Sounds of snapping shrimp (Alpheidae) as important input to the soundscape in the southeast China coastal sea
- 1State Key Laboratory of Marine Environmental Science, College of the Environment and Ecology, Xiamen University, Xiamen, China
- 2State Key Laboratory of Marine Environmental Science, College of Ocean and Earth Sciences, Xiamen University, Xiamen, China
- 3Key Laboratory of Underwater Acoustic Communication and Marine Information Technology of the Ministry of Education, Xiamen University, Xiamen, China
- 4Dongshan Swire Marine Station, Xiamen University, Dongshan, China
- 5South China Sea Fisheries Research Institute of the Chinese Academy of Fishery Sciences, Key Laboratory of South China Sea Fishery Resources Exploitation and Utilization of the Ministry of Agriculture and Rural Affairs, Guangzhou, China
As a biological sound source widely distributed in temperate and tropical coastal waters, snapping shrimp produce strong pulses which can serve as honest signals to indicate habitat-specific soundscape. The past decade has witnessed the growing interest in investigating the acoustical activity of snapping shrimp across many shallow waters including the coastal line of the west Pacific Ocean. It was extended to the Southeast China coastal area where snapping sounds and the associated soundscape were recorded at four sites. Customized codes incorporating bandwidth and amplitude threshold operations were developed to detect snaps from the ambient noise to estimate snap rate and extract snaps individually. The subsequent analysis suggested that snaps recorded at different sites were unanimously stronger than background noise. Sound pressure level of the snaps ranged from 150 dB to 190 dB (re 1 μPa). The characteristics of snaps, including sound pressure level, duration, peak frequency, -3dB bandwidth from different sites are examined to evaluate the variability across the sites. Though snapping pulses had peak frequencies and the -3 dB bandwidth consistently below 10 kHz, snaps had considerable energy extending to the high frequency range over 200 kHz. The analysis of the acoustic data received for 7 consecutive days at one site indicated that the snap rate corresponded to tidal level periodicity. A high tide was accompanied with a local high snap rate regardless of light but this local snap rate peak was much higher at night. The mean rate fluctuated between 2000 and 4000 snaps per minute and more snaps were recorded after sunset suggesting that snapping shrimp living in the area snapped in response to light. These data may indicate that snaps are important communication means in light-limited condition and deepen our understanding on the correlation of snapping behavior and ecological environments.
1 Introduction
The ocean is a natural reservoir for sounds originating from biological, geophysical, and anthropogenic processes (Wenz, 1962; Krause, 2008; Duarte et al., 2021). The increasing anthropogenic activities, including worldwide shipping, platform construction and wind farm operation are massive contributions to intensifying the ocean soundscape (McDonald et al., 2006; Slabbekoorn et al., 2010; Herbert-Read et al., 2017; Harding et al., 2019; Mooney et al., 2020). Marine animals, including invertebrates, have evolved to sense sounds and cue on environment acoustically to facilitate survival (Mann and Lobel, 1997; Hawkins and Amorim, 2000; Giorli et al., 2016; Van Oosterom et al., 2016; Erbe et al., 2017). For example, snapping shrimp are capable of producing sounds over 200 dB as an important input to the overall soundscape (Au, 1993; Au and Banks, 1998; Song et al., 2021). Biological activities from snapping shrimp along the coastal line have considerable effects on the habitat-specific soundscape (Everest et al., 1948; McClure and Wicksten, 1997; Fay, 2009; Monczak et al., 2017; Monczak et al., 2019).
Snapping sounds cover a wide range of frequency and present a highly diurnal dependence and seasonal variation, dominating over other sounds in shallow waters (Bohnenstiehl et al., 2016; Butler et al., 2017; Lillis and Mooney, 2018; Monczak et al., 2019; Mueller et al., 2020). Shrimp use its big claw to eject water, resulting in a cavitating bubble collapse and generation of a broadband pulse with energy extending to over 200 kHz (Versluis et al., 2000). Snapping shrimp has various dwelling sites including coral reefs, kelp, mangrove and oyster reefs. Sounds generated by snapping shrimps have induced numerous studies since World War II and snapping shrimp were previously thought to distribute in the tropical and subtropical zones. Many later studies have reported the sound activity of snapping shrimp in higher latitudes (Watanabe et al., 2002; Mathias et al., 2016; Bibikov and Makushevich, 2020; Lee et al., 2021).
Snaps can serve in various ways to meet the daily demands for shrimp in fighting for shelter protection, preying, rock-boring, excavation, and communicating (Nolan and Salmon, 1970; Schein, 1975; Schein, 1977; Schmitz and Herberholz, 1998). The size of the open major chela, and the resulting water jet and snap pulse are all signals produced during intraspecific encounters (Hughes, 2000). The snapping claw as a mechanosensory stimulus can be detected by setae on the major chela of the encountering competitors but both the physical size and water jet have a limited working distance (Herberholz and Schmitz, 1999). In comparison, the snapping pulse can propagate to a great distance and possibly used for group coordination (Toth and Duffy, 2005). The synchronizing snapping was reported in a previous study, raising a question on whether snapping shrimp can acoustically sense the snaps, which was examined recently in snapping shrimp (Alpheus richardsoni), suggesting this species is sensitive to sounds ranging from 80 to 1500 Hz (Dinh and Radford, 2021). Snaps are broadband signals with considerable energy below 1500 Hz, meaning that snaps may be used for acoustic signaling between conspecifics. The communication range will depend on the source level of the snaps.
The snapping either used for communication or as aggressive behaviors presented a diurnal pattern. Snaps were found to peak at dusk and dawn (Radford et al., 2008; Lammers et al., 2008; Lillis and Mooney, 2018), following a diurnal pattern to some extent. But they shift their show preferential snapping time from nighttime in the summer to daytime in the winter in the West Bay Oyster Reserve, Pamlico Sound North Carolina (Bohnenstiehl et al., 2016). A transition between dominant daytime at different seasons was observed and it may relate to light availability. These changes of snapping behavior with season were also reported in the Coastal Sea of Western Jeju, part of the western Pacific Ocean (Jeong and Paeng, 2022). The most frequent snapping events were found at night in late summer. But in late fall, snap rate was not the highest at night and dropped like the one during the day. A high tide was always accompanied with a higher snap rate by 13% than at low tide and the temporal variation of snap rate time was parallel to that of current speed during high/low tides with a time lag of about 1.25 h (Lee et al., 2021). These results paved us the way for further studies on what drives the temporal variations of snap rate during the day in different seasons and tidal levels. The biological characteristics of snapping shrimp may account for the snap rate variation to some extent.
Snaps produced from 42 snapping shrimp individuals of Synalpheus paraneomeris and 20 A. angulosus specimens were examined in acoustic measurements (Au and Banks, 1998; Song et al., 2021). They found that snaps have peak frequencies unanimously between 2 and 5 kHz. Peak-to-peak source levels varied from 183 to 189 dB (re 1 μPa) for S. paraneomeris and ranged from 164.9 to 187.7 dB (re 1 μPa) for A. angulosus (Au and Banks, 1998; Song et al., 2021). The laboratory measurements provide good controls to estimate the source level of snaps because the animals can be physically fixed 1 m away from the recording facility. In comparison, it is challenging to estimate the actual source level of snaps recorded from field. Snaps from the field and laboratory were compared for shrimp in the May River estuary, both showing a generally broadband property and the majority of energy was confined to below 10 kHz. Laboratory snaps had a much higher power spectral density than those of the field snaps, which may result from the attenuation due to the long travelling distance (Song et al., 2021). There might be other parameters that can lead to variability of snaps produced in the field, including anthropogenic noise (Spiga, 2022).
Among numerous studies on snapping shrimp sounds, only a few examine the acoustic characteristics of snaps in detail (Au and Banks, 1998; Song et al., 2021; Spiga, 2022) and in this paper, we added extra acoustic analysis on peak frequency, duration, -3dB bandwidth and sound pressure level of snaps recorded at four different sites in shallow water of southeast China coastal area. These sites located at two adjacent provinces and data were collected to probe into the temporal variation of the estimated snap rate and its correlation with tidal level. This study contributes to soundscape research concerning snapping shrimp in this region and provide information to probe into the sound habitat-specific underwater soundscape along the southeast China coastal line.
2 Materials and methods
2.1 Acoustic survey
Snaps were recorded at four different sites along the shallow waters of southeast China coastal area, with two sites at Fujian province and the rest two at Guangdong province (Figure 1).
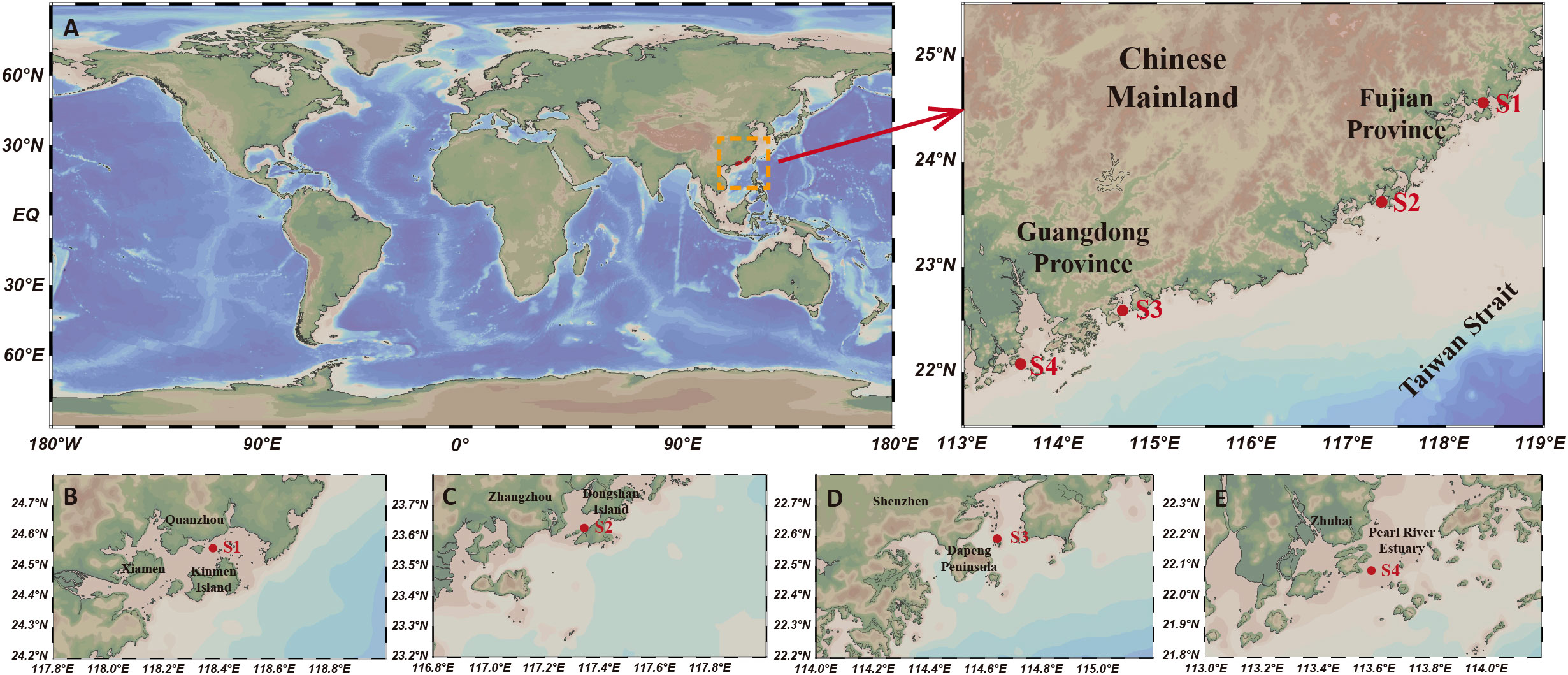
Figure 1 (A) An overall view of the location of the experimental sites S1, S2, S3 and S4 from both a global and regional context, with the four recording sites marked in red. (B) Recording site at Xiamen Bay (Xiamen, China), (C) Zhao'an Bay (Zhangzhou, China), (D) Daya Bay (Shenzhen, China) and (E) Pearl River estuary (Zhuhai, China). Figure was drawn using Ocean Data View (Schlitzer, R., Ocean Data View, https://odv.awi.de, 2021).
A compact recording system, SoundTrap recorder (ST 300 HF, Ocean Instruments Ltd, New Zealand), with a linear frequency range of 20 Hz - 150 kHz was used in experiments at site 1, site 3 and site 4. Another sound recorder (ST 600 HF, linear frequency range: 20 Hz - 150 kHz) was deployed at site 2, which was physically fixed to a buoy. The ST 300HF and ST 600 HF are both compact underwater audio recording system containing a single channel, with a low self-noise level (less than 38 dB re 1 μPa above 2 kHz), a user programmable preamplifier and a 16-bit analog-to-digital converter. The sampling rate can reach as high as 576 kHz for ST 300 HF and 384 kHz for ST 600 HF. The preamplifier and ADC converter, batteries were embedded inside the main body and the tool functioned as one unit, with a 256 GB storage memory for ST 300 HF and Up to 2TB of data storage for ST 600 HF. The recorder was hung in the water column through either a steel bar with clips at the end or physically fixed to the buoy. The recording sites had a water depth of 12.0 m, 11.0 m, 12.0 m, and 4.0 m and the recorder was placed 2.0 m, 2.0 m, 3.0 m, and 1.5 m underwater at site 1, site 2, site 3, and site 4, with a sampling frequency of 576 kHz, 96 kHz, 192 kHz and 576 kHz, respectively.
2.2 Snap analysis
Shrimp snap is thought to have extremely typical characteristics of broadband energy and high amplitude (Au and Banks, 1998; Song et al., 2021). We referred to previous studies and used an envelope correlation algorithm combined with an amplitude threshold to detect snaps (Bohnenstiehl et al., 2016; Lillis and Mooney, 2018; Lee et al., 2021). Customized Matlab scripts were developed to extract individual snaps. Snap spectrogram was calculated using the short-time Fourier transform, using a 192-point fast Fourier transform (FFT) and a rectangular window of 1 ms. These settings provided a frequency resolution of 500 Hz.
After extraction, snaps from four recording sites were compared to address the potential variations. To begin, acoustic parameters including peak-to-peak sound pressure level (SPL), duration, peak frequency and -3dB bandwidth were calculated following previous studies (Madsen and Wahlberg, 2007; Au et al., 2016; Song et al., 2021). SPL was determined after calibration using the sensitivity of SoundTrap recorders. Duration was determined a time length covering 95% of the total pulse energy. Peak frequency was defined as the frequency point at which the spectral level had the highest value. The -3 dB bandwidth was the difference of two frequency points between which the spectral level was lower than the maximum level by 3 dB.
The snap parameters were tested to examine their normality using Shapiro-Wilk method. Either the ANOVA analysis or the Kruskal-Wallis analysis of variance (ANOVA) analysis was used to determine the differences in acoustic properties of the snaps recorded at different sites. All statistical analyses were tested at significance level of 0.05.
2.3 Examination on snap rate at site 2
The temporal pattern of the snap rate and soundscape concerning were examined using the data of the longest recording length (site 2) to probe into the soundscape and its temporal variation throughout the recording time. Considering the sampling frequency at site 2 was 96 kHz, we divided the recording bandwidth into 3 sub-bandwidths to quantify soundscape: a low sub-bandwidth from 0-1.5 kHz representing the fish sounds and boat noise; a middle sub-bandwidth from 1.5-10 kHz as an index of snapping shrimp sounds and a high sub-bandwidth from 10-48 kHz. The mean power spectral density was calculated for these bandwidths and the whole bandwidth from 0-48 kHz for every minute. The output was tracked with time for 7 consecutive days. The number of snaps per minute was calculated to estimate snap rate and the resulting temporal pattern. The sound energy within bandwidth II was used to estimate the temporal change of snapping shrimp sounds because the peak frequency of the snap was confined to this bandwidth (Au and Banks, 1998; Schmitz, 2002; Song et al., 2021). The power spectral densities of bandwidth I, bandwidth II, bandwidth III and bandwidth IV can be compared to evaluate the influence of snapping shrimp sounds to the overall soundscape at different habitats.
3 Results
3.1 Acoustic properties of snaps across different sites
A representative shrimp snap train with continuous snaps showed that the thresholding method was effective to extract individual snaps from the long time series (Figures 2A, B). Snap (Figures 2C, D) presents a broadband property with frequency peaking at 3.8 kHz and it can be seen that energy was extended to over 100 kHz. The amplitude difference across 4 octaves beginning at peak frequency was less than 20 dB. The snap was composed of a precursor with relatively low amplitude and a short pulse characterized by its rapid onset and high amplitude. These features were similar to snaps reported in previous studies on snapping shrimp species, A. heterochaelis, A. angulosus, and S. parneomeris (Au and Banks, 1998; Versluis et al., 2000; Song et al., 2021).
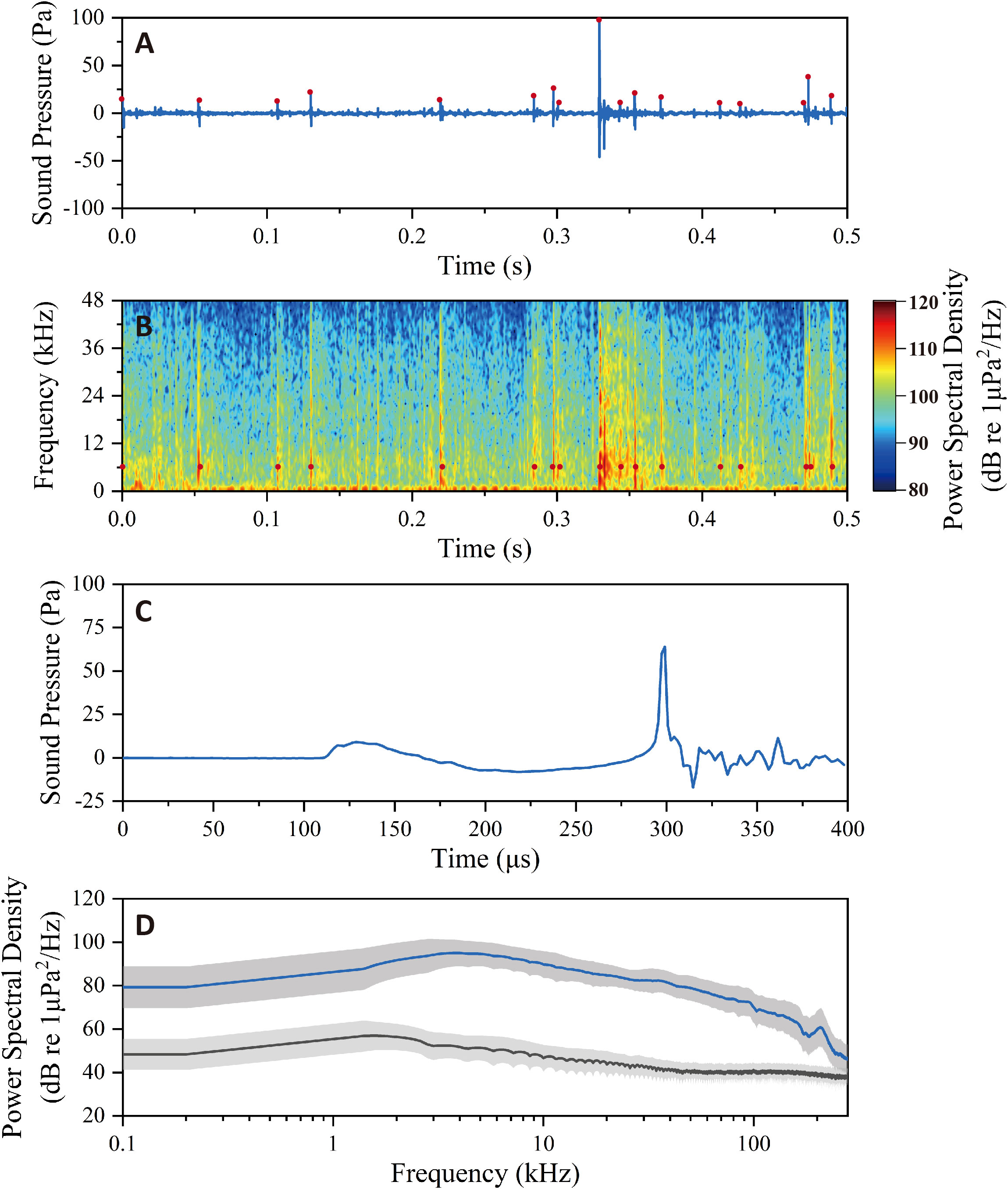
Figure 2 (A) A series of snaps in time domain and (B) time-frequency domain, with the red dots representing the detected snaps. (C) Waveform of a representative snap, showing a low amplitude precursor and a distinct high amplitude pulse. (D) Power spectral density of the snap (upper line) and its comparison to background noise (lower line). These data were recorded at site 1.
The characteristic spectrum was similar among snaps recorded at different sites (Figure 3), all showing a broadband distribution. Snaps unanimously had higher energy than background noise. There were variabilities in snap and background noise amplitudes. Using power spectral density as reference (Figure 3), the greatest amplitude difference between snap and background noise was reflected in data from site 3, reaching 59 dB at peak frequency 2.3 kHz. Snaps from site 3 had the highest amplitude, followed by site 2 and site 4, and lowest amplitude snaps was recorded at site 1. The analysis of data from site 4 suggested the spectral lines of snaps and background noise were similar below 2 kHz. Background noise at site 4 decreased rapidly for frequencies greater than 2 kHz and was close to that of site 3 at frequencies higher than 10 kHz.
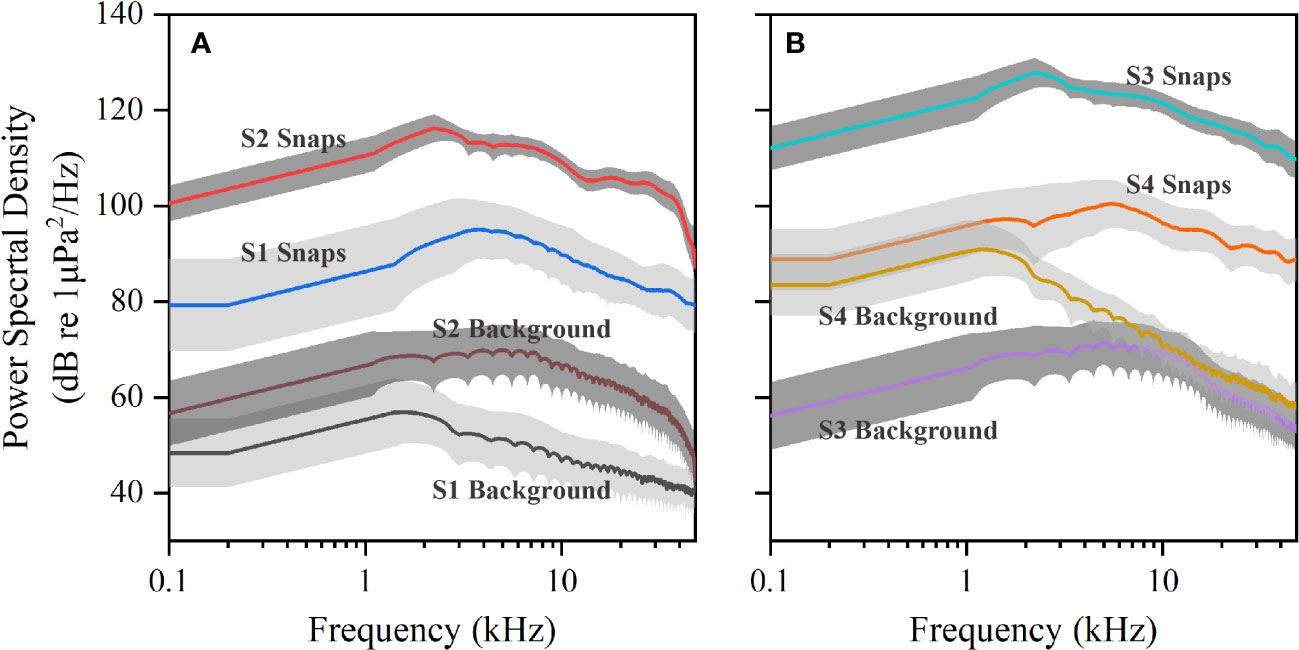
Figure 3 The comparison between the mean and SD (gray shading) frequency spectra of snaps and local background noise in decibels for (A) Site 1 and Site 2 and (B) Site 3 and Site 4.
Duration of snaps from site 3 followed a normal distribution (p=0.28) and the rest parameters all followed a non-normal distribution (p<0.001), shown in Figure 4. Thus, the Kruskal-Wallis ANOVA was used to compare the data across different sites, showing a significant difference for duration (p<0.001), peak frequency (p<0.001), -3 dB bandwidth (p<0.001), and sound pressure level (p<0.001). Snaps recorded at site 3 had the highest sound pressure level, with a mean value of 186.2 ± 1.3 dB re 1μPa (n=750) and ranged from 183.8 to 190.1 dB re 1μPa. In comparison, the mean sound pressure level of snaps recorded at site 1 was 156.0 ± 4.4 dB re 1μPa (n=482), which was the lowest among these recording sites. These values were 172.5 ± 1.7 dB re 1μPa (n=629) and 165.5 ± 3.9 dB re 1μPa (n=111) for snaps from site 2 and site 4 respectively. The amplitude range of sound pressure level were 20.6, 8.9, 6.3 and 19.0 dB for site 1, site 2, site 3 and site 4 respectively. The mean durations were 312.8 ± 87.6, 476.1 ± 55.3, 452.9 ± 78.6, and 575.6 ± 206.5 μs accordingly. Snaps at all sites had a mean peak frequency consistently below 5 kHz and -3 dB bandwidth lower than 10 kHz, which may facilitate a long range propagation. More detailed comparisons of acoustic parameters are shown in Table 1.
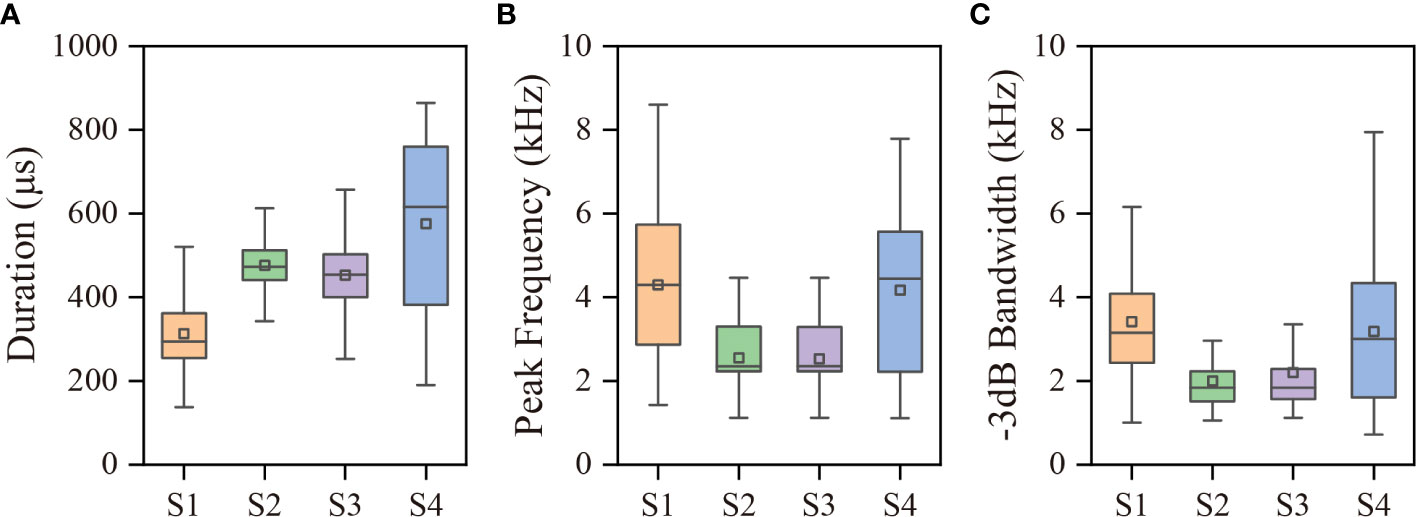
Figure 4 The comparisons of snap (A) Duration, (B) Peak frequency, and (C) -3dB bandwidth across the recording sites, where the box bottom and top denote the 25% and 75% percentile of the distribution, and the line extensions of the box represent the lower and upper edge values, respectively. The median and mean are represented by the line and square inside the boxes, respectively.
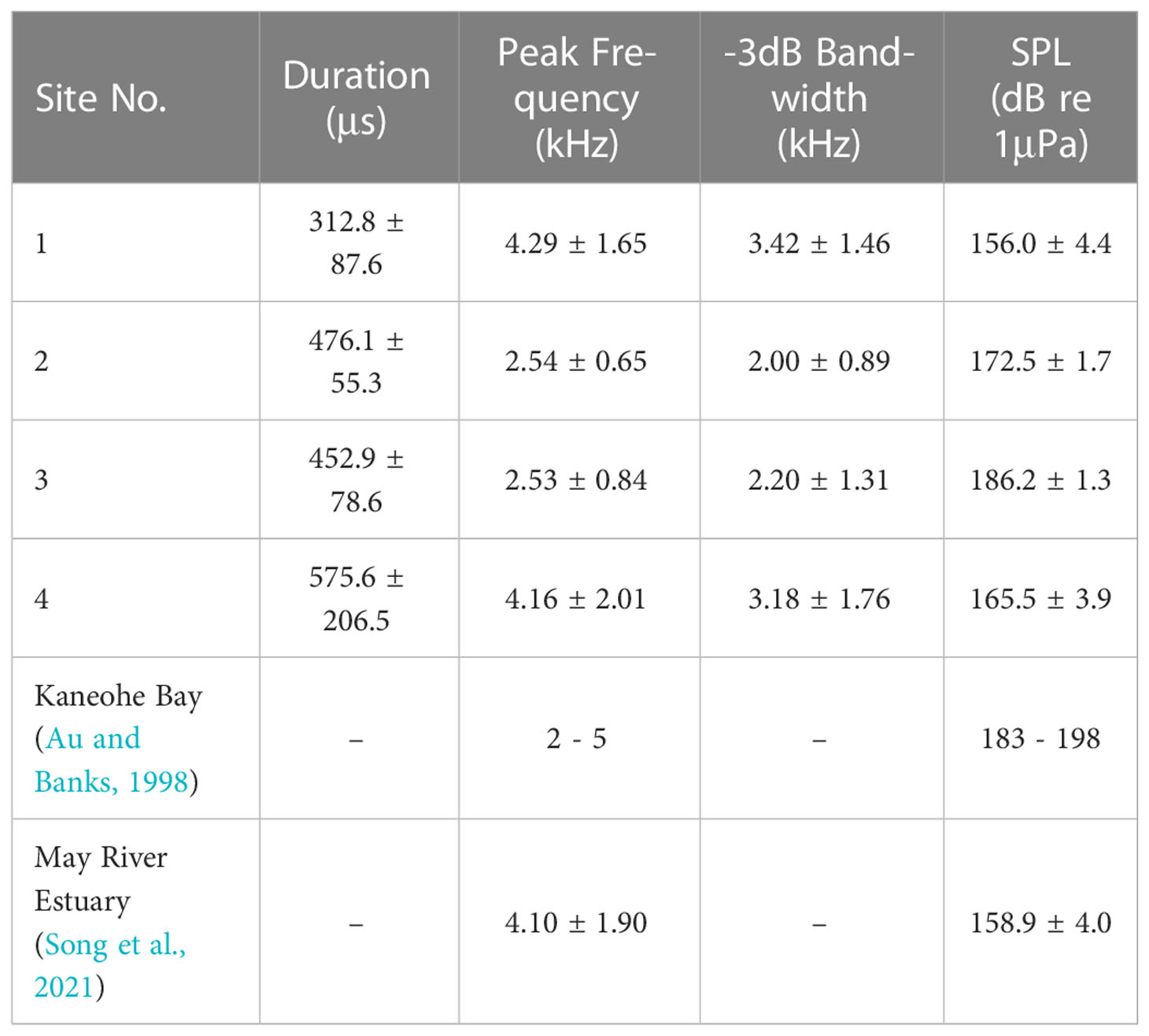
Table 1 Acoustic parameters of snaps recorded at different sites, compared to those reported in previous studies.
3.2 Temporal pattern of snap rate
The temporal variation of the snap rate extracted from site 3 was shown for a series of 7 days and the dots represented the number of snaps per minute, which was smoothed out using a 120-point moving average filter and demonstrated in a black line (Figure 5). The snap rate presented a periodical pattern and ranged from 773 to 3875 snaps per minute, with a mean value of 2935.0, much higher than a previous study using a same thresholding method (Lee et al., 2021). The periodicity of the snap rate was reflected in the smoothed line, showing at least 2 distinct peaks within a day cycle, corresponding to the two high tides with a single day. The difference of snap rate between peak and valley can reach approximately 1000 within a day. The time of the peaks changed with the tidal level. On May 19, the first snap rate peaked at about 2:00 am, which emerged approximately an hour before the first high tide. On May 25, the first snap rate peak moved to around 5:00 am, which was behind the first high tide by almost 3 hours. These results demonstrated that snapping behavior was related to the tide. The first snap rate peak occurred as the tide was rising to reach high level (Peak 1 in Figure 5B). Peak 2 was associated with the high tidal level at night. Valley of snap rate consistently appeared during the daytime in current dataset, which occurred during the low tide periods. The number of the valleys changed during the recording period. At least two valleys were found in 5 of the 7 days during the daytime, including D19, D20, D21, D22, and D25, while only a single and significant valley was observed in D23 and D24. The valleys emerged between the two high tides and at least one valley was close to the low tide at daytime.
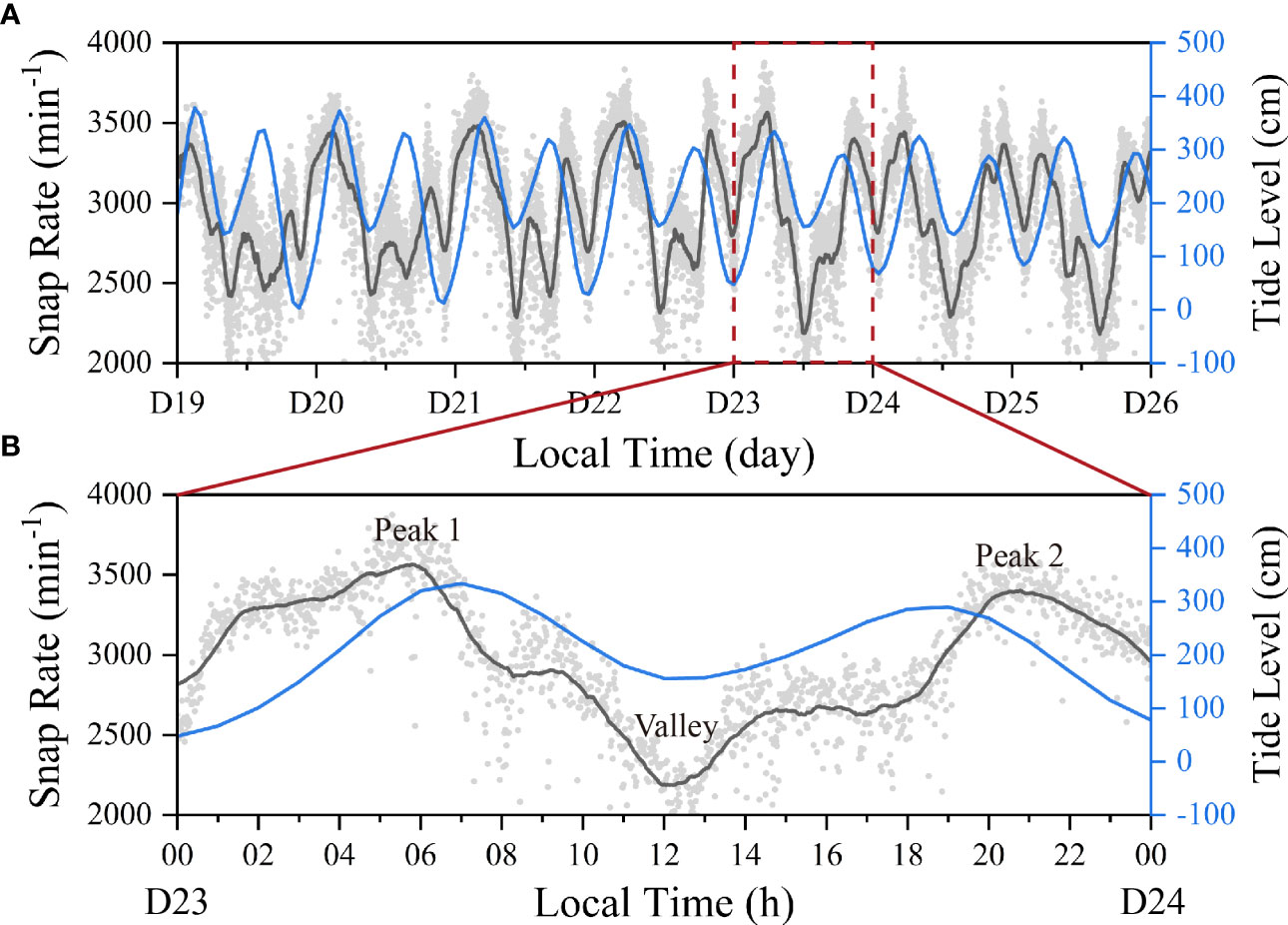
Figure 5 (A) Temporal variation of the snap rate extracted from Site 2 over a 7-day period (D 19–D 26) and the change of tidal level. (B) An enlarged layout of the snap rate within a single recording day.
The snap rate of peak 1 which corresponds to the first high tide was unanimously than that of peak 2 (corresponding to the second-high tide) for each day during the recording period. The difference of the two snap rate during the high tide periods ranged from 17 to 420. The first snap rate peak (peak 1) occurred before the first high tide during the recordings while in comparison the times of valley and peak 2 were behind the low tide and second high tide in 5 of the 7 recording days (Table 2).
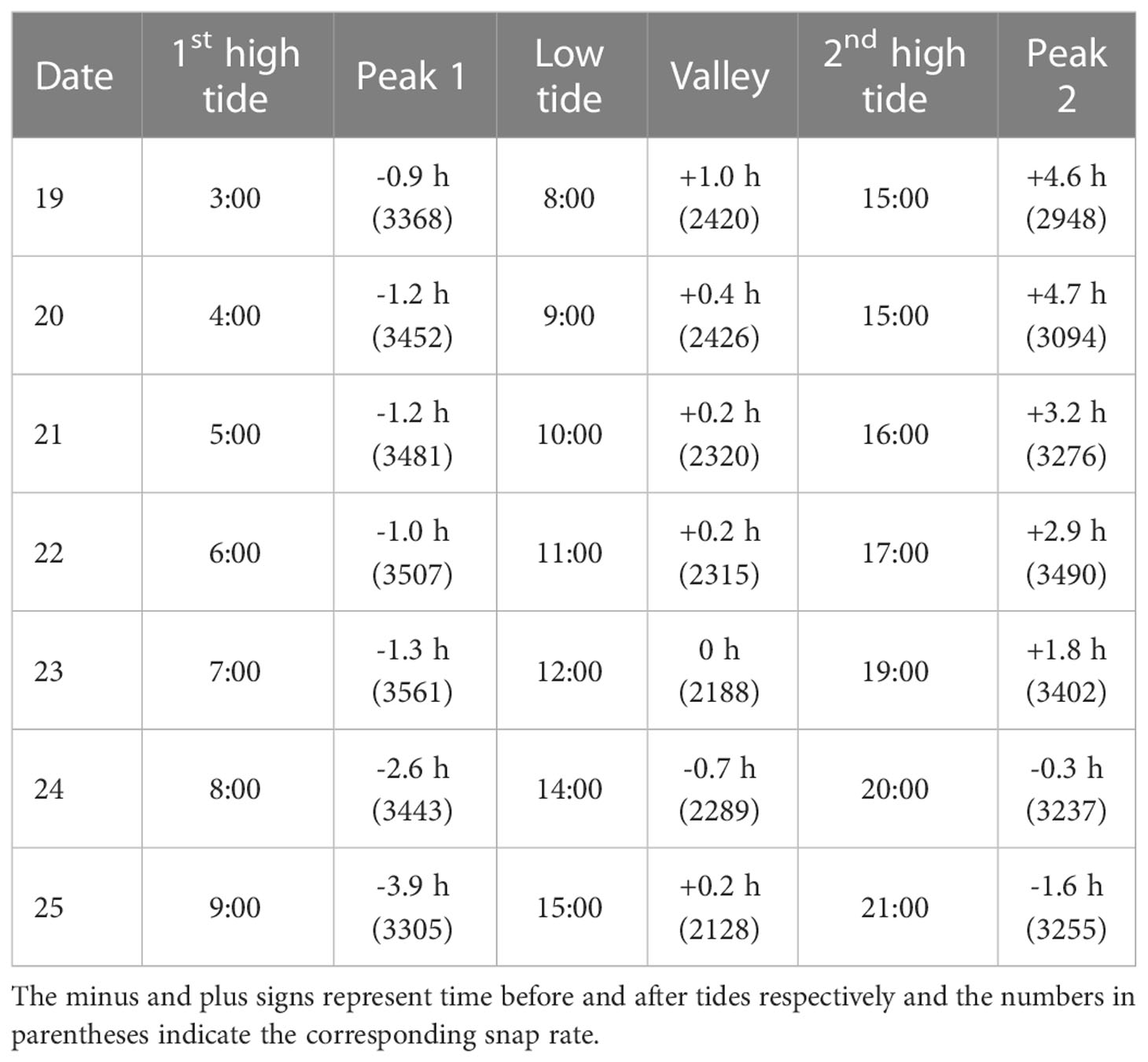
Table 2 The time of high and low tides during the 7-day recording period (D19-D25) at side 2, where the times of the snap rate peaks were tracked using the difference between times of the snap peak and high/low tides.
The snapping shrimp sounds were important contributions to the overall soundscape (Figure 6), accounting for a great proportion across the days, which can be seen in the power spectral density of bandwidth II between 1.5 and 10 kHz. This bandwidth was considered to estimate the soundscape input from snapping shrimp because the peak frequency was found in this range. For bandwidth I (0-1.5 kHz), the mean power spectral density was much lower during the night than daytime with peak-to-peak difference reaching approximately 10 dB, which was thought to be attributed to the commuting boat travels during daytime. The acoustic energy confined in frequency range from 10 to 48 kHz was the lowest (BW II). This proportion might be solely from snaps produced by shrimp as no other sound sources were found related to this part. Power spectral density across the whole recording band (up to 48 kHz) at site 3 fluctuated between 65.3 and 69.3 dB (BW IV).
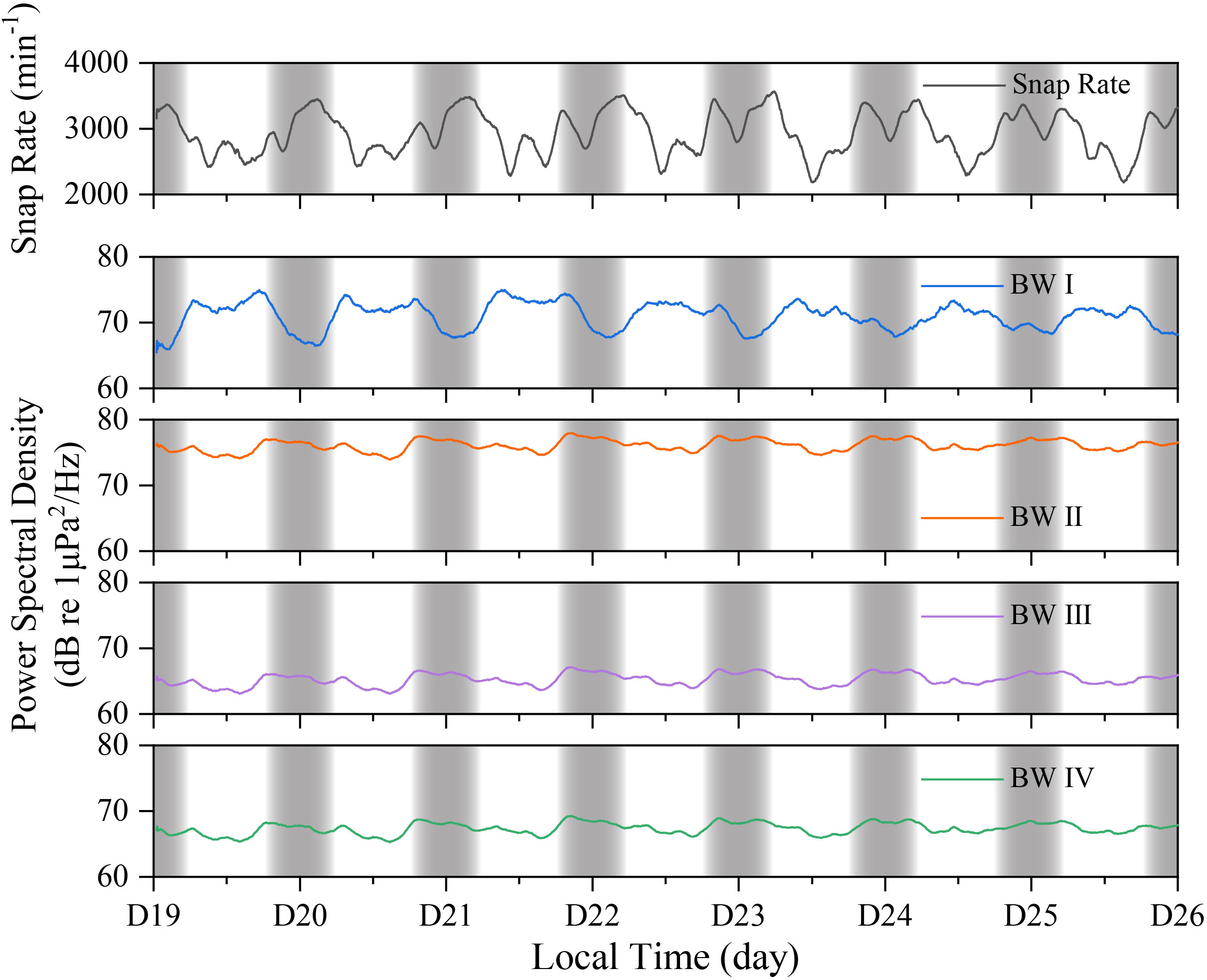
Figure 6 Relation between snap rate and the corresponding soundscape components at different frequency bandwidths, where BW I, BW II, BW III, and BW IV represent the mean power spectral densities (PSD) for frequency ranges of 0-1.5 kHz, 1.5-10 kHz, 10 kHz -48 kHz and 0-48 kHz. The PSD was calculated over a 2-minute window and their temporal variations were given as well. Gray bar areas define nighttime periods.
4 Discussion and summary
Snapping shrimp, as a dominant source of ambient noise in shallow coastal waters, produce a strong pulse of a wide bandwidth. Several studies conducted on the acoustics of snapping shrimp have covered the topics on the temporal pattern of snap rate (Bohnenstiehl et al., 2016; Lee et al., 2021) and acoustic characteristics of snapping shrimp snaps (Au and Banks, 1998; Song et al., 2021; Spiga, 2022). The recordings in current paper provided additional data of the acoustics of shrimp snaps. However, the determinations on shrimp species and number of shrimp species were not yet achieved, limiting the comparison of snap characteristics to site-level instead of species-level. Significant differences were found in duration, peak frequency, -3 dB bandwidth and sound pressure level across the sites (p<0.001). The great ranges of sound pressure level of snaps recorded at different sites may be intrinsic to the animals. Song et al. (2021) found that snaps produced by shrimp can be significantly among different individuals for A. heterochaelis and A. angulosus. The individual variation may be the result of physiological processes, such as individual fitness. The number of species and individuals, as well as size of the shrimp can potentially influence the acoustic properties of the snaps in site-level. Though much remains to be done, the results present here show that shrimp produced snaps as loud as 190 dB and these sound pressure levels were underestimated values of respective source level if snaps had propagated a certain range before reaching the recording hydrophone. Peak frequencies were consistently below 10 kHz (Figure 4), similar to those of snaps recorded in laboratory conditions and field data in May River Estuary (Song et al., 2021). Among the papers on snapping shrimp acoustics, only one paper found the peak frequency of snaps can occasionally reach over 10 kHz and below 1 kHz, which was considered as response to the impulsive stimuli (Spiga, 2022).
Shrimp responded to light as well, reflected in the diurnal pattern of snap rate. Researchers found that snapping shrimp noise levels measured at nighttime were higher than those at daytime by 3–6 dB at Yacht Harbor in San Diego (Johnson et al., 1947; Everest et al., 1948), and by nearly 4 dB on Oahu, Hawaii (Lammers et al., 2008). A sharp increase in snapping abundance both at sunrise and sunset, raising the sound pressure level compared to daytime snaps, showing a potential relationship between light and snapping behaviors (Lammers et al., 2008). The number of snaps produced was correlated with season as well (Bohnenstiehl et al., 2016). Our current dataset had a limited recording length but based on the data available, we can interpret more snaps were recorded at night than during the daytime (Figures 5 and 6).
Besides, snap rate changed with tidal level. There was more than one snap rate peak across a single day and the peak location slightly shifted day by day, which may be attributed to the daily shift of tidal level. Snap rates at peak 1 and 2, which occurred at night, were consistently higher than those calculated for valley occurring during midday (Figure 5). Taking Day 23 as an example, the snap rates of peak 1 and peak 2 were 3561 and 3402, much higher than that of 2188 found in the valley. The snapping events came to its first crest at the earlier hours of the day before the emergence of the first high tide. The second snap rate peak was mostly happening after the second-high tide and slightly lower than that of the first snap rate peak. These two peaks may switch as the moon phase changes in the long term, which needs more data to address. The numbers of the valley and peak were changing across the seven days, which is probably influenced jointly by tidal level and light. Lee et al. (2021) found that the significant correlation between snap rate and tidal level may be rooted in the change of current speed during tide fluctuation. Though the conclusions were based on data recorded in the water column at least 24 m deep and 100 km away from a local island, their results can still be representative for the snapping shrimp living in the benthic area. Jeong and Paeng (2022) used the 90 days’ recording and find snap rate was higher at high tide and lower at low tide, showing a 13% variation. Using the long term monitoring and data analysis, they observed a complex pattern in snap rate, with a diurnal component dominating over the semi-diurnal component. The 7 consecutive days’ recording in current paper was too short to drive any conclusion on tidal impact. Snap rate was the highest on D23, of which the high tide was not the greatest among the recording period. When comparing the two snap rate peaks (Peak 1 and Peak 2) corresponding to the two high tides within a single day, we found that the first snap rate peak (Peak 1) corresponding to the higher high tide was unanimously greater than that of the lower high tide (Table 2). These data altogether seem to show a positive relationship between snap rate and tidal level.
It remained to be investigated what drives the change of snap rate within a single day. There might be a possibility that shrimp produced more snaps to communicate when visual conditions are poor at night. Dinh and Radford (2021) found the snapping shrimp (A. richardsoni) was sensitive to low frequency sounds. There stands a possibility that snapping shrimp achieve communication through their snaps because there is a considerable proportion of energy spreading into the low frequency range (Figure 3). The snaps examined from four sites had significant energy below 1 kHz, overlapped with the tested audible frequency range of A. richardsoni. Supposing the snapping shrimp hear in the same way as A. richardsoni does (Dinh and Radford, 2021), we can hypothesize that shrimp can acoustically detect snaps if the sound pressure level exceeds hearing threshold. We turned to the audiogram of A. richardsoni and compared the amplitudes of hearing threshold and snaps. Shrimp present auditory responses to sounds up to 1500 Hz, at which the hearing threshold was approximately 125 dB (re 1 μPa). The lowest hearing threshold was approximately 90 dB (re 1 μPa) at 80 Hz. We calculated the spectrum of snaps using described in sound pressure level and found that snaps recorded at all sites unanimously have a higher mean sound pressure level than hearing threshold below 1 kHz. The hearing threshold increases to almost 125 dB at 1.5 kHz, surpassing the sound pressure level of snaps at site 1 and site 4. This indicates individuals of snapping shrimp at four sites may be able to acoustically sense the snaps produced by conspecifics if the shrimp in the field have a same hearing threshold to A. richardsoni.
We followed a previous study and used snaps with peak amplitude exceeding four times the root-mean-square amplitude of the received signal to estimate snap rate (Lee et al., 2021). There was no doubt that more snaps can be detected using a smaller amplitude threshold but this would increase the probability of false detection. Snaps of smaller amplitude are probably from a greater distance. Using a same thresholding method, the snap rate in shallow water of southeast China coastal area (current paper) was much higher than that in the East China Sea (Lee et al., 2021). Lee et al. (2021) placed their recording hydrophones in the water column with depth between ∼24 and ∼80 m and the recording site was 100 km away from a nearby island. Our recordings were confined to the coastal region, close to local tidal zones where the shrimps inhabited, and this might increase the snap rate calculation due to the relatively lower propagation attenuation.
We conducted field experiments at four sites in southeast China coastal area to record the broadband pulses produced by snapping shrimp. Snaps dominated the overall underwater soundscape. Research was extended geographically into the Southeast China coastal area, providing data for the snapping shrimp in this region for the first time. The characteristics of snaps were significantly different across the recording sites. Snap rate examined in one site with 7 consecutive days of recording showed a diurnal pattern and snap rate had a correlation with the tidal level, indicating that the snap rate corresponded to the tidal level periodically. A high tide was accompanied with a local high snap rate regardless of light and this local snap rate peak was much higher at night. Data of a single site made it impossible to compare the snap rate across different sites. The long-term monitoring is important to probe into the monthly or seasonal snapping behavior and its coincidence with ocean environmental factors such as temperature, water depth and light performed in previous studies.
Data availability statement
The raw data supporting the conclusions of this article will be made available by the authors, without undue reservation.
Ethics statement
Ethical review and approval was not required for the animal study because Ethical review and approval were not required for this study because there is not an ethically questionable study. Experiments were conducted in the field where animals were in their natural conditions.
Author contributions
ZS, WO, YS, and YZ: primary writing. ZS and YZ: synthesis and overall coordination. ZS, WO, YS, HL, XX, and WF: experiment design and data collection. ZS, WO, and YS: acoustic data analysis. WO, YS, XX, HL, and WF: ocean environment data analysis. ZS, WO, XX, WF, TW and YZ: paper revision and re-editing. All authors contributed to the article and approved the submitted version.
Funding
This work was financially supported by the Project supported by the National Natural Science Foundation of China (Grant Nos. 42106181; 12074323), the China National Postdoctoral Program for Innovative Talents (Grant No. BX2021168), the China Postdoctoral Science Foundation (Grant No. 2020M682086), the Outstanding Postdoctoral Scholarship and the PhD Fellowship of State Key Laboratory of Marine Environmental Science at Xiamen University, and the Science, Technology Major Project of Fujian Province (Grant Nos. 2021NZ033016), the Water Conservancy Science and Technology Innovation Project of Guangdong (2020-16), the Guangzhou Science and Technology Foundation (No. 202102020901) and the Financial Fund of the Ministry of Agriculture and Rural Affairs, P.R. China (No.NFZX2021).
Acknowledgments
The authors are grateful to Zhen Xiao from College of Ocean and Earth Sciences, Xiamen University, for his assistance in conducting this study.
Conflict of interest
The authors declare that the research was conducted in the absence of any commercial or financial relationships that could be construed as a potential conflict of interest.
Publisher’s note
All claims expressed in this article are solely those of the authors and do not necessarily represent those of their affiliated organizations, or those of the publisher, the editors and the reviewers. Any product that may be evaluated in this article, or claim that may be made by its manufacturer, is not guaranteed or endorsed by the publisher.
References
Au W. W. L., Banks K. (1998). The acoustics of the snapping shrimp synalpheus parneomeris in kaneohe bay. J. Acoust. Soc Am. 103, 41–47. doi: 10.1121/1.423234
Au W. W. L., Martin S. W., Moore P. W., Branstetter B., Copeland A. M. (2016). Dynamics of biosonar signals in free-swimming and stationary dolphins: the role of source levels on the characteristics of the signals. J. Acoust. Soc Am. 139 (3), 1381–1389. doi: 10.1121/1.4944636
Bibikov N. G., Makushevich I. V. (2020). Structure of the snapping shrimps’ acoustical activity in the black Sea shallow water. J. Acoust. Soc Am. 148 (4), EL389–EL393. doi: 10.1121/10.0002260
Bohnenstiehl D. R., Lillis A., Eggleston D. B. (2016). The curious acoustic behavior of estuarine snapping shrimp: Temporal patterns of snapping shrimp sound in sub-tidal oyster reef habitat. PloS One 11, e0143691. doi: 10.1371/journal.pone.0143691
Butler J., Butler M. J. IV, Gaff H. (2017). Snap, crackle, and pop: Acoustic-based model estimation of snapping shrimp populations in healthy and degraded hard-bottom habitats. Ecol. Indic. 77, 377–385. doi: 10.1016/j.ecolind.2017.02.041
Dinh J. P., Radford C. (2021). Acoustic particle motion detection in the snapping shrimp (Alpheus richardsoni). J. Comp. Physiol. A. 207, 641–655. doi: 10.1007/s00359-021-01503-4
Duarte C. M., Chapuis L., Collin S. P., Costa D. P., Devassy R. P., Eguiluz V. M., et al. (2021). The soundscape of the anthropocene ocean. Science 371, eaba4658. doi: 10.1126/science.aba4658
Erbe C., Parsons M., Duncan A., Osterrieder S. K., Allen K. (2017). Aerial and underwater sound of unmanned aerial vehicles (UAV). J. Unmanned. Veh. Syst. 5, 92–101. doi: 10.1139/juvs-2016-0018
Everest F. A., Young R. W., Johnson M. W. (1948). Acoustical characteristics of noise produced by snapping shrimp. J. Acoust. Soc Am. 20, 137–142. doi: 10.1121/1.1906355
Fay R. (2009). Soundscapes and the sense of hearing of fishes. Integr. Zool. 4, 26–32. doi: 10.1111/j.1749-4877.2008.00132.x
Giorli G., Au W. W. L., Neuheimer A. (2016). Differences in foraging activity of deep sea diving odontocetes in the ligurian Sea as determined by passive acoustic recorders. Deep. Sea. Res. PT. I. 107, 1–8. doi: 10.1016/j.dsr.2015.10.002
Harding H. R., Gordon T. A. C., Eastcott E., Simpson S. D., Radford A. N. (2019). Causes and consequences of intraspecific variation in animal responses to anthropogenic noise. Behav. Ecol. 30 (6), 1501–1511. doi: 10.1093/beheco/arz114
Hawkins A. D., Amorim M. C. P. (2000). Spawning sounds of the male haddock, Melanogrammus aeglefinus. Envir. Biol. Fish. 59, 29–41. doi: 10.1023/A:1007615517287
Herberholz J., Schmitz B. (1999). Flow visualisation and high speed video analysis of water jets in the snapping shrimp (Alpheus heterochaelis). J. Comp. Physiol. A. 185, 41–49. doi: 10.1007/s003590050364
Herbert-Read J. E., Kremer L., Bruintjes R., Radford A. N., Ioannou C. C. (2017). Anthropogenic noise pollution from pile-driving disrupts the structure and dynamics of fish shoals. P. R. Soc B. 284, 20171627. doi: 10.1098/rspb.2017.1627
Hughes M. (2000). Deception with honest signals: Signal residuals and signal function in snapping shrimp. Behav. Ecol. 11, 614–623. doi: 10.1093/beheco/11.6.614
Jeong I., Paeng D. G. (2022). Circadian and tidal changes in snapping shrimp (Alpheus brevicristatus) sound observed by a moored hydrophone in the coastal Sea of Western jeju. Appl. Sci. 12, 6493. doi: 10.3390/app12136493
Johnson M. W., Everest F. A., Young R. W. (1947). The role of snapping shrimp (Crangon and Synalpheus) in the production of underwater noise in the sea. Biol. Bull. 93, 122–138. doi: 10.2307/1538284
Lammers M. O., Brainard R. E., Au W. W. L., Mooney T. A., Wong K. B. (2008). An ecological acoustic recorder (EAR) for long-term monitoring of biological and anthropogenic sounds on coral reefs and other marine habitats. J. Acoust. Soc Am. 123, 1720–1728. doi: 10.1121/1.2836780
Lee D. H., Choi J. W., Shin S., Song H. C. (2021). Temporal variability in acoustic behavior of snapping shrimp in the East China Sea and its correlation with ocean environments. Front. Mar. Sci. 8. doi: 10.3389/fmars.2021.779283
Lillis A., Mooney T. A. (2018). Snapping shrimp sound production patterns on Caribbean coral reefs: Relationships with celestial cycles and environmental variables. Coral. Reefs. 37, 597–607. doi: 10.1007/s00338-018-1684-z
Madsen P. T., Wahlberg M. (2007). Recording and quantification of ultrasonic echolocation clicks from free-ranging toothed whales. Deep. Sea. Res. PT. I. 54, 1421–1444. doi: 10.1016/j.dsr.2007.04.020
Mann D. A., Lobel P. S. (1997). Propagation of damselfish (Pomacentridae) courtship sounds. J. Acoust. Soc Am. 101, 3783–3791. doi: 10.1121/1.418425
Mathias D., Gervaise C., Iorio L. D. (2016). Wind dependence of ambient noise in a biologically rich coastal area. J. Acoust. Soc Am. 139, 839–850. doi: 10.1121/1.4941917
McClure M. R., Wicksten M. K. (1997). Morphological variation of species of the edwardsii group of alpheus in the northern gulf of Mexico and northwestern Atlantic (Decapoda: Caridea: Alpheidae). J. Crustacean. Biol. 17, 480–487. doi: 10.2307/1549442
McDonald M. A., Hildebrand J. A., Wiggins S. M. (2006). Increases in deep ocean ambient noise in the northeast pacific west of San Nicholas island, California. J. Acoust. Soc Am. 120, 711–718. doi: 10.1121/1.2216565
Monczak A., Berry A., Kehrer C., Montie E. W. (2017). Long-term acoustic monitoring of fish calling provides baseline estimates of reproductive timelines in the may river estuary, southeastern USA. Mar. Ecol. Prog. Ser. 581, 1–19. doi: 10.3354/meps12322
Monczak A., Mueller C., Miller M. E., Ji Y., Borgianini S. A., Montie E. W. (2019). Sound patterns of snapping shrimp, fish, and dolphins in an estuarine soundscape of the southeastern USA. Mar. Eco. Prog. Ser. 609, 49–68. doi: 10.3354/meps12813
Mooney T. A., Mathias H. A., Stanley J. (2020). Acoustic impacts of offshore wind energy on fishery resources: An evolving source and varied effects across a wind farm’s lifetime. Oceanography 33 (4), 83–95. doi: 10.5670/oceanog.2020.408
Mueller C., Monczak A., Soueidan J., McKinney B., Smott S., Mills T., et al. (2020). Sound characterization and fine-scale spatial mapping of an estuarine soundscape in the southeastern USA. Mar. Ecol. Prog. Ser. 645, 1–23. doi: 10.3354/meps13373
Nolan B. A., Salmon M. (1970). The behavior and ecology of snapping shrimp (Crustacea: Alpheus heterochaelis and alpheus normanni). Forma. Functio. 2, 289–335.
Radford C., Jeffs A., Tindle C., Montgomery J. (2008). Temporal patterns in ambient noise of biological origin from a shallow water temperate reef. Oecologia 156, 921–929. doi: 10.1007/s00442-008-1041-y
Schein H. (1975). Aspects of the aggressive and sexual behaviour of alpheus heterochaelis say. Mar. Behav. Physiol. 3, 83–96. doi: 10.1080/10236247509378498
Schein H. (1977). The role of snapping in alpheus heterochaelis say,1818, the big-clawed snapping shrimp. Crustaceana 33, 182–188. doi: 10.1163/156854077X00089
Schlitzer R. (2021) Ocean data view. Available at: https://odv.awi.de.
Schmitz B. (2002). “Sound production in crustacea with special reference to the alpheidae,” in The crustacean nervous system. Ed. Wiese K. (Berlin: Springer), 536–547. doi: 10.1007/978-3-662-04843-6_40
Schmitz B., Herberholz J. (1998). Snapping behaviour in intraspecific agonistic encounters in the snapping shrimp (Alpheus heterochaelis). J. Biosci. 23, 623–632. doi: 10.1007/BF02709175
Slabbekoorn H., Bouton N., Opzeeland I., Coers A., Cate C., Popper A. N. (2010). A noisy spring: the impact of globally rising underwater sound levels on fish. Trends Ecol. Evol. 25, 419–427. doi: 10.1016/j.tree.2010.04.005
Song Z., Salas A. K., Montie E. W., Laferriere A., Zhang Y., Aran Mooney T. (2021). Sound pressure and particle motion components of the snaps produced by two snapping shrimp species (Alpheus heterochaelis and Alpheus angulosus). J. Acoust. Soc Am. 150, 3288–3301. doi: 10.1121/10.0006973
Spiga I. (2022). The acoustic response of snapping shrimp to synthetic impulsive acoustic stimuli between 50 and 600 Hz. Mar. pollut. Bull. 185 (2022), 114238. doi: 10.1016/j.marpolbul.2022.114238
Toth E., Duffy J. E. (2005). Coordinated group response to nest intruders in social shrimp. Biol. Lett. 1, 49–52. doi: 10.1098/rsbl.2004.0237
Van Oosterom L., Montgomery J. C., Jeffs A. G., Radford C. A. (2016). Evidence for contact calls in fish: conspecific vocalisations and ambient soundscape influence group cohesion in a nocturnal species. Sci. Rep. 6, 19098. doi: 10.1038/srep19098
Versluis M., Schmitz B., von der Heydt A., Lohse D. (2000). How snapping shrimp snap: Through cavitating bubbles. Science 289, 2114–2117. doi: 10.1126/science.289.5487.211
Watanabe M., Sekine M., Hamada E., Ukita M., Imai T. (2002). Monitoring of shallow sea environment by using snapping shrimps. Water. Sci. Technol. 46, 419–424. doi: 10.2166/wst.2002.0772
Keywords: snapping shrimp, west-Pacific Ocean, bioacoustics, animal behavior, coastal water
Citation: Song Z, Ou W, Su Y, Li H, Fan W, Sun S, Wang T, Xu X and Zhang Y (2023) Sounds of snapping shrimp (Alpheidae) as important input to the soundscape in the southeast China coastal sea. Front. Mar. Sci. 10:1029003. doi: 10.3389/fmars.2023.1029003
Received: 26 August 2022; Accepted: 05 January 2023;
Published: 30 January 2023.
Edited by:
Marta Solé, BarcelonaTech (UPC), SpainReviewed by:
Carrie Wall, University of Colorado Boulder, United StatesDonatas Bagočius, Aplinkos Apsaugos Agentura, Lithuania
Copyright © 2023 Song, Ou, Su, Li, Fan, Sun, Wang, Xu and Zhang. This is an open-access article distributed under the terms of the Creative Commons Attribution License (CC BY). The use, distribution or reproduction in other forums is permitted, provided the original author(s) and the copyright owner(s) are credited and that the original publication in this journal is cited, in accordance with accepted academic practice. No use, distribution or reproduction is permitted which does not comply with these terms.
*Correspondence: Yu Zhang, yuzhang@xmu.edu.cn
†These authors have contributed equally to this work