection has not generally been made a priority.
The framework implemented permits an objective estimation of the achievement of protection targets under different protection scenarios, considering the present protection status. The approach can assist managers and decision makers in identifying protection gaps, and the ecosystem components which they should pay attention to (i.e., because of an under representation of such components in protected areas). In general terms, it is observed that the areas already under protection are close to the legally binding obligations for benthic habitats. However, already protected areas are not really covering the protection of areas of highest biological value, ecosystem services provision capacity or high sensitive habitats to human activities, either, which can be considered as useful additional protection features (Adame et al., 2015).
SCP permits the definition of a different set of protection targets. According to the ones defined in this research, the least costly in terms of fishing activity were Scenarios 5 and 6, but they do not achieve the legally binding targets of protecting priority habitats (Janßen et al., 2019). In turn, Scenario 3, which had very good cost-effectiveness, only covers priority and all benthic habitat types. However, when different ecological and legally binding targets are attempted, including biological value, ecosystem services provision and sensitivity of benthic habitats to human activities, too (as in Scenarios 7 and 8), it is shown that, as more ecosystem components are considered for protection, the size, and the cost of the area to be conserved increase. Both scenarios were quite similar in terms of the size of the protected areas required to achieve the protection objectives (Figure 3A). Thus, according to the available data, the areas showing highest levels of ecosystem service provisioning levels and biological value, were also the ones showing highest sensitivity to human pressures. These scenarios also showed that, when considering the existing MPAs, the size of the protected area should be increased, indicating again that the areas already under protection were not reaching the predefined protection targets. The results indicate the spatial dispersion of the targeted ecosystem components, thus requiring larger areas to achieve the protection objectives (Baker-Médard et al., 2019). In addition, the areas already under protection do not totally meet the targets defined in the scenarios, and consequently, the MPAs are not located and do not cover the optimal zones, in terms of biological value, habitats or ecosystem services. Hence, according to our results, MPAs should be extended, in new adjacent sites meet the protection targets defined in the scenarios. For example, in Scenario 8, although the cost for fishing activity is higher, and the cost-effectiveness is lower than in Scenario 3, the protection objective is more ambitious. In that particular case, it should be highlighted that the targeted number of features to be protected in Scenario 8 is higher than in Scenario 3, and consequently, the biodiversity conservation would be higher if 10.4% of the whole study area were protected. The highest costs were associated with the declaration of areas that were targeting a larger number of ecosystem components (i.e., Scenarios 7, 4, and 8), which in turn were also the ones that showed greater differences when considering the identification of new areas or the extension of existing protected ones. For example, in the case of Scenario 8, the cost, in terms of fishing effort displacement, was more than the double when a new area to be protected was identified than when the same protection target was achieved by extending the existing MPAs. This is because, within the already declared areas, there is no fishing activity, and the declaration of new zones would displace higher fishing pressure.
The results also indicate that the current fishing pressure is distributed away from existing conserved zones, and thus, increasing their area would impact less than declaring new MPAs. A conflict could be faced here, because the areas to prioritize in terms of ecosystem elements considered here, overlap the areas in which artisanal fishing activity is also high. Thus, the declaration of a no-take area would represent a negative impact on the fishing activity, and should encourage the call for reallocation of marine resource access privileges (Carter, 2003; Coccoli et al., 2018). The hypothesis is that relationships exist between the biodiversity and delivery of ecosystem services (Melià et al., 2020). The protection of ecosystem components will maintain in good ecological condition, with positive effects on the delivery of ecosystem services (Potts et al., 2014; Arkema et al., 2015), from which fishers would be one of the direct beneficiaries. In that sense, tradeoffs between the direct cost of the closure of the area and the potential benefits should also be calculated in future research (Sala et al., 2013); as well as the potential threats that anthropogenic extractive activities can pose in achieving conservation and restoration objectives (Vilas et al., 2020).
Management plans should establish structured and evidence-based instruments to guide managers to make sound decisions in accordance with the ecological needs and protection of vulnerable habitat types and species, and the SCP fulfills such conditions. Moreover, an important point to be highlighted is that, in this research, we used a spatially explicit protection planning framework to explore the trade-offs and opportunities for aligning ecological conservation goals and human activities. Systematic conservation planning demonstrates how biologically significant marine areas can be better included in MSP (Dunstan et al., 2016; Fernandes et al., 2018; Trouillet and Jay, 2021). Targeting ecosystem services directly could be expected to meet the multiple ecosystem services and biodiversity goals more efficiently, but not be a substitute for targeted biodiversity protection (Chan et al., 2006; Rees et al., 2012; Duarte et al., 2016; Farella et al., 2020).
An important aspect to be highlighted is that the quality and representativeness of background data is essential when implementing SCP algorithm, and when producing results aimed at being useful to assist in decision-making (Ardron et al., 2010). Mapping ecosystem components is often a bottleneck for protection planning, and a common excuse to justify the lack of conservation actions due to insufficient information on the distribution, state, functioning, and interactions of ecological components (Giakoumi et al., 2012). The availability of information regarding human activities (in terms of economic and social relevance), is even more difficult to collate (Korpinen et al., 2019). Fishing activity information is particularly interesting due to its spatial distribution pattern and the potential consequences of implementing MPA management measures, in terms of socio-economic costs (Bastardie et al., 2015; Vaughan, 2017; Teixeira et al., 2018; Rodríguez-Basalo et al., 2019).
The approach shown here provides different spatially explicit solutions to achieve different protection targets, not only according to legal requirements, but also, as a way of integrating more ecosystem conservation focused targets. Hence, we argue that this approach supports MSP and the ecosystem approach (also known as ecosystem-based MSP).
Conclusion
The need to increase nature protection measures is clear, but it needs to be aligned with the socio-economic sustainability of maritime sectors to be effective. Thus, integrative approaches combining ecological and socio-economic dimensions are the ones that could better inform effective management. The SCP framework offers scope for identifying representative areas for protection based on a combination of legally binding and ecological criteria. Apart from including protected habitats and species, the inclusion of biological value, ecosystem services, and habitat sensitivity in protection planning provides valuable information for biodiversity protection.
The SCP approach implemented permitted the quantitative valuation of the current conservation status against legally binding obligations and the estimation of the areas for expansion, to achieve the objectives with minimum cost for artisanal fishing activity. Moreover, the definition of scenarios based on different ecosystem components, permitted the estimation of the most cost-effective measures for protection. We considered not only priority habitats, but also other ecosystem features that would contribute to biodiversity protection. The results indicate that the inclusion of more protection features increases the size of the area to be protected, but it demonstrates that it could be a cost-effective measure. The establishment of different protection criteria and targets highlighted the irreplaceability of areas to be protected, and the definition of the size and shape of the area to be declared, minimizing the impact on artisanal fishery. Hence, the cost-effectiveness in designing conservation and protection areas, using the SCP, has been demonstrated useful for management advice. Due to such capabilities, SCP contributes to the emerging need for integrative and evidence-based approaches to inform managers in support of the ecosystem-based MSP.
Data Availability Statement
The raw data supporting the conclusions of this article will be made available by the authors, without undue reservation.
Author Contributions
IG developed the idea, wrote the first draft, and coordinated the works. IG and ÁB obtained and analyzed the results. Both authors made insights into the first drafts and contributed equally to the discussion and in writing the final manuscript.
Funding
This research was supported by the VAPEM project, funded by the Fisheries and Aquaculture Directorate of the Basque Government, and has been performed in the scope of the INTEMARES project. The LIFE IP INTEMARES project is coordinated by the Biodiversity Foundation of the Ministry for the Ecological Transition and the Demographic Challenge. It receives financial support from the European Union’s LIFE program (LIFE15 IP ES 012 INTEMARES).
Conflict of Interest
The authors declare that the research was conducted in the absence of any commercial or financial relationships that could be construed as a potential conflict of interest.
Acknowledgments
We would like to thank Bob Smith (University of Kent) for the technical support, and Branwen Val (CMB Servicios Lingüísticos) for the English revision. This paper is contribution number 1042 from AZTI BRTA (Marine Research Division).
Supplementary Material
The Supplementary Material for this article can be found online at: https://www.frontiersin.org/articles/10.3389/fmars.2021.683271/full#supplementary-material
References
Adame, M. F., Hermoso, V., Perhans, K., Lovelock, C. E., and Herrera-Silveira, J. A. (2015). Selecting cost-effective areas for restoration of ecosystem services. Conserv. Biol. 29, 493–502. doi: 10.1111/cobi.12391
Agardy, T. (2017). Justified ambivalence about MPA effectiveness. ICES J. Mar. Sci. 75, 1183–1185. doi: 10.1093/icesjms/fsx083
Alkiza, M., Galparsoro, I., Uyarra, M. C., Muxika, I., and Borja, A. (2016). Mapeo de la sensibilidad ecológica de los hábitats bentónicos frente a las actividades humanas en el noreste Atlántico. Rev. Investigación Mar. AZTI 23, 9–22.
Álvarez-Romero, J. G., Mills, M., Adams, V. M., Gurney, G. G., Pressey, R. L., Weeks, R., et al. (2018a). Research advances and gaps in marine planning: towards a global database in systematic conservation planning. Biol. Conserv. 227, 369–382. doi: 10.1016/j.biocon.2018.06.027
Álvarez-Romero, J. G., Munguía-Vega, A., Beger, M., del Mar Mancha-Cisneros, M., Suárez-Castillo, A. N., Gurney, G. G., et al. (2018b). Designing connected marine reserves in the face of global warming. Glob. Chang. Biol. 24, e671–e691.
Ardron, J. A., Possingham, H. P., and Klein, C. J. (2010). Marxan Good Practices Handbook, Version 2. Victoria, BC: Pacific Marine Analysis and Research Association, 165.
Arkema, K. K., Verutes, G. M., Wood, S. A., Clarke-Samuels, C., Rosado, S., Canto, M., et al. (2015). Embedding ecosystem services in coastal planning leads to better outcomes for people and nature. Proc. Natl. Acad. Sci. U.S.A. 112:7390. doi: 10.1073/pnas.1406483112
Baker-Médard, M., Allnutt, T. F., Baskett, M. L., Watson, R. A., Lagabrielle, E., and Kremen, C. (2019). Rethinking spatial costs and benefits of fisheries in marine conservation. Ocean Coast. Manag. 178:104824. doi: 10.1016/j.ocecoaman.2019.104824
Baldi, G., Texeira, M., Martin, O. A., Grau, H. R., and Jobbágy, E. G. (2017). Opportunities drive the global distribution of protected areas. PeerJ 5:e2989.
Ball, I. R., and Possingham, H. P. (2000). MARXAN (V1.8.2): Marine Reserve Design Using Spatially Explicit Annealing. A Manual Prepared for The Great Barrier Reef Marine Park Authority. Saint Lucia, QLD: University of Queensland, 70.
Ball, I. R., Possingham, H. P., and Watts, M. (2009). “Marxan and relatives: software for spatial conservation prioritisation,” in Spatial Conservation Prioritisation: Quantitative Methods and Computational Tools, eds A. Moilanen, K. A. Wilson, and H. P. Possingham (Oxford: Oxford University Press), 185–195.
Bastardie, F., Nielsen, J. R., Eigaard, O. R., Fock, H. O., Jonsson, P., and Bartolino, V. (2015). Competition for marine space: modelling the Baltic Sea fisheries and effort displacement under spatial restrictions. ICES J. Mar. Sci. 72, 824–840. doi: 10.1093/icesjms/fsu215
Bennett, E. M., Cramer, W., Begossi, A., Cundill, G., Díaz, S., Egoh, B. N., et al. (2015). Linking biodiversity, ecosystem services, and human well-being: three challenges for designing research for sustainability. Curr. Opin. Environ. Sustain. 14, 76–85. doi: 10.1016/j.cosust.2015.03.007
Boletín Oficial del Estado [BOE] (2017). Real Decreto 363/2017, de 8 de Abril, Por el que se Establece un Marco para la Ordenación del Espacio Marítimo. Madrid: Boletín Oficial del Estado.
Borja, A., Murillas, A., Pascual, M., and Uyarra, M. C. (2015). “Marine and coastal ecosystems: delivery of goods and services through conservation,” in Ecosystem Services and River Basin Ecohydrology, eds L. Chícharo, F. Muller, N. Fohrer, and E. Wolanski (Berlin: Springer).
Borja, A., White, M. P., Berdalet, E., Bock, N., Eatock, C., Kristensen, P., et al. (2020). Moving toward an agenda on ocean health and human health in Europe. Front. Mar. Sci. 7:37. doi: 10.3389/fmars.2020.00037
Carter, D. W. (2003). Protected areas in marine resource management: another look at the economics and research issues. Ocean Coast. Manag. 46, 439–456. doi: 10.1016/s0964-5691(03)00017-6
Carvalho, S. B., Gonçalves, J., Guisan, A., and Honrado, J. P. (2016). Systematic site selection for multispecies monitoring networks. J. Appl. Ecol. 53, 1305–1316. doi: 10.1111/1365-2664.12505
Carwardine, J., Rochester, W. A., Richardson, K. S., Williams, K. J., Pressey, R. L., and Possingham, H. P. (2006). Conservation planning with irreplaceability: does the method matter? Biodivers. Conserv. 16, 245–258. doi: 10.1007/s10531-006-9055-4
Chan, K. M. A., Hoshizaki, L., and Klinkenberg, B. (2011). Ecosystem Services in conservation planning: targeted benefits vs. co-benefits or costs? PLoS One 6:e24378. doi: 10.1371/journal.pone.0024378
Chan, K. M. A., Shaw, M. R., Cameron, D. R., Underwood, E. C., and Daily, G. C. (2006). Conservation planning for ecosystem services. PLoS Biol. 4:e379. doi: 10.1371/journal.pbio.0040379
Cheng, B. S., Altieri, A. H., Torchin, M. E., and Ruiz, G. M. (2019). Can marine reserves restore lost ecosystem functioning? A global synthesis. Ecology 100:e02617.
Coccoli, C., Galparsoro, I., Murillas, A., Pınarbas̨ı, K., and Fernandes, J. A. (2018). Conflict analysis and reallocation opportunities in the framework of marine spatial planning: a novel, spatially explicit Bayesian belief network approach for artisanal fishing and aquaculture. Mar. Policy 94, 119–131. doi: 10.1016/j.marpol.2018.04.015
Costanza, R., d’Arge, R., de Groot, R., Farber, S., Grasso, M., Hannon, B., et al. (1997). The value of the world’s ecosystem services and natural capital. Nature 387, 253–260.
Costanza, R., de Groot, R., Sutton, P., van der Ploeg, S., Anderson, S. J., Kubiszewski, I., et al. (2014). Changes in the global value of ecosystem services. Glob. Environ. Chang. 26, 152–158.
Cowling, R. M., Pressey, R. L., Sims-Castley, R., le Roux, A., Baard, E., Burgers, C. J., et al. (2003). The expert or the algorithm? Comparison of priority conservation areas in the cape floristic region identified by park managers and reserve selection software. Biol. Conserv. 112, 147–167. doi: 10.1016/s0006-3207(02)00397-x
Culhane, F. E., Robinson, L. A., and Lillebø, A. I. (2020). “Approaches for estimating the supply of ecosystem services: concepts for ecosystem-based management in coastal and marine environments,” in Ecosystem-Based Management, Ecosystem Services and Aquatic Biodiversity : Theory, Tools and Applications, eds T. G. O’Higgins, M. T. Lago, and H. DeWitt (Cham: Springer International Publishing), 105–126. doi: 10.1007/978-3-030-45843-0_6
Dailianis, T., Smith, C. J., Papadopoulou, N., Gerovasileiou, V., Sevastou, K., Bekkby, T., et al. (2018). Human activities and resultant pressures on key European marine habitats: an analysis of mapped resources. Mar. Policy 98, 1–10. doi: 10.1016/j.marpol.2018.08.038
Davies, C. E., Moss, D., and Hill, M. O. (2004). EUNIS Habitat Classification Revised 2004. Paris: European Topic Centre on Nature Protection and Biodiversity, 310.
Derous, S., Agardy, T., Hillewaert, H., Hostens, K., Jamieson, G., Lieberknecht, L., et al. (2007). A concept for biological valuation in the marine environment. Oceanologia 49, 99–128.
Diz, D., Johnson, D., Riddell, M., Rees, S., Battle, J., Gjerde, K., et al. (2018). Mainstreaming marine biodiversity into the SDGs: the role of other effective area-based conservation measures (SDG 14.5). Mar. Policy 93, 251–261. doi: 10.1016/j.marpol.2017.08.019
Duarte, G. T., Ribeiro, M. C., and Paglia, A. P. (2016). Ecosystem services modeling as a tool for defining priority areas for conservation. PLoS One 11:e0154573. doi: 10.1371/journal.pone.0154573
Dunstan, P. K., Bax, N. J., Dambacher, J. M., Hayes, K. R., Hedge, P. T., Smith, D. C., et al. (2016). Using ecologically or biologically significant marine areas (EBSAs) to implement marine spatial planning. Ocean Coast. Manag. 121, 116–127. doi: 10.1016/j.ocecoaman.2015.11.021
European Commission [EC] (2020). EU Biodiversity Strategy for 2030. Bringing Nature Back into Our Lives. COM/2020/380 Final. Brussels: European Commission.
Farella, G., Menegon, S., Fadini, A., Depellegrin, D., Manea, E., Perini, L., et al. (2020). Incorporating ecosystem services conservation into a scenario-based MSP framework: an adriatic case study. Ocean Coast. Manag. 193:105230. doi: 10.1016/j.ocecoaman.2020.105230
Fernandes, M. L., Quintela, A., and Alves, F. L. (2018). Identifying conservation priority areas to inform maritime spatial planning: a new approach. Sci. Total Environ. 639, 1088–1098. doi: 10.1016/j.scitotenv.2018.05.147
Galparsoro, I., Borja, A., and Uyarra, M. C. (2014). Mapping ecosystem services provided by benthic habitats in the European North Atlantic Ocean. Front. Mar. Sci. 1:23. doi: 10.3389/fmars.2014.00023
Galparsoro, I., Rodríguez, J. G., Menchaca, I., Quincoces, I., Garmendia, J. M., and Borja, Á (2015). Benthic habitat mapping on the basque continental shelf (SE Bay of Biscay) and its application to the European marine strategy framework directive. J. Sea Res. 100, 70–76. doi: 10.1016/j.seares.2014.09.013
Game, E. T., and Grantham, H. S. (2008). Marxan User Manual: For Marxan Version 1.8.10. Vancouver, CA: Pacific Marine Analysis and Research Association.
Geange, S. W., Leathwick, J., Linwood, M., Curtis, H., Duffy, C., Funnell, G., et al. (2017). Integrating conservation and economic objectives in MPA network planning: a case study from New Zealand. Biol. Conserv. 210, 136–144. doi: 10.1016/j.biocon.2017.04.011
Geange, S., Townsend, M., Clark, D., Ellis, J. I., and Lohrer, A. M. (2019). Communicating the value of marine conservation using an ecosystem service matrix approach. Ecosyst. Serv. 35, 150–163. doi: 10.1016/j.ecoser.2018.12.004
Giakoumi, S., Katsanevakis, S., Vassilopoulou, V., Panayotidis, P., Kavadas, S., Issaris, Y., et al. (2012). Could European marine conservation policy benefit from systematic conservation planning? Aquat. Conserv. 22, 762–775. doi: 10.1002/aqc.2273
Halpern, B. S., Walbridge, S., Selkoe, K. A., Kappel, C. V., Micheli, F., D’Agrosa, C., et al. (2008). A global map of human impact on marine ecosystems. Science 319, 948–952.
Hector, A., and Bagchi, R. (2007). Biodiversity and ecosystem multifunctionality. Nature 44:188. doi: 10.1038/nature05947
Hummel, C., Poursanidis, D., Orenstein, D., Elliott, M., Adamescu, M. C., Cazacu, C., et al. (2019). Protected area management: fusion and confusion with the ecosystem services approach. Sci. Total Environ. 651, 2432–2443. doi: 10.1016/j.scitotenv.2018.10.033
Janßen, H., Göke, C., and Luttmann, A. (2019). Knowledge integration in marine spatial planning: a practitioners’ view on decision support tools with special focus on Marxan. Ocean Coast. Manag. 168, 130–138. doi: 10.1016/j.ocecoaman.2018.11.006
Katsanevakis, S., Coll, M., Fraschetti, S., Giakoumi, S., Goldsborough, D., Mačić, V., et al. (2020). Twelve recommendations for advancing marine conservation in European and contiguous seas. Front Mar. Sci. 7:565968. doi: 10.3389/fmars.2020.565968
Kelleher, G., and Kenchington, R. (1992). Guidelines for Establishing Marine Protected Areas. A Marine Conservation and Development Report. Gland: IUCN.
Kirkman, S. P., Holness, S., Harris, L. R., Sink, K. J., Lombard, A. T., Kainge, P., et al. (2019). Using systematic conservation planning to support marine spatial planning and achieve marine protection targets in the transboundary benguela ecosystem. Ocean Coast. Manag. 168, 117–129. doi: 10.1016/j.ocecoaman.2018.10.038
Korpinen, S., Klančnik, K., Peterlin, M., Nurmi, M., Laamanen, L., Zupančič, G., et al. (2019). Multiple Pressures and Their Combined Effects in Europe’s Seas. ETC/ICM Technical Report 4/2019: European Topic Centre on Inland, Coastal and Marine Waters. Copenhagen: European Environment Agency, 164.
Laurila-Pant, M., Lehikoinen, A., Uusitalo, L., and Venesjärvi, R. (2015). How to value biodiversity in environmental management? Ecol. Indic. 55, 1–11. doi: 10.1016/j.ecolind.2015.02.034
Makino, A., Klein, C. J., Beger, M., Jupiter, S. D., and Possingham, H. P. (2013). Incorporating conservation zone effectiveness for protecting biodiversity in marine planning. PLoS One 8:e78986. doi: 10.1371/journal.pone.0078986
Martín-García, L., Sangil, C., Brito, A., and Barquín-Diez, J. (2015). Identification of conservation gaps and redesign of island marine protected areas. Biodivers. Conserv. 24, 511–529. doi: 10.1007/s10531-014-0833-0
Mazaris, A. D., Kallimanis, A., Gissi, E., Pipitone, C., Danovaro, R., Claudet, J., et al. (2019). Threats to marine biodiversity in European protected areas. Sci. Total Environ. 677, 418–426.
McDonnell, M., Possingham, H. P., Ball, I. R., and Cousins, E. (2002). Mathematical methods for spatially cohesive reserve design. Environ. Model. Assess. 7, 107–114.
Melià, P., Casagrandi, R., Di Franco, A., Guidetti, P., and Gatto, M. (2020). Protection reveals density-dependent dynamics in fish populations: a case study in the central Mediterranean. PLoS One 15:e0228604. doi: 10.1371/journal.pone.0228604
Okey, T. A., Agbayani, S., and Alidina, H. M. (2015). Mapping ecological vulnerability to recent climate change in Canada’s Pacific marine ecosystems. Ocean Coast Manag. 106, 35–48. doi: 10.1016/j.ocecoaman.2015.01.009
O’Leary, B. C., Winther-Janson, M., Bainbridge, J. M., Aitken, J., Hawkins, J. P., and Roberts, C. M. (2016). Effective coverage targets for ocean protection. Conserv. Lett. 9, 398–404. doi: 10.1111/conl.12247
Pascual, M., Borja, A., Eede, S. V., Deneudt, K., Vincx, M., Galparsoro, I., et al. (2011). Marine biological valuation mapping of the Basque continental shelf (Bay of Biscay), within the context of marine spatial planning. Estuar. Coast. Shelf Sci. 95, 186–198. doi: 10.1016/j.ecss.2011.08.031
Pascual, M., Borja, A., Galparsoro, I., Ruiz, J., Mugerza, E., Quincoces, I., et al. (2013). Total fishing pressure produced by artisanal fisheries, from a marine spatial planning perspective: a case study from the basque country (Bay of Biscay). Fish. Res. 147, 240–252. doi: 10.1016/j.fishres.2013.06.010
Peckett, F. J., Glegg, G. A., and Rodwell, L. D. (2014). Assessing the quality of data required to identify effective marine protected areas. Mar. Policy 45, 333–341. doi: 10.1016/j.marpol.2013.09.013
Pınarbaşı, K., Galparsoro, I., Alloncle, N., Quemmerais, F., and Borja, Á (2020). Key issues for a transboundary and ecosystem-based maritime spatial planning in the Bay of Biscay. Mar. Policy 120:104131. doi: 10.1016/j.marpol.2020.104131
Pomeroy, R., and Douvere, F. (2008). The engagement of stakeholders in the marine spatial planning process. Mar. Policy 32, 816–822. doi: 10.1016/j.marpol.2008.03.017
Popescu, V. D., Rozylowicz, L., Cogãlniceanu, D., Niculae, I. M., and Cucu, A. L. (2013). Moving into protected areas? Setting conservation priorities for romanian reptiles and amphibians at risk from climate change. PLoS One 8:e79330. doi: 10.1371/journal.pone.0079330
Potts, T., Burdon, D., Jackson, E., Atkins, J., Saunders, J., Hastings, E., et al. (2014). Do marine protected areas deliver flows of ecosystem services to support human welfare? Mar. Policy 44, 139–148. doi: 10.1016/j.marpol.2013.08.011
Pouso, S., Borja, Á, and Uyarra, M. C. (2020). An interdisciplinary approach for valuing changes after ecological restoration in marine cultural ecosystem services. Front. Mar. Sci. 7:715. doi: 10.3389/fmars.2020.00715
Rasheed, A. R. (2020). Marine protected areas and human well-being – a systematic review and recommendations. Ecosyst. Serv. 41:101048. doi: 10.1016/j.ecoser.2019.101048
Rees, S. E., Austen, M. C., Attrill, M. J., and Rodwell, L. D. (2012). Incorporating indirect ecosystem services into marine protected area planning and management. Int. J. Biodivers. Sci. Ecosyst. Serv. Manag. 8, 273–285. doi: 10.1080/21513732.2012.680500
Rees, S. E., Mangi, S. C., Hattam, C., Gall, S. C., Rodwell, L. D., Peckett, F. J., et al. (2015). The socio-economic effects of a marine protected area on the ecosystem service of leisure and recreation. Mar. Policy 62, 144–152. doi: 10.1016/j.marpol.2015.09.011
Rodríguez-Basalo, A., Sánchez, F., Punzón, A., and Gómez-Ballesteros, M. (2019). Updating the master management plan for El Cachucho MPA (Cantabrian Sea) using a spatial planning approach. Cont. Shelf Res. 184, 54–65. doi: 10.1016/j.csr.2019.06.010
Sala, E., Costello, C., Dougherty, D., Heal, G., Kelleher, K., Murray, J. H., et al. (2013). A general business model for marine reserves. PLoS One 8:e58799. doi: 10.1371/journal.pone.0058799
Salomidi, M., Katsanevakis, S., Borja, A., Braeckman, U., Damalas, D., Galparsoro, I., et al. (2012). Assessment of goods and services, vulnerability, and conservation status of European seabed biotopes: a stepping stone towards ecosystem-based marine spatial management. Mediterranean Marine Science 13, 49–88. doi: 10.12681/mms.23
Schmiing, M., Diogo, H., Serrão Santos, R., and Afonso, P. (2014). Marine conservation of multispecies and multi-use areas with various conservation objectives and targets. ICES J. Mar. Sci. 72, 851–862. doi: 10.1093/icesjms/fsu180
Schröter, M., and Remme, R. P. (2016). Spatial prioritisation for conserving ecosystem services: comparing hotspots with heuristic optimisation. Landsc. Ecol. 31, 431–450. doi: 10.1007/s10980-015-0258-5
Smith, R. J. (2019). The CLUZ plugin for QGIS: designing conservation area systems and other ecological networks. Res. Ideas Outcomes 5:e33510.
Smith, R. J., Eastwood, P. D., Ota, Y., and Rogers, S. I. (2009). Developing best practice for using marxan to locate marine protected areas in European waters. ICES J. Mar. Sci. 66, 188–194. doi: 10.1093/icesjms/fsn198
Stratoudakis, Y., Hilário, A., Ribeiro, C., Abecasis, D., Gonçalves, E. J., Andrade, F., et al. (2019). Environmental representativity in marine protected area networks over large and partly unexplored seascapes. Glob. Ecol. Conserv. 17:e00545. doi: 10.1016/j.gecco.2019.e00545
Teixeira, J. B., Moura, R. L., Mills, M., Klein, C., Brown, C. J., Adams, V. M., et al. (2018). A habitat-based approach to predict impacts of marine protected areas on fishers. Conserv. Biol. 32, 1096–1106. doi: 10.1111/cobi.12974
Tognelli, M. F., Fernández, M., and Marquet, P. A. (2009). Assessing the performance of the existing and proposed network of marine protected areas to conserve marine biodiversity in Chile. Biol. Conserv. 142, 3147–3153. doi: 10.1016/j.biocon.2009.08.016
Trouillet, B., and Jay, S. (2021). The complex relationships between marine protected areas and marine spatial planning: towards an analytical framework. Mar. Policy 127:104441. doi: 10.1016/j.marpol.2021.104441
Vassallo, P., Paoli, C., Buonocore, E., Franzese, P. P., Russo, G. F., and Povero, P. (2017). Assessing the value of natural capital in marine protected areas: a biophysical and trophodynamic environmental accounting model. Ecol. Model. 355, 12–17. doi: 10.1016/j.ecolmodel.2017.03.013
Vaughan, D. (2017). Fishing effort displacement and the consequences of implementing marine protected area management – an english perspective. Mar. Policy 84, 228–234. doi: 10.1016/j.marpol.2017.07.007
Vilas, D., Coll, M., Corrales, X., Steenbeek, J., Piroddi, C., Calò, A., et al. (2020). The effects of marine protected areas on ecosystem recovery and fisheries using a comparative modelling approach. Aquat. Conserv. 30, 1885–1901. doi: 10.1002/aqc.3368
Wei, H., Fan, W., Wang, X., Lu, N., Dong, X., Zhao, Y., et al. (2017). Integrating supply and social demand in ecosystem services assessment: a review. Ecosyst. Serv. 25, 15–27. doi: 10.1016/j.ecoser.2017.03.017
Keywords: marine spatial planning, Marxan, marine conservation, ecosystem services, spatial management, human activities
Citation: Galparsoro I and Borja Á (2021) Defining Cost-Effective Solutions in Designing Marine Protected Areas, Using Systematic Conservation Planning. Front. Mar. Sci. 8:683271. doi: 10.3389/fmars.2021.683271
Received: 20 March 2021; Accepted: 18 June 2021;
Published: 07 July 2021.
Edited by:
Carolyn J. Lundquist, National Institute of Water and Atmospheric Research (NIWA), New ZealandReviewed by:
Joanna Maria Pitt, Department of Environment and Natural Resources, BermudaTom Brough, National Institute of Water and Atmospheric Research (NIWA), New Zealand
Copyright © 2021 Galparsoro and Borja. This is an open-access article distributed under the terms of the Creative Commons Attribution License (CC BY). The use, distribution or reproduction in other forums is permitted, provided the original author(s) and the copyright owner(s) are credited and that the original publication in this journal is cited, in accordance with accepted academic practice. No use, distribution or reproduction is permitted which does not comply with these terms.
*Correspondence: Ibon Galparsoro, igalparsoro@azti.es