- 1School of Medical and Life Sciences, Chengdu University of Traditional Chinese Medicine, Chengdu, China
- 2School of Medical Technology and Engineering, Fujian Medical University, Fuzhou, China
- 3Institute of Translational Pharmacology and Clinical Application, Sichuan Academy of Chinese Medical Sciences, Chengdu, China
Colorectal cancer (CRC) ranks third in terms of global incidence and second in terms of death toll among malignant tumors. Gut microbiota are involved in the formation, development, and responses to different treatments of CRC. Under normal physiological conditions, intestinal microorganisms protect the intestinal mucosa, resist pathogen invasion, and regulate the proliferation of intestinal mucosal cells via a barrier effect and inhibition of DNA damage. The composition of gut microbiota and the influences of diet, drugs, and gender on the composition of the intestinal flora are important factors in the early detection of CRC and prediction of the results of CRC treatment. Regulation of gut microbiota is one of the most promising new strategies for CRC treatment, and it is essential to clarify the effect of gut microbiota on CRC and its possible mechanisms to facilitate the prevention and treatment of CRC. This review discusses the role of gut microbiota in the pathogenesis of CRC, the potential of gut microbiota as biomarkers for CRC, and therapeutic approaches to CRC based on the regulation of gut microbiota. It might provide new ideas for the use of gut microbiota in the prevention and treatment of CRC in the near future and thus reduce the incidence of CRC.
Introduction
Colorectal cancer (CRC) is a malignant tumor occurring in the colon or rectum in the lower gastrointestinal tract. According to data from a 2020 survey by the World Health Organization, CRC ranks third and second in terms of incidence and mortality among all cancers and third and second in terms of incidence among men and women, respectively (Sung et al., 2021). The latest data show that the number of new cases of CRC in the world is as high as 193 million in 2020, and it is expected that the number of new cases of CRC in the world will increase to 2.5 million by 2035, surpassing common cancers such as liver cancer and gastric cancer (Sung et al., 2021).
The evolution of CRC is a complex multistep process. With the continuous developments and changes in people’s lifestyles toward Western lifestyles, the incidence and mortality rates of CRC are rising (Chen W. et al., 2016), which seriously threatens human health. The pathogenesis of CRC is complex and is due to synergistic effects of multiple factors. Its risk of occurrence is not only associated with mutations in multiple genes but also depends on the immune status of the patient, which is positively correlated with the intestinal flora. In recent years, with the intensification of research on intestinal microorganisms, research on the use of immunotherapy for treating CRC has been widely carried out in clinical settings. Further research on gut microbiota is likely to provide a promising approach for improving the efficacy of existing chemotherapy drugs, reducing their toxicity, and improving the sensitivity of immunotherapy (Gopalakrishnan et al., 2018).
Gut microbiota promote intestinal repair by promoting cell proliferation and differentiation, which are essential for maintaining the integrity of the intestinal barrier (Krishnan et al., 2015). There is growing evidence that the large and complex gut microbiota interact via a complex mechanism with substances in the surrounding microenvironment (Geller et al., 2017). Gut microbiota play a critical role not only in maintaining the homeostasis of the intestinal environment but also in the maturation and sustainable development of the immune system (Thaiss et al., 2016). Various factors such as age, diet, lifestyle, and genotype act synergistically to cause changes in the structure and abundance of intestinal microbial populations (Seesaha et al., 2020). Imbalances in gut microbiota and disorders of flora population structure such as reductions in populations of beneficial bacteria and the presence of Clostridium, Enterobacter, and other pathogenic bacteria result in microecological imbalances and thereby disrupt cell DNA, activate carcinogenic signaling pathways, and ultimately affect the development of CRC (Brennan and Garrett, 2016; Figure 1).
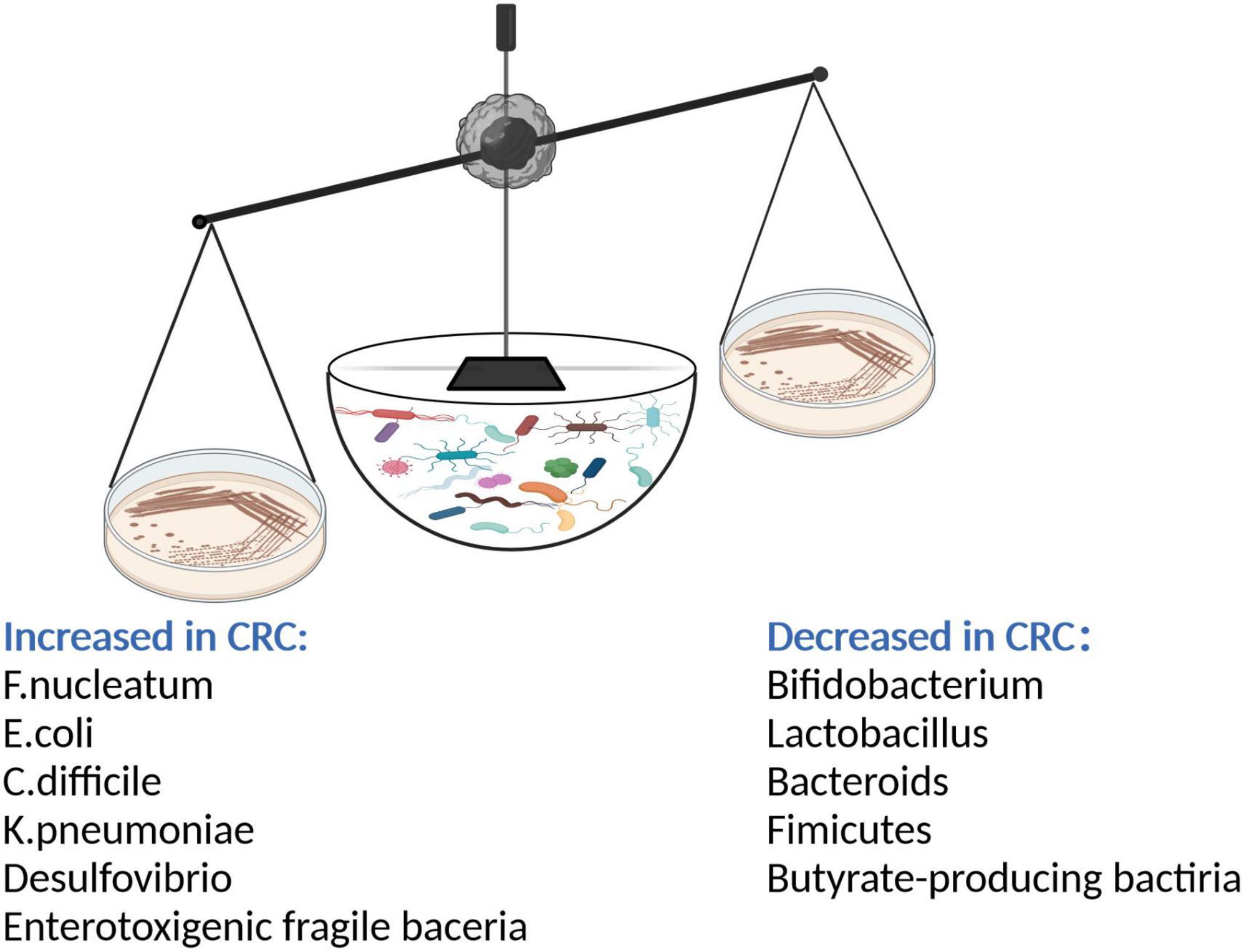
Figure 1. After gut microbiota imbalance, intestinal probiotics such as Bifidobacterium, Lactobacillus, Bacteroides, butyrate-producing bacteria decreased; the number of pathogenic bacteria such as Fusobacterium nucleatum, Enterotoxigenic fragile bacteria, Escherichia coli, Clostridium difficile, Klebsiella pneumonia, Desulphurization increased. The pathogenic bacteria secreted a large number of toxic factors to damage intestinal epithelial cells and promote the occurrence of CRC.
In recent years, fecal microbiota transplantation has improved the benefits of treatment for patients with many diseases (Lu et al., 2019). Fecal microbiota transplantation involves the transfer of fecal microbiota from a healthy donor to the patient’s intestine to correct bacterial imbalances and restore intestinal homeostasis. By reconstructing the intestinal flora, it has an antitumor immune effect. The mechanisms by which the intestinal flora affects carcinogenesis, inflammation, and immune and therapeutic responses at the local level have been revealed in existing studies (Kim et al., 2020; Wang and Li, 2022). However, the specific mechanisms whereby microorganisms at the distal intestinal epithelial barrier regulate the occurrence of CRC, immunity, and physiological functions of multiple organs are not clear.
Although methods for “accurately” regulating intestinal microflora are still in their infancy, there is a lack of ideal screening methods, and there have been few studies on treatment techniques using intestinal microflora, there is no doubt that the regulation of intestinal microflora will be more widely used for overall health improvements and adjuvant therapy (Liu et al., 2018). Therefore, in the era of precision medicine, it is still necessary to promote research on techniques for the steady-state reconstruction of intestinal microflora to further clarify the relationship between intestinal microflora and CRC. Personalized treatment tailored according to the genetic background of the patient and the individual intestinal microflora promises to reduce the risk of CRC and improve patient prognosis, which will have a wide range of positive effects on public health.
Interactions between intestinal flora and colorectal cancer
There are abundant microbial communities in the human body, which are mainly concentrated in the nose, mouth, skin, and intestines, especially the intestines. The total number of intestinal microorganisms may reach 100 trillion. The intestinal flora is an increasingly important factor in human digestion, metabolism, immunity, and other processes (Valdes et al., 2018), and it is therefore referred to as another “organ” of the human body. The intestinal flora can regulate immune function, synthesize vitamins, maintain the intestinal barrier, and promote the metabolism and absorption of materials. The pathogenesis of CRC is a multistep and multifactor process (Dahmus et al., 2018). It is therefore necessary to elucidate the mutual effects of the intestinal flora and gastrointestinal tract and their roles in the pathogenesis of CRC and to provide potential therapeutic targets and new ideas for the prevention and treatment of CRC.
The intestinal flora is divided into probiotics, conditionally pathogenic bacteria, and pathogenic bacteria. Probiotics, such as Bifidobacterium and Lactobacillus, widely exist in common fermented milk and have various beneficial effects on health (Sokol et al., 2020). Probiotics are mostly specific anaerobic bacteria and are dominant in the intestinal flora. Conditional pathogens are mainly facultative anaerobic bacteria, such as Enterobacter and Enterococcus, whereas pathogenic bacteria include Salmonella, Proteus, and pathogenic Escherichia coli. The dominant intestinal flora at the phylum level mainly comprises Firmicutes, Bacteroidetes, and Actinobacteria (Chen J. et al., 2022; Mohammadi et al., 2022). The normal intestinal flora plays a significant role in the homeostasis of the intestinal environment, including participating in the protection, structure formation, and metabolism of the intestinal epithelium. Imbalances in the intestinal flora can change the intestinal microenvironment, including changes in intestinal epithelial genes, the occurrence of inflammatory reactions, the production of toxic metabolites, and damage to the intestinal epithelial barrier (Gagniere et al., 2016). All these changes are potential pathogenic factors with regard to CRC (Dinan and Cryan, 2017). For example, the intestinal flora plays a crucial role in the development of CRC by inducing abnormal immune responses in colon tissue to weaken the intestinal epithelial barrier and form a specific immune microenvironment (Si et al., 2021; Figure 2).
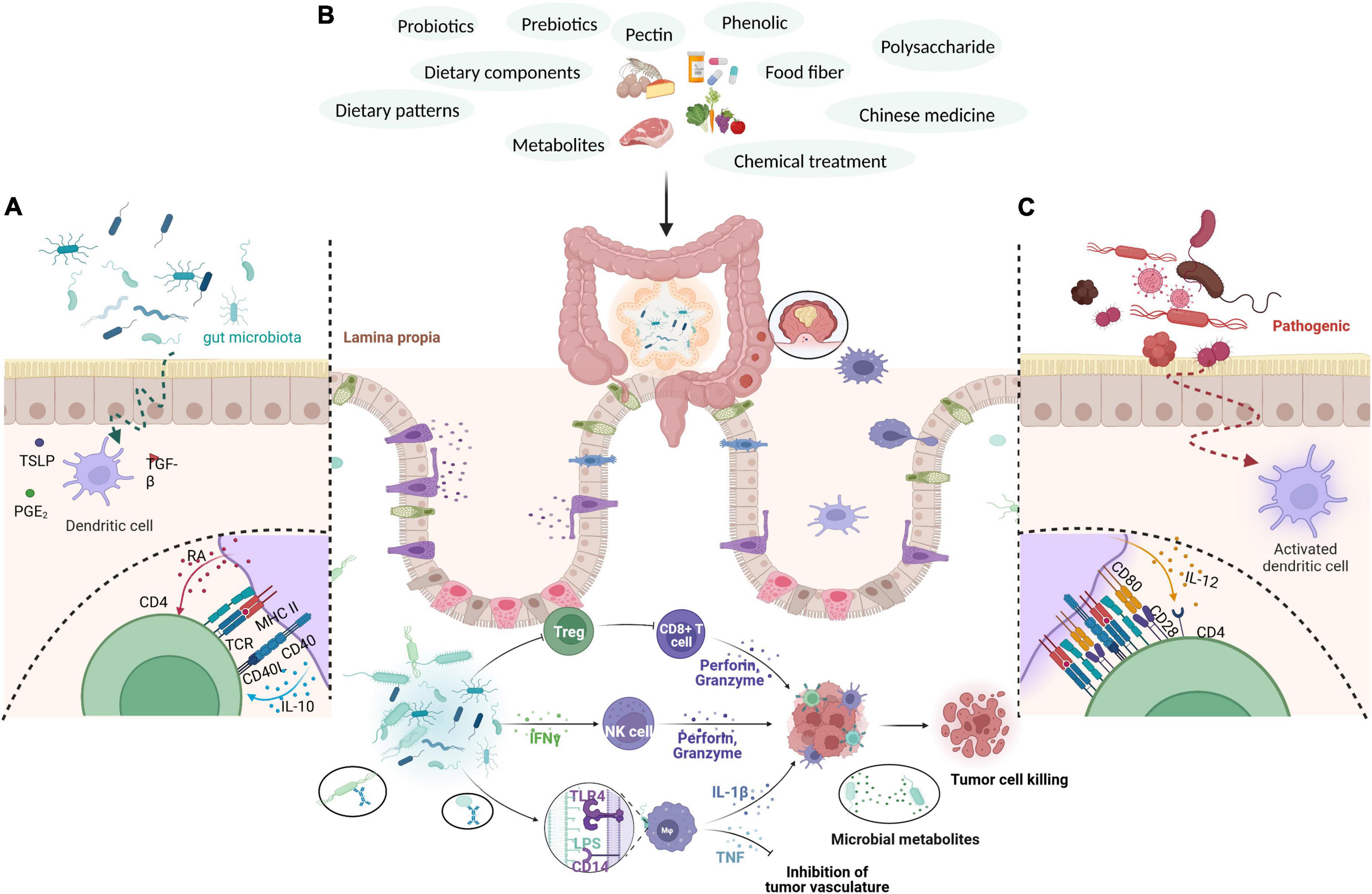
Figure 2. Through external intervention measures, such as the use of probiotics, prebiotics, polysaccharides, pectin, polyphenols, plant fibers, bacterial metabolites, drugs, and diet, the richness of intestinal flora is increased, intestinal immunity is regulated, the immune response of the body is enhanced, the secretion of anti-cancer cytokines is increased, and the colorectal mucosa is protected, thereby effectively intervening in CRC. (A) Gut microbiota produce substances such as PGE2, TGF-β, and TSLP to inhibit the maturation of dendritic cells (DCs). Immature DCs give weak co-stimulatory signals and secrete cytokines such as RA and IL-10 to induce CD4T cells to differentiate into Treg cells. (B) Gut microbiota induces the formation of T cells, secretes INF-γ, and activates NK cells. After contacting the intestinal epithelium, it enhances the phagocytic activity of macrophages through the receptor pathway, promotes cytokines such as TNF and IL, stimulates the body’s immune response, and exerts an anti-tumor effect. (C) Intestinal pathogenic bacteria penetrate the intestinal epithelium to activate DCs. The activated DCs express strong costimulatory ligands and induce CD4T cells to differentiate into effective TH1 and TH17 cells.
Ecological imbalances in the intestine promote the development of CRC, and the microbial community at a lesion site is significantly different from that in the adjacent healthy mucosa. The toxic substances produced by bacterial metabolism during an imbalance in the intestinal flora mainly comprise hydrogen sulfide, reactive oxygen species, active nitrogen species, and bile acid metabolites, which can damage intestinal epithelial cells, reduce the protective barrier effect of intestinal epithelial cells, and induce cell proliferation. Carcinogenic metabolites can also induce DNA damage in intestinal epithelial cells and thus produce mutations and induce the development of CRC (Avuthu and Guda, 2022). Studies have shown that fecal microbiota transplantation can induce polyp formation and change the local immune environment in mice (Sokol et al., 2020). Researchers have attempted to use probiotics to prevent and treat CRC. Different bacterial species play different roles in stimulating inflammation, inducing the release of proinflammatory toxins, increasing the production of reactive oxygen species, changing signaling pathways, and preventing immune function against CRC (Pennisi, 2013). According to research, probiotics, prebiotics, and synbiotics have been developed (Amitay et al., 2020). In addition to the effect of probiotics on treatment response, clinical trials (Gagliani et al., 2014; Panebianco et al., 2018) have examined the effects of probiotics on treatment-related toxicity. Studies have shown that in colon cancer patients the administration of 5-fluorouracil (5-FU) in combination with Lactobacillus rhamnosus can help improve the oral mucosa and alleviate diarrhea (Mego et al., 2013). In a mouse model of colon cancer induced by inflammation, supplementation with probiotics can reduce tumor cell proliferation and the number of tumors, downregulate the activation of nuclear factor (NF)-κB, and promote the activity of probiotics in the intestine (Osterlund et al., 2007). Recent studies in mice with colon cancer have shown that oral intake of probiotic supplements containing Lactobacillus helveticus can reduce the number of interleukin (IL)-17+ T cells and inhibit proliferation and tumor formation, which may be achieved via a change in the intestinal microflora (Osterlund et al., 2007).
In recent years, intestinal microorganisms have been proved to interact with the central nervous system indirectly via the gut–brain axis and thus affect the chemistry and functioning of the central nervous system. The gut–brain axis is a two-way communication system that integrates nerve, hormone, and immune signals between the central nervous system and intestinal tract (Dinan and Cryan, 2017). The intestinal tract can synthesize and secrete various neuroactive substances that can penetrate the blood–brain barrier and affect the brain (Di et al., 2019). Similarly, neuroactive molecules derived from the brain can affect the intestinal tract via the sympathetic and parasympathetic systems or humoral pathways (Petra et al., 2015); examples include substance P, calcitonin gene-related peptide, and neuropeptide Y (Evans et al., 2013). Microorganisms can affect the body’s stress level and behavior by participating in two-way regulatory pathways within the gut-brain axis, including neural signaling pathways, immune responses, the intestinal mucosal barrier, and 5-hydroxytryptamine and tryptophan metabolic pathways, and play a role in tumor growth (Miller et al., 2008; Holzer and Farzi, 2014; Alpert et al., 2021). Bacteria in the intestinal microenvironment can regulate cancer. Probiotics with metabolic activity can change the chemical structures of chemotherapy drugs by increasing or decreasing their activity and thereby changing their effective local concentration. It is increasingly clear that synbiotic microorganisms promote overall immunity (Rong et al., 2019). In addition, evidence suggests that these microorganisms may increase susceptibility to certain cancers by direct effects due to their local existence in the tumor microenvironment or systemic effects via distant microbial communities such as those in the gut and skin (Popa et al., 2022). The latter effects are particularly related to the ability of intestinal microbial regulation to respond to the toxicity of traditional chemotherapy drugs and immunotherapy and may affect the prognosis of patients (Vieira et al., 2013).
Drug therapies for CRC are mainly divided into basic chemotherapy drugs, targeted drugs, biological agents, and traditional Chinese medicinal prescriptions (Zhang et al., 2020). In recent years, numerous studies have been devoted to investigating the intestinal flora and its metabolites associated with the incidence, development, and treatment of CRC in order to better utilize the intestinal flora to prevent and treat CRC. Biological agents, targeted therapies, and traditional Chinese medicinal prescriptions when combined with chemotherapy can achieve ideal curative effects in clinical treatment and provide various treatment methods for CRC patients. Therapeutic strategies targeting the intestinal flora have shown great potential (Sivan et al., 2015). The modulatory role of the intestinal flora in the treatment of CRC includes enhancing the sensitivity of patients to immunotherapy, reducing adverse reactions to chemotherapy drugs, and reducing radiation damage. However, it is worth drawing attention to the fact that the onset of CRC is occult, and public awareness of its symptoms is low. Many patients are already in the late stage of disease when diagnosed, which results in poor therapeutic effects and a poor prognosis. Therefore, screening for CRC should also receive attention.
Regulatory effect of probiotics on intestinal flora in colorectal cancer
The intestinal flora is a complex microbial community symbiotic with the human body and has attracted the attention of an increasing number of researchers over recent years. In this way, the study of probiotics has become more in-depth (Sobhani et al., 2011; Zhu et al., 2014). In recent years, the regulatory role of probiotics in the immune system and their antitumor characteristics have gradually become clear. Probiotics are live microorganisms that are beneficial to the body, of which the most widely used are Lactobacillus, Bifidobacterium, and Clostridium butyricum (Zhong et al., 2014). Bacterial strains may be responsible for detecting and degrading potential carcinogens and producing short-chain fatty acids (SCFAs) that affect cell death and proliferation (Eslami et al., 2019). Probiotic strains reduce the production of anti-inflammatory cytokines or inhibit their expression and thereby prevent the occurrence of CRC (Silveira et al., 2020). Probiotics can additionally activate phagocytosis to eliminate early cancer cells (Gorska et al., 2019). In a comparison of postoperative complications in CRC patients, Bajramagic et al. (2019) discovered that complications occurred more often in patients who did not receive probiotic therapy, especially in cases of intestinal obstruction, which suggests that supplementation with probiotic therapy in patients given surgical treatment for CRC is beneficial. Probiotics are involved in the immune process by stimulating antibody responses and inhibiting monocyte proliferation. In addition, probiotics can increase levels of anti-inflammatory cytokines, such as IL-10 and IL-12, and decrease levels of proinflammatory cytokines, such as IL-1β and IL-6, which have strong anti-inflammatory activities (Gorska et al., 2019). It has been discovered that microecological agents (Bultman, 2016), especially probiotics, can exert antitumor effects by stimulating immune responses, enhancing the phagocytic activity of macrophages, and promoting the activity of cytokines such as TNF-α, INF-γ, IL-12, and NO.
The presence of Lactobacillus in the gut has been shown to play a role in the regression of cancer as a result of its effects on immune regulation, which can be used as evidence of interactions between bacterial metabolites, immune cells, and epithelial cells (Huang R. et al., 2022). Lactobacillus can not only downregulate the Wnt/β-catenin pathway (Ghanavati et al., 2020), but also upregulate TNF-related apoptosis-inducing ligand, downregulate the transcription expression of cyclin D1 and BIRC5a, reduce the proliferation of CRC cells, and induce apoptosis of CRC cells (Tiptiri-Kourpeti et al., 2016). Shadnoush et al. (2013) demonstrated that the stability of the intestinal environment can be maintained by probiotics. Probiotics are involved in the prevention of CRC by inhibiting allergies, controlling serum cholesterol levels, modulating immune function, and inhibiting the growth of potentially harmful bacteria. In vitro studies (Zhu et al., 2011) have also found that probiotics can affect malignant biological activities of CRC cells such as proliferation, apoptosis, and adhesion. For example, Lactobacillus-derived polyphosphate induces apoptosis in CRC cells; Bacillus polyfermenticus exhibits adhesion to the surface of colon adenocarcinoma cells and suppresses the proliferation of CRC cells in a dose-dependent manner; and Bifidobacterium bifidum inhibits the proliferation of the CRC cell lines HT-29, SW480, and Caco-2 and thereby alters cell morphology and suppresses tumor growth. Lactobacillus acidophilus inhibits pathogenic bacteria and maintains the balance of the intestinal flora. Other probiotics such as Saccharomyces cerevisiae and Lactobacillus casei can induce apoptosis by blocking the cell cycle, and Bacillus polyfermenticus and Lactobacillus rhamnosus are associated with regulation of the cell cycle (Lin et al., 2009) and inhibition of the progression of CRC.
De Moreno De Leblanc and Perdigon (2010) demonstrated that fermented milk weakened intestinal inflammatory responses by increasing the number of IL-10-secreting cells, promoting apoptosis, and reducing the activity of procarcinogenic enzymes. These effects put gut microbiota into an ideal state and reduced the risk of cancer. Wang T. et al. (2022) confirmed that increasing the expression of the tight junction proteins claudin-1, zonula occludens-1, and occludin and increasing the loss of goblet cells can prevent damage to intestinal barrier function. In addition, these changes not only inhibit the expression of proinflammatory cytokines, such as IL-1β, IL-6, IL-17A, IFN-γ, and TNF-α, and chemokines, such as C-X-C motif chemokine ligand-2, C-X-C motif chemokine ligand-3, C-X-C motif chemokine ligand-5, and chemokine ligand-7, but also increase the abundance of probiotics and decrease the abundance of pathogenic bacteria and thus reduce the extent of intestinal inflammation. Colitis caused by CRC can be alleviated via the abovementioned three methods.
Xu et al. (2021) found in a study of Lactobacillus rhamnosus Probio-m9 that it can inhibit the expression of p-signal transducer and activator of transcription-3 and p-Akt, improve the balance of the intestinal flora, especially Akkermansia, Bifidobacterium, and Blautia, and suppress effects leading to CRC-related carcinogenesis. In particular, the study indicated that L. rhamnosus Probio-m9 can affect the metabolism of microbiota, especially glucose metabolism, and pointed out that glucose metabolism plays a far-reaching role as a driving force in the occurrence of tumors. Chung et al. (2021) developed a drug delivery system using the lactic acid bacterium Pediococcus pentosaceus, which carried a promoter that enabled the accurate expression of a fused protein containing P8, which is a small protein obtained from L. rhamnosus CBT LR5. This system significantly reduced the volume of tumors and inhibited the growth of CRC.
Bifidobacterium bifidum inhibits the development and progression of CRC in multiple ways, including antibacterial activity, improving vitamin metabolism, and enhancing immunity (Frick and Autenrieth, 2013), and also enhances the efficacy of antitumor immunotherapy by improving the local microenvironment (Sivan et al., 2015). Bifidobacterium can promote the expression of the proapoptotic gene BAX in CRC, reduce the expression of Bcl-2, and promote apoptosis of cancer cells. The phospholipid wall on the intestinal surface, as a ligand of toll-like receptor, can also induce apoptosis of cancer cells and participate in regulation of tumor growth (Yamamoto and Matsumoto, 2016; Ghanavati et al., 2020). Bifidobacterium spp. are among the most commonly used probiotics and have favorable effects on various diseases. However, Yoon and Michels (2021) showed in a study that only a specific Bifidobacterium strain improved the effect of cancer treatment. Bifidobacterium breve JCM92 enhanced antitumor immunity by increasing the number of CD8+ T cells and CD8+ effector T cells and increasing the ratios of CD8+ T cells to regulatory T cells and CD8+ effector T cells to regulatory T cells. B. breve JCM92 enhanced the efficacy of antitumor treatment, including oxaliplatin and programmed cell death protein-1 (PD-1) blockade, via lymphocyte-mediated immune responses.
In vitro research also discovered (Arimochi et al., 2009) that Salmonella typhimurium can suppress the proliferation of SW480 human CRC cells and induce cell cycle arrest and apoptosis in CRC cells. A growing number of studies have proved that microbe-based therapy is an available means of regulating gut microbiota and treating CRC. However, according to current relevant research, the safety of this therapy is still the greatest challenge. A recent study (Zheng et al., 2020) demonstrated that a modified form of the prebiotic dextran that encapsulated spores of Clostridium butyricum could be specifically enriched in tumor tissues and increase the populations of anticancer SCFA-producing bacteria, such as Eubacterium and Roseburia, which not only increased the overall abundance of intestinal microbiota but also transformed tumor-promoting intestinal microbiota into antitumor microbiota. Moreover, the easily modified prebiotic also represented a therapeutic target for the combination of capecitabine and diclofenac. Most importantly, this study indicated that C. butyricum spores encapsulated by the prebiotic dextran have no obvious side effects and provide a highly reliable and effective strategy for the treatment of CRC and other gastrointestinal diseases (Liu et al., 2020).
Chen D. et al. (2020) pointed out that C. butyricum downregulated the Wnt/β-catenin signaling pathway, reduced levels of pathogens, such as Clostridium XIVb and Clostridium XI, and increased the abundance of Lactobacillus. Importantly, this study found that treatment with C. butyricum can change the metabolites of microorganisms, such as secondary bile acids and SCFAs, and activate the G protein-coupled receptors GPR43 and GPR109A to inhibit the development and progression of CRC (Figure 3).
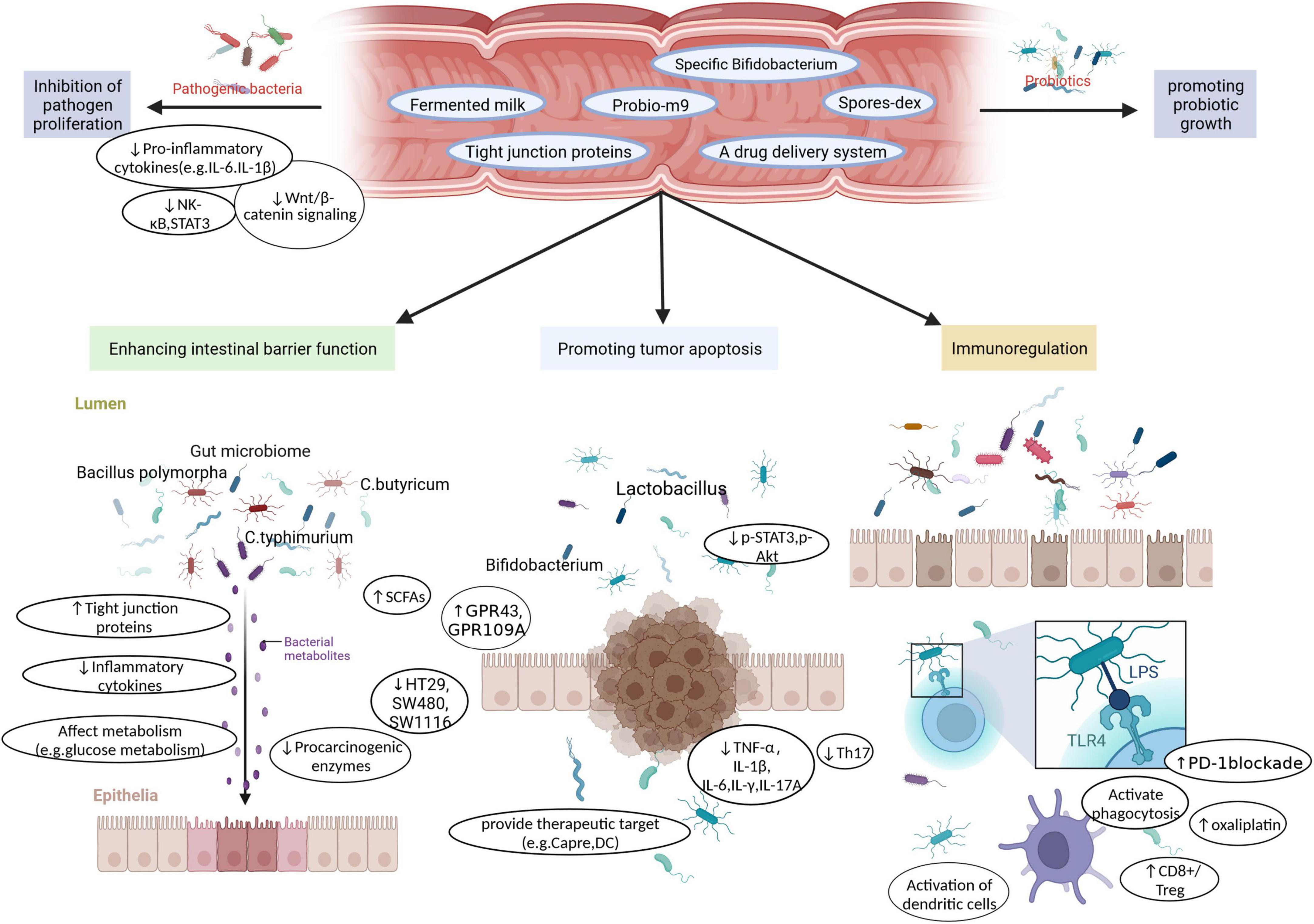
Figure 3. Probiotics as functional factors, such as Fermented milk, Probio-m9, specific Bifidobacterium, Spores-dex, Tight junction proteins, a drug delivery system, not only increase the proliferation of beneficial bacteria. It also inhibits the growth of pathogenic bacteria by inhibiting Pro-inflammatory cytokines, down-regulating Wnt/β-catenin signaling, inhibiting NK-κB and STAT3. Furthermore, various probiotic strains play a role in enhancing intestinal barrier function, promoting tumor cell apoptosis, and enhancing immune regulation through unique or common functions, to achieve the purpose of prevention or treatment of CRC.
Regulatory effect of prebiotics on intestinal flora in colorectal cancer
In recent years, the use of prebiotics as an adjuvant treatment strategy for CRC patients has been gradually promoted. In 1995, Gibson and Roberfroid defined a prebiotic as an undigested or indigestible food component that selectively stimulates the proliferation and metabolism of a range of bacteria and thereby benefits the health of the host. The activated bacteria should be naturally beneficial, such as bifidobacteria and lactobacilli. In 2008, the Food and Agriculture Organization of the United Nations added to the definition of prebiotics at a special conference on prebiotics: prebiotics are inanimate food components that promote the growth of beneficial intestinal bacteria while inhibiting harmful bacteria (Pineiro et al., 2008). In this section, we discuss the effects of prebiotics, including polysaccharides, pectin, phenolics, and food fiber, on CRC and the associated role of the intestinal flora (Table 1).
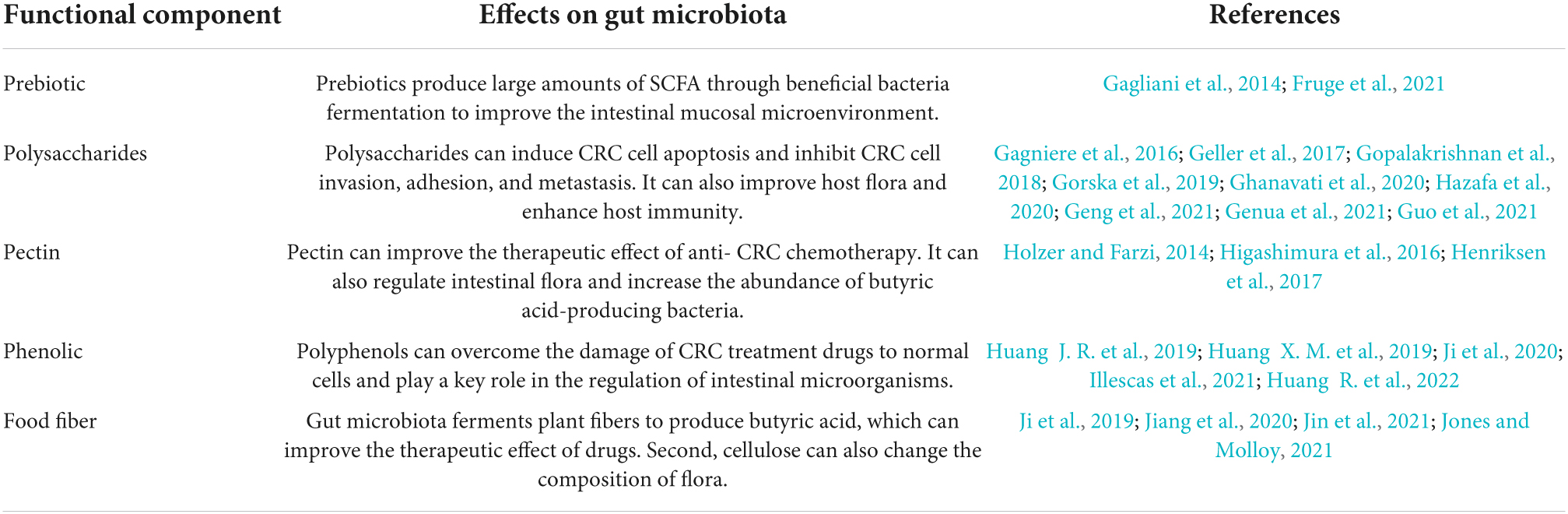
Table 1. This chart summarizes the effects of prebiotic on gut microbiota, and directly or indirectly plays a role in CRC.
Prebiotics can enable the production of a large amount of SCFAs via the fermentation of beneficial bacteria. SCFAs can not only provide 60–70% of the energy supply of mucosal cells and promote their growth but also acidify the gut environment, facilitate the production of mucin, improve the microenvironment of the intestinal mucosa, and reduce the migration of spoilage bacteria such as Bacillus spp., Clostridium spp., and Escherichia coli. (Eslami et al., 2019). SCFAs can also stimulate the expression of anti-inflammatory factors in dendritic cells and macrophages and induce the production of regulatory T cells and IL-10 (Wlodarczyk et al., 2021). In addition, prebiotics inhibit the release of TNF-α from inflammatory cells and exert anti-inflammatory effects (Genua et al., 2021). Moreover, SCFA analogs suppress the cycling of SW1116 cells in CRC, induce apoptosis, and reduce the expression of proto-oncogenes and cell proliferation, in which butyric acid is the main compound that plays a role (Rad et al., 2021).
Polysaccharides
Polysaccharides are natural polymers that are composed of more than 10 monosaccharide units linked by glycosidic bonds and are widely obtained from animals, plants, algae, and microorganisms (Song et al., 2021). Polysaccharides act against tumor cells directly or indirectly. Their direct antitumor effects are reflected in their ability to induce apoptosis in cancer cells, block the cell cycle, and inhibit tumor cell invasion, adhesion, metastasis, and growth (Shi et al., 2013; Shang et al., 2022). Secondly, the indirect antitumor effects of polysaccharides involve enhancing immune function (Feng et al., 2019).
Polysaccharides play an important role in human immune regulation. Firstly, polysaccharides can increase the number of macrophages and thus improve the body’s immunity (Li et al., 2020b). Secondly, polysaccharides affect the proliferation ability, subgroup structure, and cytokine secretion activity of lymphocytes (Ji et al., 2020). Thirdly, polysaccharides can activate natural killer cells and enhance their cytocidal activity (Ao and Kim, 2020). Fourthly, polysaccharides can activate the human complement system (Tao et al., 2017). Polysaccharides can also prevent CRC and reduce its recurrence rate. With regard to the regulation of the intestinal flora by polysaccharides, clinical experiments have shown that polysaccharides can stimulate the activity of intestinal probiotics, inhibit harmful intestinal bacteria, improve the intestinal inflammatory environment, reduce the release of carcinogens, and affect signaling pathways involved in tumor formation, which thereby inhibits the occurrence of intestinal tumors (Yang W. et al., 2022). In addition, SCFAs, which are metabolites of polysaccharides fermented by the intestinal flora, can inhibit the proliferation of tumor cells, induce the synthesis of mucin, and strengthen tight junctions between epithelial cells, which thereby prevents inflammation and ultimately inhibits the growth of CRC (Tao et al., 2017; Xue et al., 2020).
Owing to the lack of carbohydrate-activated enzymes in the human body, most polysaccharides cannot be directly digested and absorbed by the human body (Yu Y. et al., 2018). However, carbohydrate-activated enzymes encoded by human intestinal microflora can convert oligosaccharides and polysaccharides into monosaccharides to produce easily absorbed SCFAs and other metabolites (Ping et al., 2020; Li et al., 2022). Therefore, the intestinal flora serves as a bridge between the host and polysaccharides. For example, Yu Z. et al. (2018) confirmed that an extracellular polysaccharide from Rhizopus nigricans can resist hydrolysis in gastric conditions and successfully reach the intestine. Probiotics in the intestine can selectively use this polysaccharide, and its metabolites can increase both the villus length and villus length/crypt depth ratio in colon tissue and the number of goblet cells capable of secreting acidic mucus and thereby enhance the functioning of the immune system. Pool-Zobel and Sauer (2007) confirmed that inulin-type fructans produce SCFAs via microbial fermentation in the human intestine, of which butyric acid and propionic acid can inhibit the growth of CRC cells and histone deacetylase. Moreover, butyric acid can effectively suppress the apoptosis of tumor cells, reduce the metastasis of cancer cells, and inhibit carcinogens by increasing the expression of enzymes involved in detoxification. Guo et al. (2021) confirmed that polysaccharides from Ganoderma lucidum could inhibit toll-like receptor-4/MyD88/NF-κB signal transduction, increase the number of goblet cells, the secretion of mucin 2, and the expression of tight junction proteins, inhibit macrophage infiltration, downregulate the expression of IL-1β, inducible nitric oxide synthase, and cyclooxygenase-2, and suppress lipopolysaccharide (LPS)-induced inflammatory signatures and the activation of mitogen-activated protein kinase (MAPK) in RAW264.7 macrophages, HT-29 intestinal cells, and NCM460 cells. Ji et al. (2019) confirmed that polysaccharides from Ziziphus jujuba cv. Muzao can not only improve the intestinal flora of the host but also maintain metabolic function. Li et al. (2020b) confirmed that an apple polysaccharide inhibited the nuclear aggregation of β-catenin and thereby inhibited the activation of the Wnt pathway in colon tissue. The abovementioned studies have proved that the administration of polysaccharides is a valid way to treat CRC. Rivera-Huerta et al. (2017) discovered that fructans from chicory and Mexican agave can decrease the concentration of TNF-α and prevent the formation of intestinal polyps, villous atrophy, and lymphatic hyperplasia, which may help to reduce the risk of CRC. Wang et al. (2018) found that carboxymethylated pachyman has strong antitumor activity. A treatment regimen comprising carboxymethylated pachyman in combination with 5-FU markedly increased the levels of probiotics, such as Lactobacillus, Bacteroides, butyrate-producing bacteria, and acetic acid-producing bacteria. It played a crucial role in regulating the ecological balance of intestinal microbiota. This research also showed that carboxymethylated pachyman can alleviate 5-FU-induced colon injury, and the mechanism may be related to regulation of the NF-κB, Nrf2/antioxidant responsive element, and MAPK/p38 pathways. Yuan et al. (2021) found that polysaccharides from Albuca bracteata also have strong antitumor activity. Experimental data show that A. bracteata polysaccharides either alone or in combination with 5-FU can reduce the concentration of cancer cells and the expression of β-catenin and related proteins. Moreover, treatment with A. bracteata polysaccharides can specifically enrich Ruminococcus, Anaerostipes, and Oscillospira, effectively improve the composition of gut microbiota, and improve the levels of fecal SCFAs, such as propionic acid, acetic acid, and butyric acid, to improve the antitumor efficacy of 5-FU and reduce adverse reactions to 5-FU. LPS is a significant product of intestinal gram-negative flora. In blood and cancer tissues from patients with CRC, the level of LPS increased significantly. This suggests that LPS is an important target for the treatment of CRC. Song et al. (2018) are developing a nanoparticle-based LPS-trapping system for promoting PD-1-based immunity in CRC. The development of this LPS-trapping system offers new ideas for the future treatment of the intestinal microbiome in CRC. Scientists can cultivate genetically engineered bacteria, which can secrete LPS traps, and polymyxin B can even be produced directly by Bacillus polymyxa. Because these bacteria can reasonably enter the host gut and then remove LPS and regulate the CRC microenvironment, they may achieve therapeutic effects.
Pectin
Pectin (PC) is a kind of water-soluble food fiber that can protect the intestinal wall, activate useful bacteria in the intestine, adjust gastrointestinal function, remove intestinal waste, and prevent CRC. Palko-Labuz et al. (2021) found that novel enzymatically extracted PC from apples is an effective inhibitor of bacterial β-glucuronidase that can improve the cytotoxicity of irinotecan and promote the apoptosis of tumor cells. Their study suggested that PC can be used as an adjuvant for irinotecan treatment in patients with CRC and can reduce the side effects of chemotherapy and improve its therapeutic effect. Pi et al. (2020) confirmed that the PD-1/programmed death ligand-1 signaling pathway can modulate the metabolic activities of gut microbiota and thereby promote the immune monitoring function of the intestinal flora with regard to tumors. Another study (Zhang et al., 2021) showed that PC in combination with an anti-PD-1 monoclonal antibody can safely improve the efficacy of the anti-PD-1 antibody. Moreover, the use of PC can beneficially regulate the intestinal flora. PC increases the abundance of butyric acid and promotes the infiltration of T lymphocytes and thus enhances the efficacy of anti-PD-1 monoclonal antibodies.
Phenolics
Polyphenol (Zhao and Jiang, 2021) is the general name for a group of chemical substances in plants, which are so named because they contain multiple phenolic groups. In the plant world, there are more than 6500 polyphenols or phenolic compounds and their derivatives, which generally exist in grains, vegetables, fruits, and dual-purpose plants used for both medicine and food (Huang X. M. et al., 2019). Polyphenols are the antioxidant substances that people ingest in the largest amounts from food every day. In humans and other animals, phenolic acids formed by the metabolism of polyphenols by the intestinal flora have biological activities such as scavenging free radicals, antioxidant and antitumor activities, bacteriostasis, and improving immunity (Yang Q. et al., 2022). Polyphenols can avoid damage caused by CRC drugs to normal cells by only acting on tumor cells, leaving normal cells unaffected. Therefore, anti-CRC immunotherapy based on polyphenols and intestinal microbiota (Zhao and Jiang, 2021) is worth investigating.
Luo et al. (2021) confirmed that ferulic acid was transformed into 4-vinylguaiacol in the intestine by gut microbiota. The activity of the latter was mediated by cell cycle arrest at the G1 phase and induction of apoptosis. Dobani et al. (2021) have shown that raspberry polyphenols can reduce DNA damage and protect the colonic epithelium by upregulation of the cytoprotective Nrf2/antioxidant responsive element pathway. Wang et al. (2020) pointed out that tea polyphenols (TPs) can exert chemopreventive and therapeutic effects on CRC via multiple pathways mediated by gut microbiota. For example, TPs can inhibit the proliferation of tumor cells via molecular pathways such as the phosphoinositide 3-kinase/Akt, MAPK, Wnt/β-catenin, and Janus kinase/signal transducer and activator of transcription pathways. TP is the general term for a class of polyhydroxyphenol compounds present in tea, of which the main members are catechin compounds (Wang X. et al., 2022). TPs may inhibit CRC metastasis by inhibiting matrix metalloproteinase-9, which is closely associated with tumor metastasis and plays an important role in the infiltration of tumor cells (Mileo et al., 2019). Augusti et al. (2021) presented the chemopreventive effect of jaboticaba peel powder in terms of reducing inflammation and CRC cell proliferation in a complex 3D model of CRC for the first time. Jaboticaba peel powder contains phytochemicals extracted from jaboticaba fruit peel, which are fermented by flora in the intestine to form phenolic compounds.
Food fiber
Short-chain fatty acids, such as butyric acid and propionic acid, are produced via the fermentation of dietary fiber by the intestinal flora. A high concentration of butyric acid can inhibit the proliferation of CRC cells independently of the Warburg effect and inactivate a large number of carcinogenic signals by inhibiting histone deacetylases. In addition, butyric acid can promote the proliferation of regulatory T cells and strengthen intestinal immunity (Tuncil et al., 2020; Liu et al., 2022). It has been pointed out that fermentation of dietary fiber by the intestinal flora can produce butyric acid, which can improve the anti-tumor cell proliferation effect of drugs such as irinotecan (Encarnacao et al., 2018). Windey et al. (2015) confirmed that dietary addition of wheat bran extract, which is a prebiotic supplement containing arabinoxylan oligosaccharides, decreased colonic protein fermentation, changed the metabolic mode in the colon, and selectively promoted the growth of bifidobacteria. Moreover, it was found that cellulose changed the composition of the gut flora, increased the richness of probiotics, decreased the abundance of inflammatory intestinal bacteria, and inhibited colonic inflammation and tumor formation induced by treatment with azoxymethane/dextran sulfate sodium (Li Q. et al., 2021). Yoon and Michels (2021) found by a feasibility study that combined supplementation with fiber and calcium can prevent CRC via changes in the composition of intestinal microbiota. This is mainly because the consumption of inulin can increase the absorption of calcium.
Regulatory effect of metabolites of intestinal microorganisms in colorectal cancer
As we have already mentioned with regard to the relationship between probiotics and CRC, Clostridium butyricum is a probiotic that produces butyric acid, which is a classical SCFA with strong anticancer activity. So how does butyric acid work? Data from Li et al. (2017) demonstrated that histone deacetylase relied upon the activity of butyric acid in the inhibition of the motility of CRC cells via deactivation of Akt/extracellular-regulated kinase signaling. Besides, Geng et al. (2021) discovered that butyric acid, in addition to acting as a histone deacetylase inhibitor and activating the G protein-coupled receptor GPR109a, not only significantly inhibited glucose transport and glycolysis in CRC cells by reducing the contents of glucose transporter 1 in the cell membrane and glucose-6-phosphate dehydrogenase in the cytoplasm but also dramatically improved the chemotherapeutic effect of 5-FU on tumor cells. It has been shown that blueberry and coffee bean extracts rich in hydroxycinnamic acids (HCAs) inhibit the growth of CRC-associated pathogenic bacteria, such as Fusobacterium nucleatum, Bacteroides fragilis, and Prevotella intermedia (Budryn et al., 2015; Zheng et al., 2019). In addition, HCAs can promote potential anti-oncogenes in Bifidobacterium, Lactobacillus, and Roseburia and reduce the occurrence of CRC (Leonard et al., 2021). Experiments by Li Z. et al. (2021) demonstrated that hydroxycarboxylic acid receptor-2 mediated tumor development and progression by promoting gut mucosal immunity and reducing the expression of oncogenes. However, HCAs are a double-edged sword because they not only promote the growth of Bacteroides spp. but also lead to organism-wide inflammatory reactions and metabolic disturbances, which induce the occurrence of CRC (Coman and Vodnar, 2020). Therefore, we need more research to confirm the relationship between HCAs and CRC.
Furthermore, it has been pointed out that trimethylamine N-oxide is a metabolite produced from choline and carnitine in food by intestinal microorganisms and may be an independent risk factor. Consequently, suppression of the formation of trimethylamine N-oxide is a potential drug target for reducing the risk of development of CRC (Oellgaard et al., 2017). Tryptophan (Ala, 2021) metabolites affect CRC via the indole pathway in intestinal microbiota. The same study also found that tryptophan metabolites can play a role in suppressing inflammatory enteritis and CRC via the 5-hydroxytryptamine system in intestinal pigment cells and the kynurenine pathway in immune cells and the intestinal lining (Table 2).
Regulatory effect of traditional Chinese medicine on intestinal flora in colorectal cancer
Chinese medicine regulates the structure of the intestinal microflora and modulates the alteration of intestinal flora
Increasing the level of probiotics in the gut
After gavage with curcumin in rats, Weng and Goel (2020) discovered a significant improvement in the balance of the intestinal flora by quantifying fecal bacteria, with a decrease in levels of Escherichia coli and an increase in those of probiotics, such as Bifidobacterium and Lactobacillus. Besides, curcumin can upregulate the expression of peroxisome proliferator-activated receptor-γ and inhibit cancer cell proliferation (Zhang et al., 2016). Li et al. (2018) isolated three inulin-type fructans with high polymerization degrees from the roots of Codonopsis pilosula and found that they could stimulate an increase in bifidobacteria levels in the gut. Jin et al. (2021) demonstrated that p-cymene could stimulate the growth of probiotic bacteria such as isobacteria, bifidobacteria, and Clostridium IV in the intestinal tract.
Inhibiting the growth of pathogenic bacteria
Sui et al. (2020) found that YYFZBJS inhibited the production of regulatory T cells by altering intestinal bacterial populations, including those of Bacteroides fragilis and Lachnospiraceae spp. Although YYFZBJS itself had no suppressive effect on tumor cell proliferation, YYFZBJS-mediated alterations in regulatory T cells limited their growth, while β-catenin phosphorylation levels were reduced. Moreover, the researchers suggested YYFZBJS as a new potential drug option for the treatment of CRC. Shao et al. (2021) discovered that Xiao-Chai-Hu-Tang reversed dysregulation of gut microbiota by downregulating toll-like receptor-4/MyD88/NF-κB signaling and, in particular, by reducing the levels of Parabacteroides, Blautia, and Ruminococcaceae bacteria. The essential role of the intestinal flora in the treatment of CRC with depressive symptoms was elucidated.
Research has proved that berberine can reduce the population of Clostridium nucleatum, as well as inhibiting the overgrowth of Pseudomonas aeruginosa and Staphylococcus spp. caused by C. nucleatum colonization, which leads to a decrease in the secretion of immune factors and thus inhibits the occurrence of CRC (Yu et al., 2015; Chen H. et al., 2020). Furthermore, berberine can downregulate the expression of cyclooxygenase-2 and prostaglandin E2 and inhibit the invasion and metastasis of tumor cells (Liu et al., 2015). Ji et al. (2019) showed that polysaccharides from Ziziphus jujuba cv. Muzao reduced the growth of pathogenic bacteria such as Sclerotinia sclerotiorum, alleviated dysbiosis of the intestinal flora caused by colitis, and thus prevented the onset of tumors. Paris polyphylla and its active components polyphyllin VI and pennogenin 3-O-β-chacotrioside can inhibit the growth of Fusobacterium and thus inhibit the migration and invasion of CRC cells. Moreover, polyphyllin VI and pennogenin 3-O-β-chacotrioside can have a significant inhibitory effect on Bifidobacterium. For this reason, when they are used in the treatment of CRC, supplementation with bifidobacteria is required to overcome this inhibitory effect (Lin et al., 2021). Triterpenoid phytosaponins derived from gibberellins can reduce the amount of sulfate-reducing bacteria in the intestine of APCMin/ + model mice, strengthen the intestinal epithelial mucosal barrier, and simultaneously downregulate the expression of Src in the intestinal mucosa and other proteins and inhibit tumor growth (Chen L. et al., 2016).
Chinese medicine increases the abundance of gut microflora and improves the intestinal microecological balance
Chen et al. (2018) researched the effect of Panax notoginseng saponins on the intestinal microflora in mice. The results indicated that these saponins markedly increased the abundance and diversity of the intestinal flora, especially Akkermansia spp., and that ginsenoside compound K, which is a metabolite of P. notoginseng saponins produced by gut microflora, had anti-CRC activity, suppressed the proliferation of tumor cells, and regulated the homeostasis of the intestinal flora. Terasaki et al. (2021) found that fucoxanthin remarkably reduced levels of Bacteroidales, Rikenellaceae, Lachnospiraceae, and Erysipelotrichaceae spp. in the intestine of mice and that the abundances of Lactobacillus, Lactococcus, Bifidobacterium, and several butyrate-producing bacteria increased. This was accompanied by a change in the ratio of Firmicutes to Bacteroidetes. These findings indicated that fucoxanthin induced changes in the bowel microbiota and the tumor microenvironment, which may be an important mechanism responsible for its potential anticancer effect. Zhu et al. (2021) found that alisol B 23-acetate decreased the levels of pathogenic bacteria, such as Klebsiella, Citrobacter, and Akkermansia, and increased the populations of beneficial bacteria, such as Bacteroides, Lactobacillus, and Alloprevotella. Wang et al. (2017) identified that the regulation of the microbial balance by American ginseng contributed to the maintenance of intestinal homeostasis, as evidenced by restoration in the form of population shifts and recovery from dysbiosis among gut microbial populations, with significant changes in abundance in the families Bacteroidaceae and Porphyromonadaceae.
Another study revealed that Sini Decoction (Wang et al., 2021) effectively increased the diversity of the intestinal flora, markedly increased the number of dominant intestinal bacterial groups, including Bacillus coagulans, Lactobacillus, Xylophilus, and Bifidobacterium, and reduced levels of pathogenic bacteria, including sulfate-reducing bacteria and Bacteroides fragilis, and thereby protected the colonic mucosal barrier and regulated the intestinal microecological balance. Quxie Capsule (Sun et al., 2020) notably reduced the proportion of harmful intestinal bacteria, such as Shigella and Cyanobacteria, and increased the abundance of beneficial bacteria, such as Actinobacteria and Lachnospiraceae. Wu Mei Wan (Jiang et al., 2020) dramatically increased the content of Firmicutes, decreased the content of Bacteroidetes, and downregulated the expression of p65, IL-6, and p-signal transducer and activator of transcription-3 in cancer cells in CAC mice.
Chinese medicine participates in immune function by regulating intestinal microflora
In vitro experiments demonstrated that Dendrobium Sonia polysaccharide could activate the immune system by increasing the viability and phagocytic capacity of macrophages. These experiments indicated that it may be an effective macrophage activator that can promote immune function in mice with cyclophosphamide-induced immunosuppression by promoting the release of the cytokines IL-6, TNF-α, and interferon-γ. In addition, Dendrobium Sonia polysaccharide significantly alleviated dysregulation of the intestinal microbiota in mice with cyclophosphamide-induced immunosuppression and increased the proportion of probiotic bacteria (Liu et al., 2019; Xie et al., 2019). Bifidobacterium can play a protective role in TNF-α-induced inflammatory responses in Caco-2 cells via the NF-κB and p38/MAPK pathways (Nie et al., 2020). Astragalus can modulate the structure of the gut flora and regulate immunity by inhibiting activation of NF-κB, which in turn decreases high expression levels of proinflammatory genes and weakens intestinal inflammatory responses mediated by proinflammatory factors (Zhang et al., 2019). Baicalin is converted into baicalein by human intestinal microorganisms. On comparing the antiproliferative effects of baicalein and baicalin, experiments have confirmed that the latter has a more potent effect. Moreover, baicalin was able to reduce damage to the organism by activating the Wnt/β-catenin signaling pathway and promoting the expression of Bax protein, which leads to apoptosis of cancer cells (Wang et al., 2015, 2019).
Sankaranarayanan et al. (2021) identified five bacterial species, namely, Flavonifractor plautii, Bacillus glycinifermentans, Bacteroides eggerthii, Eubacterium eligens, and Olsenella scatoligenes, which can degrade quercetin and produce different bioactive metabolites. Some of these species have been proved to have anti-CRC cell antiproliferative effects. In particular, B. glycinifermentans and F. plautii were prominent in terms of their ability to degrade quercetin, and the authors considered them to have excellent potential for the development of anti-CRC probiotics for the human gut. The combination of microencapsulated Bifidobacterium bifidum and Lactobacillus gasseri with quercetin, which was invented by Benito et al. (2021), suppressed the Wnt/β-catenin signaling pathway in the mouse colon and thereby inhibited the progression of CRC. Another study revealed that Gegen Qinlian decoction not only regulated components of the intestinal microbiota and promoted the antitumor activity of anti-PD-1 antibodies (Li et al., 2020a) but also enhanced the immunity of patients and protected the functioning of the intestinal barrier (Lv et al., 2019; Figure 4).
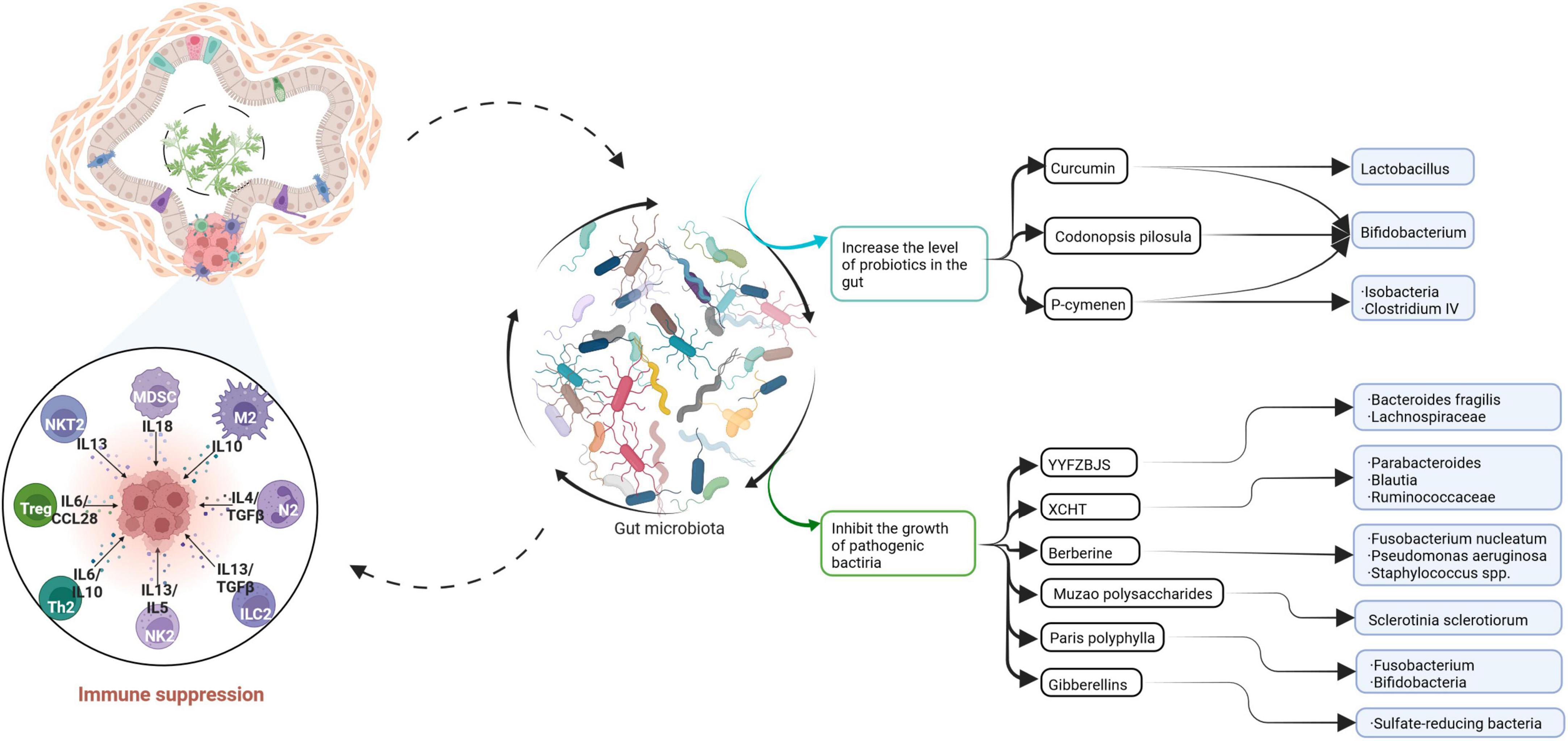
Figure 4. This paper summarizes the relationship between traditional Chinese medicine and gut microbiota and CRC. Curcumin, Codonopsis pilosula, and P-cymenen increased the abundance of intestinal probiotics. Some Chinese herbal extracts or prescriptions can inhibit the growth of pathogenic bacteria. Traditional Chinese medicine increases the richness of gut microbiota, thereby enhancing the body’s immunity and playing an anti-CRC role.
Regulatory effect of chemical treatments on intestinal flora in colorectal cancer
Chemotherapeutic approaches for treating CRC have received increased attention in recent years. Many cytotoxic agents are regularly used in combination, for example, 5-FU in combination with L-oxaliplatin and capecitabine in combination with either oxaliplatin (XELOX regimen), irinotecan (XELIR regimen), cyclooxygenase-2 inhibitors, cetuximab, or bevacizumab. According to investigation data, however, the therapeutic efficacy of chemotherapy frequently leads to failure of CRC treatment as a result of drug resistance.
There is a growing amount of empirical data suggesting that the use of metformin increases the level of SCFA-producing intestinal microorganisms. Metformin (Jones and Molloy, 2021) indirectly affects SCFAs by altering the composition of the gut flora and has a role in the treatment of CRC. In their experimental study, (Huang J. R. et al., 2019) produced liposomal irinotecan, which remarkably reduced the level of prostaglandin E2 in colon tissue and significantly reduced the rate of bifidobacterial infection in comparison with irinotecan.
Aspirin-based gut microbe therapy has been investigated. In a randomized clinical study, aspirin was proved to increase the abundance of Prevotella, Akkermansia, and Ruminococcaceae spp. while reducing the abundance of Bacteroides, Parabacteroides, and Dorea spp., which is closely associated with the reduction of the risk of CRC achieved with aspirin (Prizment et al., 2020). A study by Diao and Cai (2020) indicated that intestinal microorganisms interacted with aspirin. On the one hand, gut microbes converted aspirin into inactive metabolites and enhanced its preventive effect against CRC. On the other hand, aspirin increased the abundance of some probiotic bacteria in the gut, such as bifidobacteria and lactobacilli. Another group of studies pointed out that biotransformation of aspirin by intestinal flora may be a source of 2,3-dihydroxybenzoic acid and 2,5-dihydroxybenzoic acid, which have an inhibitory effect on the growth of CRC cells and play critical roles in the anticancer effect of aspirin (Sankaranarayanan et al., 2020).
Other studies have shown that fecal microbiota transplantation can safely alleviate intestinal inflammation, maintain the integrity of the colonic epithelium, and reduce intestinal damage caused by FOLFOX therapy, which is a 5-FU-based chemotherapeutic regimen (Chang et al., 2020).
Regulatory effect of diet on intestinal flora in colorectal cancer
Different dietary components, microbiota, and colorectal cancer
Fat
Wan et al. (2019) organized low-fat, medium-fat, and high-fat diet intervention experiments on 217 healthy young adults and found that the diversity of intestinal microbiota in young adults on a high-fat diet was significantly reduced. The abundance of Alipipes and Bacteroides increased significantly, while that of Faecalibacterium was significantly reduced. Furthermore, it was found in related animal experiments that a high-fat diet promoted the secretion of bile acids in mice. An imbalance in the intestinal microenvironment and an increase in levels of secondary bile acids will lead to deterioration of the functioning of large intestinal epithelial cells and damage to the mucosal layer and thereby increase the risk of CRC in mice (Ridlon et al., 2016). Moreover, when mice were given a high-fat diet, the abundance of Lactobacillales decreased and that of the Clostridium subcluster XIVa increased (Higashimura et al., 2016). In addition, after rats were switched from a low-fat diet to a high-fat diet, the diversity of intestinal microbiota decreased markedly, and the relative abundance of Firmicutes increased greatly (Taira et al., 2015).
Protein
The influences of different sources of protein on the composition of intestinal microbiota and their roles in the occurrence of CRC are significantly different. Studies have indicated that the intake of chicken protein can increase the abundance of Lactobacillus in rat intestines, whereas soy protein can increase the abundance of Ruminococcus and Bacteroides and reduce the relative abundance of Lactobacillus (Zhu et al., 2017). The intake of fish meat can more effectively prevent the occurrence of CRC, whereas the intake of red meat will increase the risk of CRC (Farvid et al., 2021).
Carbohydrates
Fermentation of complex carbohydrates (e.g., dietary fiber) by intestinal microflora produces high levels of SCFAs, such as butyric acid. High levels of SCFAs may reduce the risk of the development of CRC. Butyric acid induces apoptosis in colon cells, inhibits the activity of CRC cells by blocking the expression of genes regulating histone deacetylase (Li et al., 2017), and also activates glycoisomerization via a cAMP-dependent mechanism.
Micronutrients
Recent work has demonstrated that micronutrients can influence the development and progression of CRC by modulating the structure of the intestinal microbial community.
Vitamin D (Tabatabaeizadeh et al., 2018) plays a significant role in maintaining the stability of gut microbiota and regulating intestinal probiotics and the intestinal barrier regulated by Akkermansia muciniphila. Vitamin D (Tabatabaeizadeh et al., 2018), as a cell differentiation inducer, affects telomerase activity and exerts an antitumor effect via its receptors on cells. An experimental study by Zhou et al. (2020) discovered that a sufficient amount of vitamin D can effectively reverse CRC. This is because vitamin D has a strong regulatory effect on the integrity of the intestinal barrier mediated by A. muciniphila. This finding may suggest a new target for the therapy of CRC via intestinal microbiota. Vitamin E (Yang et al., 2021) is a highly potent fat-soluble intracellular antioxidant that can inhibit the formation of free radicals and the activity of protein kinase, which is closely associated with the growth and differentiation of tumor cells. Vitamin E in the form of δ-tocotrienol and its metabolite 13’-carboxychromanol increased levels of potentially beneficial Lactococcus and Bacteroides. Yang et al. (2021) supported the hypothesis that this metabolite may play a role in δ-tocotrienol’s anticancer activity and modulation of gut microbes. A sufficient amount of vitamin A can increase the diversity of intestinal microbiota, and vitamin A plays an indirect role in regulating the intestinal barrier and immune responses. All-trans-retinoic acid (Cantorna et al., 2019) is the most active form of vitamin A and induces apoptosis of tumor cells and has a therapeutic effect in CRC. The intestinal flora acts as a mediating effector. Vitamin B12 (Lurz et al., 2020) causes changes in the abundance of gut microbiota, such as a decrease in Lactobacillus and an increase in Bacteroides.
In contrast, Phipps et al. (2020) discovered from the effect of iron on the gut flora in CRC that supplementation with oral iron preparations in iron-deficient CRC patients may lead to noteworthy changes in gut microbiota, the growth of harmful microorganisms, and exacerbation of the disease process. Hence, micronutrients themselves and their effects on the intestinal microecological balance may contribute to the development of CRC, and their route of intake and disruptive effect on the intestinal flora need to be taken into account when supplementing with micronutrient preparations, especially iron preparations.
Different dietary patterns, gut microbiota, and colorectal cancer
Mediterranean diet
Studies (Illescas et al., 2021) have shown that people in southern European countries with the Mediterranean diet are much less likely to suffer from CRC than those in other European and American countries. The Mediterranean diet is a representative model of a healthy diet that has been gradually promoted in recent years. Its characteristic feature is to encourage people to consume more nutritious fresh fruits and vegetables, beans, nuts, and whole grains, with fish and olive oil as the main sources of fat, supplemented by small amounts of dairy products, red meat, and wine. The Mediterranean diet is enriched with omega-3 polyunsaturated fatty acids (e.g., docosahexaenoic acid and eicosapentaenoic acid) and phytochemicals (e.g., polyphenols and flavonoids) that inhibit the expression of colonic proinflammatory factors and improve and regulate the intestinal microenvironment and thus reduce the risk of CRC (Illescas et al., 2021; Yammine et al., 2021). Yammine et al. (2021) found that dietary plant polyphenols in the Mediterranean diet have a significant effect on the prevention of CRC. These dietary plant polyphenols include apigenin, curcumin, epigallocatechin gallate, quercetin, rutin, and resveratrol. Further understanding of the mechanisms of polyphenol–microbiota interactions may provide further insights into strategies for the prevention or treatment of CRC. Metabolites produced by gut microbiota are more pharmacologically active than these natural molecules.
Vegetarian diet
The strictest vegetarian diet is defined as a diet that completely excludes meat and foods of animal origin (Sakkas et al., 2020). Currently, a limited number of prospective studies have demonstrated the positive effect of a vegetarian diet on the prevention of CRC (Orlich et al., 2015; Henriksen et al., 2017). At present, there is no definite conclusion on the specific mechanism by which a vegetarian diet can reduce the risk of CRC. Nevertheless, it is certain that reducing the intake of red meat and animal protein, together with the high contents of dietary fiber, monounsaturated and polyunsaturated fats, antioxidant vitamins, and phytochemicals such as polyphenols and carotenoids in a vegetarian diet, can increase the microbial diversity in the intestine, maintain intestinal homeostasis, and alleviate inflammation and oxidative stress in the intestine and thus reduce the risk of CRC (Hazafa et al., 2020; Sakkas et al., 2020).
Reducing intake of inflammatory foods
The term “inflammatory foods” refers to processed meat, red meat, viscera, refined flour, and sugary drinks, which are the foods most closely associated with CRC-related inflammation. Therefore, reducing the intake of inflammatory foods plays a role in the prevention and treatment of CRC. These foods have the opposite effects to “anti-inflammatory foods,” such as green leafy vegetables, dark yellow vegetables, whole grains, coffee, and fruit juice. Research has shown that dark green leafy vegetables (Fruge et al., 2021), rice bran (So et al., 2021), an increased intake of dietary indoles (Wyatt and Greathouse, 2021), vitamin D, calcium, folic acid, fruits, and other vegetables (Song et al., 2015) play substantial roles in the prevention of CRC. How does the composition of the diet prevent CRC and reduce the risk of CRC? After researching and analyzing the relationships between dietary interventions and CRC in recent years, the authors found that the mediating effect of gut microbiota is an important factor in dietary interventions and the occurrence of CRC. However, more work is needed to determine the effects of diet on gut microbiota. In the future, research on the individual differences and diversity of gut microbiota may contribute to a deeper comprehension of the treatment of CRC and may lead to deeper insights into the field of medicine.
Processed products, gut microbiota, and colorectal cancer
Fernandez et al. (2018) developed an anthocyanin-rich sausage that prevented CRC after processing. Anthocyanins were the main components responsible for its anticancer effect and have been described as essential modulators of intestinal microbiota. For example, supplementation with anthocyanins in animal models of CRC was able to reduce the abundance of pathogenic bacteria (e.g., Enterococcus spp. and Desulfovibrio spp.) and increase the abundance of probiotics (e.g., Eubacterium rectale and Faecalibacterium prausnitzii) (Chen et al., 2018). They can therefore be used as an alternative to dietary interventions in CRC, especially in people who consume excessive amounts of meat products. Besides, Zhuang et al. (2021) observed in their experiments that CD8+ T cells were enriched in the intestinal microenvironment of mice with CRC treated orally with jujube powder, which increased the diversity of the intestinal microflora and the abundance of bifidobacteria and thus contributed to the production of butyric acid, which could inhibit tumor growth, in cecal contents. Moreover, the use of jujube powder to enrich the intestinal flora can improve the efficacy of chemotherapy for CRC, such as cyclophosphamide. Furthermore, the consumption of First Leaf, which consists of blackcurrant extract powder, lactoferrin, and lutein, and Cassis Anthomix 30, which comprises blackcurrant extract powder, as a novel prebiotic preparation had an anticancer effect in humans by increasing the abundance of intestinal probiotics, namely, Lactobacillus and Bifidobacterium, which may have been achieved by markedly decreasing the fecal pH and the activity of bacterial β-glucuronidase (Molan et al., 2014). In addition, the beneficial effect of Boswellia serrata resin extract in alleviating microbial dysbiosis in azoxymethane/dextran sulfate sodium-induced CRC was demonstrated by alterations in the composition of gut microbiota in the form of an increase in the amount of Clostridium perfringens and a decrease in the abundance of Bacteroidetes (Chou et al., 2017; Table 3).
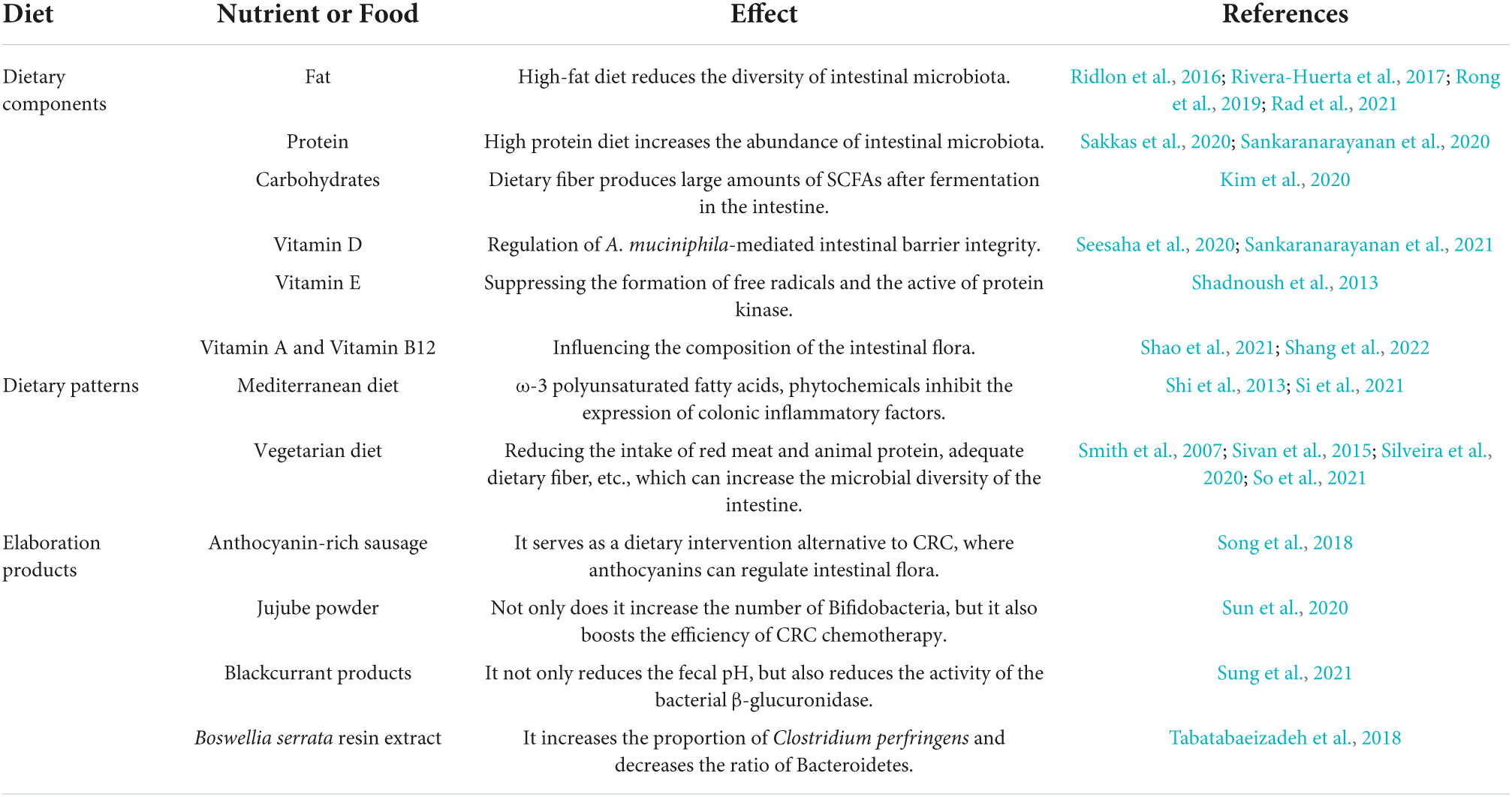
Table 3. The chart summarizes how different dietary components, dietary patterns, and some elaboration products affect gut microbiota and thus play a role in preventing CRC.
Conclusion
At present, the study of microbial communities has been applied in related fields of human disease and has achieved success. However, the role and significance of microbial communities in treatment have not been fully studied, and we still face many unresolved problems. Therefore, it is necessary to conduct further research on current topics to verify existing findings and develop treatment strategies. It is worth noting that current research on microbial regulation of anti-CRC therapy mostly focuses on mice, and how to apply the resulting academic findings in clinical practice remains a challenge. Although the pathological and immune responses of mice implanted with human microbiota are similar to those of humans, there are still differences (Smith et al., 2007).
In the future, if the most beneficial microbial compositions under various clinical conditions can be identified, it will be possible to use microbial compositions as biomarkers, diagnostic tools, or therapeutic targets and develop microbial therapies that can not only enhance the effects of anticancer therapies but also reduce their systemic toxicity. Therefore, treatment interventions targeting microbial groups will become one of the next areas of focus for precise and personalized treatment of cancer. This may lead to breakthroughs in various areas of medicine and not only support the development and production of immunotherapies or innovative vaccines for cancer treatment but also improve drug delivery in other intestinal diseases and prevent and reduce inflammation. A clinical trial of this strategy has the potential to improve the feasibility of using probiotics as comprehensive drug carriers in non-invasive cancer treatment in humans and as suitable adjuvants in traditional anticancer therapy.
Author contributions
MC, LS, and SZ: conceptualization. MC, WL, and NL: writing—original draft preparation. MC, AZ, QW, SZ, and LS: writing—review and editing. AZ and LS: visualization. All authors have read and agreed to the published version of the manuscript.
Funding
This work was supported by Foundation of National Natural Science Foundation of China (82004176) and Foundation of Science and Technology Department of Sichuan Province (2020YJ0147).
Conflict of interest
The authors declare that the research was conducted in the absence of any commercial or financial relationships that could be construed as a potential conflict of interest.
Publisher’s note
All claims expressed in this article are solely those of the authors and do not necessarily represent those of their affiliated organizations, or those of the publisher, the editors and the reviewers. Any product that may be evaluated in this article, or claim that may be made by its manufacturer, is not guaranteed or endorsed by the publisher.
References
Ala, M. (2021). Tryptophan metabolites modulate inflammatory bowel disease and colorectal cancer by affecting immune system. Int. Rev. Immunol. 41, 326–345. doi: 10.1080/08830185.2021.1954638
Alpert, O., Begun, L., Issac, T., and Solhkhah, R. (2021). The brain-gut axis in gastrointestinal cancers. J. Gastrointest. Oncol. 12, S301–S310.
Amitay, E. L., Carr, P. R., Gies, A., Laetsch, D. C., and Brenner, H. (2020). Probiotic/synbiotic treatment and postoperative complications in colorectal cancer patients: systematic review and meta-analysis of randomized controlled trials. Clin. Transl. Gastroenterol. 11:e00268.
Ao, X., and Kim, I. H. (2020). Effects of Achyranthes bidentata polysaccharides on performance, immunity, antioxidant capacity, and meat quality in Pekin ducks. Poult. Sci. 99, 4884–4891. doi: 10.1016/j.psj.2020.06.026
Arimochi, H., Morita, K., Nakanishi, S., Kataoka, K., and Kuwahara, T. (2009). Production of apoptosis-inducing substances from soybean protein by Clostridium butyricum: characterization of their toxic effects on human colon carcinoma cells. Cancer Lett. 277, 190–198. doi: 10.1016/j.canlet.2008.12.006
Augusti, P. R., Quatrin, A., Mello, R., Bochi, V. C., Rodrigues, E., Prazeres, I. D., et al. (2021). Antiproliferative effect of colonic fermented phenolic compounds from jaboticaba (Myrciaria trunciflora) fruit peel in a 3D cell model of colorectal cancer. Molecules 26:4469. doi: 10.3390/molecules26154469
Avuthu, N., and Guda, C. (2022). Meta-analysis of altered gut microbiota reveals microbial and metabolic biomarkers for colorectal cancer. Microbiol. Spectr. e0001322. Online ahead of print,
Bajramagic, S., Hodzic, E., Mulabdic, A., Holjan, S., Smajlovic, S. V., and Rovcanin, A. (2019). Usage of probiotics and its clinical significance at surgically treated patients sufferig from colorectal carcinoma. Med. Arch. 73, 316–320. doi: 10.5455/medarh.2019.73.316-320
Benito, I., Encio, I. J., Milagro, F. I., Alfaro, M., Martinez-Penuela, A., Barajas, M., et al. (2021). Microencapsulated Bifidobacterium bifidum and Lactobacillus gasseri in combination with quercetin inhibit colorectal cancer development in Apc(Min/+) mice. Int. J. Mol. Sci. 22:4906. doi: 10.3390/ijms22094906
Brennan, C. A., and Garrett, W. S. (2016). Gut microbiota, inflammation, and colorectal cancer. Annu. Rev. Microbiol. 70, 395–411.
Budryn, G., Palecz, B., Rachwal-Rosiak, D., Oracz, J., Zaczynska, D., Belica, S., et al. (2015). Effect of inclusion of hydroxycinnamic and chlorogenic acids from green coffee bean in beta-cyclodextrin on their interactions with whey, egg white and soy protein isolates. Food Chem. 168, 276–287. doi: 10.1016/j.foodchem.2014.07.056
Bultman, S. J. (2016). The microbiome and its potential as a cancer preventive intervention. Semin. Oncol. 43, 97–106.
Cantorna, M. T., Snyder, L., and Arora, J. (2019). Vitamin A and vitamin D regulate the microbial complexity, barrier function, and the mucosal immune responses to ensure intestinal homeostasis. Crit. Rev. Biochem. Mol. Biol. 54, 184–192. doi: 10.1080/10409238.2019.1611734
Chang, C. W., Lee, H. C., Li, L. H., Chiang Chiau, J. S., Wang, T. E., Chuang, W. H., et al. (2020). Fecal microbiota transplantation prevents intestinal injury, Upregulation of toll-like receptors, and 5-Fluorouracil/Oxaliplatin-induced toxicity in colorectal cancer. Int. J. Mol. Sci. 21:386.
Chen, D., Jin, D., Huang, S., Wu, J., Xu, M., Liu, T., et al. (2020). Clostridium butyricum, a butyrate-producing probiotic, inhibits intestinal tumor development through modulating Wnt signaling and gut microbiota. Cancer Lett. 469, 456–467. doi: 10.1016/j.canlet.2019.11.019
Chen, H., Zhang, F., Zhang, J., Zhang, X., and Yao, Q. (2020). A holistic view of berberine inhibiting intestinal carcinogenesis in conventional mice based on microbiome-metabolomics analysis. Front. Immunol. 11:588079. doi: 10.3389/fimmu.2020.588079
Chen, J., Liang, H., Lu, J., He, Y., and Lai, R. (2022). Probiotics improve postoperative adaptive immunity in colorectal cancer patients: a systematic review and meta-analysis. Nutr. Cancer 1–8. Online ahead of print, doi: 10.1080/01635581.2022.2056619
Chen, L., Brar, M. S., Leung, F. C., and Hsiao, W. L. (2016). Triterpenoid herbal saponins enhance beneficial bacteria, decrease sulfate-reducing bacteria, modulate inflammatory intestinal microenvironment and exert cancer preventive effects in ApcMin/+ mice. Oncotarget 7, 31226–31242. doi: 10.18632/oncotarget.8886
Chen, L., Jiang, B., Zhong, C., Guo, J., Zhang, L., Mu, T., et al. (2018). Chemoprevention of colorectal cancer by black raspberry anthocyanins involved the modulation of gut microbiota and SFRP2 demethylation. Carcinogenesis 39, 471–481. doi: 10.1093/carcin/bgy009
Chen, W., Zheng, R., Baade, P. D., Zhang, S., Zeng, H., Bray, F., et al. (2016). Cancer statistics in China, 2015. CA Cancer J. Clin. 66, 115–132.
Chou, Y. C., Suh, J. H., Wang, Y., Pahwa, M., Badmaev, V., Ho, C. T., et al. (2017). Boswellia serrata resin extract alleviates azoxymethane (AOM)/dextran sodium sulfate (DSS)-induced colon tumorigenesis. Mol. Nutr. Food Res. 61:1600984. doi: 10.1002/mnfr.201600984
Chung, Y., Ryu, Y., An, B. C., Yoon, Y. S., Choi, O., Kim, T. Y., et al. (2021). A synthetic probiotic engineered for colorectal cancer therapy modulates gut microbiota. Microbiome 9:122. doi: 10.1186/s40168-021-01071-4
Coman, V., and Vodnar, D. C. (2020). Hydroxycinnamic acids and human health: recent advances. J. Sci. Food Agric. 100, 483–499.
Dahmus, J. D., Kotler, D. L., Kastenberg, D. M., and Kistler, C. A. (2018). The gut microbiome and colorectal cancer: a review of bacterial pathogenesis. J. Gastrointest. Oncol. 9, 769–777.
Di, Y. Z., Han, B. S., Di, J. M., Liu, W. Y., and Tang, Q. (2019). Role of the brain-gut axis in gastrointestinal cancer. World J. Clin. Cases 7, 1554–1570.
Diao, F., and Cai, S. (2020). Aspirin-based chemoprevention of colorectal cancer: the role for gut microbiota. Cancer Commun. 40, 633–635.
Dinan, T. G., and Cryan, J. F. (2017). The microbiome-gut-brain axis in health and disease. Gastroenterol. Clin. North Am. 46, 77–89.
Dobani, S., Latimer, C., Mcdougall, G. J., Allwood, J. W., Pereira-Caro, G., Moreno-Rojas, J. M., et al. (2021). Ex vivo fecal fermentation of human ileal fluid collected after raspberry consumption modifies (poly)phenolics and modulates genoprotective effects in colonic epithelial cells. Redox Biol. 40:101862. doi: 10.1016/j.redox.2021.101862
Encarnacao, J. C., Pires, A. S., Amaral, R. A., Goncalves, T. J., Laranjo, M., Casalta-Lopes, J. E., et al. (2018). Butyrate, a dietary fiber derivative that improves irinotecan effect in colon cancer cells. J. Nutr. Biochem. 56, 183–192. doi: 10.1016/j.jnutbio.2018.02.018
Eslami, M., Yousefi, B., Kokhaei, P., Hemati, M., Nejad, Z. R., Arabkari, V., et al. (2019). Importance of probiotics in the prevention and treatment of colorectal cancer. J. Cell Physiol. 234, 17127–17143.
Evans, J. M., Morris, L. S., and Marchesi, J. R. (2013). The gut microbiome: the role of a virtual organ in the endocrinology of the host. J. Endocrinol. 218, R37–R47.
Farvid, M. S., Sidahmed, E., Spence, N. D., Mante Angua, K., Rosner, B. A., and Barnett, J. B. (2021). Consumption of red meat and processed meat and cancer incidence: a systematic review and meta-analysis of prospective studies. Eur. J. Epidemiol. 36, 937–951.
Feng, Z., Yang, R., Wu, L., Tang, S., Wei, B., Guo, L., et al. (2019). Atractylodes macrocephala polysaccharides regulate the innate immunity of colorectal cancer cells by modulating the TLR4 signaling pathway. Oncol. Targets Ther. 12, 7111–7121. doi: 10.2147/OTT.S219623
Fernandez, J., Garcia, L., Monte, J., Villar, C. J., and Lombo, F. (2018). Functional anthocyanin-rich sausages diminish colorectal cancer in an animal model and reduce pro-inflammatory bacteria in the intestinal microbiota. Genes 9:133. doi: 10.3390/genes9030133
Frick, J. S., and Autenrieth, I. B. (2013). The gut microflora and its variety of roles in health and disease. Curr. Top. Microbiol. Immunol. 358, 273–289.
Fruge, A. D., Smith, K. S., Riviere, A. J., Tenpenny-Chigas, R., Demark-Wahnefried, W., Arthur, A. E., et al. (2021). A dietary intervention high in green leafy vegetables reduces oxidative DNA damage in adults at increased risk of colorectal cancer: biological outcomes of the randomized controlled meat and three greens (M3G) feasibility trial. Nutrients 13:1220.
Gagliani, N., Hu, B., Huber, S., Elinav, E., and Flavell, R. A. (2014). The fire within: microbes inflame tumors. Cell 157, 776–783. doi: 10.1016/j.cell.2014.03.006
Gagniere, J., Raisch, J., Veziant, J., Barnich, N., Bonnet, R., Buc, E., et al. (2016). Gut microbiota imbalance and colorectal cancer. World J. Gastroenterol. 22, 501–518.
Geller, L. T., Barzily-Rokni, M., Danino, T., Jonas, O. H., Shental, N., Nejman, D., et al. (2017). Potential role of intratumor bacteria in mediating tumor resistance to the chemotherapeutic drug gemcitabine. Science 357, 1156–1160. doi: 10.1126/science.aah5043
Geng, H. W., Yin, F. Y., Zhang, Z. F., Gong, X., and Yang, Y. (2021). Butyrate suppresses glucose metabolism of colorectal cancer cells via GPR109a-AKT signaling pathway and enhances chemotherapy. Front. Mol. Biosci. 8:634874. doi: 10.3389/fmolb.2021.634874
Genua, F., Mirkovic, B., Mullee, A., Levy, M., Gallagher, W. M., Vodicka, P., et al. (2021). Association of circulating short chain fatty acid levels with colorectal adenomas and colorectal cancer. Clin. Nutr. ESPEN 46, 297–304.
Ghanavati, R., Akbari, A., Mohammadi, F., Asadollahi, P., Javadi, A., Talebi, M., et al. (2020). Lactobacillus species inhibitory effect on colorectal cancer progression through modulating the Wnt/beta-catenin signaling pathway. Mol. Cell Biochem. 470, 1–13. doi: 10.1007/s11010-020-03740-8
Gopalakrishnan, V., Helmink, B. A., Spencer, C. N., Reuben, A., and Wargo, J. A. (2018). The influence of the gut microbiome on cancer, immunity, and cancer immunotherapy. Cancer Cell 33, 570–580.
Gorska, A., Przystupski, D., Niemczura, M. J., and Kulbacka, J. (2019). Probiotic bacteria: a promising tool in cancer prevention and therapy. Curr. Microbiol. 76, 939–949.
Guo, C., Guo, D., Fang, L., Sang, T., Wu, J., Guo, C., et al. (2021). Ganoderma lucidum polysaccharide modulates gut microbiota and immune cell function to inhibit inflammation and tumorigenesis in colon. Carbohydr. Polym. 267:118231. doi: 10.1016/j.carbpol.2021.118231
Hazafa, A., Rehman, K. U., Jahan, N., and Jabeen, Z. (2020). The role of polyphenol (Flavonoids) compounds in the treatment of cancer cells. Nutr. Cancer 72, 386–397.
Henriksen, H. B., Raeder, H., Bohn, S. K., Paur, I., Kvaerner, A. S., Billington, S. A., et al. (2017). The Norwegian dietary guidelines and colorectal cancer survival (CRC-NORDIET) study: a food-based multicentre randomized controlled trial. BMC Cancer 17:83. doi: 10.1186/s12885-017-3072-4
Higashimura, Y., Naito, Y., Takagi, T., Uchiyama, K., Mizushima, K., Ushiroda, C., et al. (2016). Protective effect of agaro-oligosaccharides on gut dysbiosis and colon tumorigenesis in high-fat diet-fed mice. Am. J. Physiol. Gastrointest. Liver Physiol. 310, G367–G375. doi: 10.1152/ajpgi.00324.2015
Holzer, P., and Farzi, A. (2014). Neuropeptides and the microbiota-gut-brain axis. Adv. Exp. Med. Biol. 817, 195–219.
Huang, J. R., Lee, M. H., Li, W. S., and Wu, H. C. (2019). Liposomal irinotecan for treatment of colorectal cancer in a preclinical model. Cancers 11:281. doi: 10.3390/cancers11030281
Huang, R., Wu, F., Zhou, Q., Wei, W., Yue, J., Xiao, B., et al. (2022). Lactobacillus and intestinal diseases: mechanisms of action and clinical applications. Microbiol. Res. 260:127019.
Huang, X. M., Yang, Z. J., Xie, Q., Zhang, Z. K., Zhang, H., and Ma, J. Y. (2019). Natural products for treating colorectal cancer: a mechanistic review. Biomed. Pharmacother. 117:109142.
Illescas, O., Rodriguez-Sosa, M., and Gariboldi, M. (2021). Mediterranean diet to prevent the development of colon diseases: a meta-analysis of gut microbiota studies. Nutrients 13:2234. doi: 10.3390/nu13072234
Ji, X., Hou, C., Gao, Y., Xue, Y., Yan, Y., and Guo, X. (2020). Metagenomic analysis of gut microbiota modulatory effects of jujube (Ziziphus jujuba Mill.) polysaccharides in a colorectal cancer mouse model. Food Funct. 11, 163–173. doi: 10.1039/c9fo02171j
Ji, X., Hou, C., Zhang, X., Han, L., Yin, S., Peng, Q., et al. (2019). Microbiome-metabolomic analysis of the impact of Zizyphus jujuba cv. Muzao polysaccharides consumption on colorectal cancer mice fecal microbiota and metabolites. Int. J. Biol. Macromol. 131, 1067–1076. doi: 10.1016/j.ijbiomac.2019.03.175
Jiang, F., Liu, M., Wang, H., Shi, G., Chen, B., Chen, T., et al. (2020). Wu Mei Wan attenuates CAC by regulating gut microbiota and the NF-kB/IL6-STAT3 signaling pathway. Biomed. Pharmacother. 125:109982. doi: 10.1016/j.biopha.2020.109982
Jin, H., Leng, Q., Zhang, C., Zhu, Y., and Wang, J. (2021). P-cymene prevent high-fat diet-associated colorectal cancer by improving the structure of intestinal flora. J. Cancer 12, 4355–4361. doi: 10.7150/jca.57049
Jones, G. R., and Molloy, M. P. (2021). Metformin, microbiome and protection against colorectal cancer. Dig. Dis. Sci. 66, 1409–1414. doi: 10.1007/s10620-020-06390-4
Kim, M., Vogtmann, E., Ahlquist, D. A., Devens, M. E., Kisiel, J. B., Taylor, W. R., et al. (2020). Fecal metabolomic signatures in colorectal adenoma patients are associated with gut microbiota and early events of colorectal cancer pathogenesis. mBio 11, e03186–19.
Krishnan, S., Alden, N., and Lee, K. (2015). Pathways and functions of gut microbiota metabolism impacting host physiology. Curr. Opin. Biotechnol. 36, 137–145.
Leonard, W., Zhang, P., Ying, D., and Fang, Z. (2021). Hydroxycinnamic acids on gut microbiota and health. Compr. Rev. Food Sci. Food Saf. 20, 710–737.
Li, J., Zhang, X., Cao, L., Ji, J., and Gao, J. (2018). Three inulin-type fructans from Codonopsis pilosula (Franch.) Nannf. roots and their prebiotic activity on Bifidobacterium longum. Molecules 23:3123. doi: 10.3390/molecules23123123
Li, Q., Chen, C., Liu, C., Sun, W., Liu, X., Ci, Y., et al. (2021). The effects of cellulose on AOM/DSS-treated C57BL/6 colorectal cancer mice by changing intestinal flora composition and inflammatory factors. Nutr. Cancer 73, 502–513. doi: 10.1080/01635581.2020.1756355
Li, Q., Ding, C., Meng, T., Lu, W., Liu, W., Hao, H., et al. (2017). Butyrate suppresses motility of colorectal cancer cells via deactivating Akt/ERK signaling in histone deacetylase dependent manner. J. Pharmacol. Sci. 135, 148–155. doi: 10.1016/j.jphs.2017.11.004
Li, Y., Li, Z. X., Xie, C. Y., Fan, J., Lv, J., Xu, X. J., et al. (2020a). Gegen Qinlian decoction enhances immunity and protects intestinal barrier function in colorectal cancer patients via gut microbiota. World J. Gastroenterol. 26, 7633–7651. doi: 10.3748/wjg.v26.i48.7633
Li, Y., Wang, S., Sun, Y., Xu, W., Zheng, H., Wang, Y., et al. (2020b). Apple polysaccharide protects ICR mice against colitis associated colorectal cancer through the regulation of microbial dysbiosis. Carbohydr. Polym. 230:115726. doi: 10.1016/j.carbpol.2019.115726
Li, Z., Mccafferty, K. J., and Judd, R. L. (2021). Role of HCA2 in regulating intestinal homeostasis and suppressing colon carcinogenesis. Front. Immunol. 12:606384. doi: 10.3389/fimmu.2021.606384
Lin, L. T., Shi, Y. C., Choong, C. Y., and Tai, C. J. (2021). The fruits of paris polyphylla inhibit colorectal cancer cell migration induced by Fusobacterium nucleatum-derived extracellular vesicles. Molecules 26:4081. doi: 10.3390/molecules26134081
Lin, P. W., Myers, L. E., Ray, L., Song, S. C., Nasr, T. R., Berardinelli, A. J., et al. (2009). Lactobacillus rhamnosus blocks inflammatory signaling in vivo via reactive oxygen species generation. Free Radic. Biol. Med. 47, 1205–1211. doi: 10.1016/j.freeradbiomed.2009.07.033
Liu, L., Tabung, F. K., Zhang, X., Nowak, J. A., Qian, Z. R., Hamada, T., et al. (2018). Diets that promote colon inflammation associate with risk of colorectal carcinomas that contain Fusobacterium nucleatum. Clin. Gastroenterol. Hepatol. 16, 1622–1631.e3. doi: 10.1016/j.cgh.2018.04.030
Liu, M., Xie, W., Wan, X., and Deng, T. (2020). Clostridium butyricum modulates gut microbiota and reduces colitis associated colon cancer in mice. Int. Immunopharmacol. 88:106862. doi: 10.1016/j.intimp.2020.106862
Liu, S., Fang, Z., and Ng, K. (2022). Recent development in fabrication and evaluation of phenolic-dietary fiber composites for potential treatment of colonic diseases. Crit. Rev. Food Sci. Nutr. 1–25. Online ahead of print, doi: 10.1080/10408398.2022.2043236
Liu, W., Yan, R., and Zhang, L. (2019). Dendrobium sonia polysaccharide regulates immunity and restores the dysbiosis of the gut microbiota of the cyclophosphamide-induced immunosuppressed mice. Chin. J. Nat. Med. 17, 600–607. doi: 10.1016/S1875-5364(19)30062-7
Liu, X., Ji, Q., Ye, N., Sui, H., Zhou, L., Zhu, H., et al. (2015). Berberine inhibits invasion and metastasis of colorectal cancer cells via COX-2/PGE2 mediated JAK2/STAT3 signaling pathway. PLoS One 10:e0123478. doi: 10.1371/journal.pone.0123478
Lu, Y., Wang, L., Zhang, J., Li, J., and Wan, G. (2019). Induction of CD8 T cell cytotoxicity by fecal bacteria from healthy individuals and colorectal cancer patients. Biochem. Biophys. Res. Commun. 516, 1007–1012. doi: 10.1016/j.bbrc.2019.06.078
Luo, Y., Wang, C. Z., Sawadogo, R., Yuan, J., Zeng, J., Xu, M., et al. (2021). 4-Vinylguaiacol, an active metabolite of ferulic acid by enteric microbiota and probiotics, possesses significant activities against drug-resistant human colorectal cancer cells. ACS Omega 6, 4551–4561. doi: 10.1021/acsomega.0c04394
Lurz, E., Horne, R. G., Maattanen, P., Wu, R. Y., Botts, S. R., Li, B., et al. (2020). Vitamin B12 deficiency alters the gut microbiota in a murine model of colitis. Front. Nutr. 7:83. doi: 10.3389/fnut.2020.00083
Lv, J., Jia, Y., Li, J., Kuai, W., Li, Y., Guo, F., et al. (2019). Gegen Qinlian decoction enhances the effect of PD-1 blockade in colorectal cancer with microsatellite stability by remodelling the gut microbiota and the tumour microenvironment. Cell Death Dis. 10:415. doi: 10.1038/s41419-019-1638-6
Mego, M., Holec, V., Drgona, L., Hainova, K., Ciernikova, S., and Zajac, V. (2013). Probiotic bacteria in cancer patients undergoing chemotherapy and radiation therapy. Complement. Ther. Med. 21, 712–723.
Mileo, A. M., Nistico, P., and Miccadei, S. (2019). Polyphenols: immunomodulatory and therapeutic implication in colorectal cancer. Front. Immunol. 10:729. doi: 10.3389/fimmu.2019.00729
Miller, A. H., Ancoli-Israel, S., Bower, J. E., Capuron, L., and Irwin, M. R. (2008). Neuroendocrine-immune mechanisms of behavioral comorbidities in patients with cancer. J. Clin. Oncol. 26, 971–982. doi: 10.1200/JCO.2007.10.7805
Mohammadi, M., Mirzaei, H., and Motallebi, M. (2022). The role of anaerobic bacteria in the development and prevention of colorectal cancer: a review study. Anaerobe 73:102501. doi: 10.1016/j.anaerobe.2021.102501
Molan, A. L., Liu, Z., and Plimmer, G. (2014). Evaluation of the effect of blackcurrant products on gut microbiota and on markers of risk for colon cancer in humans. Phytother. Res. 28, 416–422. doi: 10.1002/ptr.5009
Nie, N., Bai, C., Song, S., Zhang, Y., Wang, B., and Li, Z. (2020). Bifidobacterium plays a protective role in TNF-alpha-induced inflammatory response in Caco-2 cell through NF-kappaB and p38MAPK pathways. Mol. Cell Biochem. 464, 83–91. doi: 10.1007/s11010-019-03651-3
Oellgaard, J., Winther, S. A., Hansen, T. S., Rossing, P., and Von Scholten, B. J. (2017). Trimethylamine N-oxide (TMAO) as a new potential therapeutic target for insulin resistance and cancer. Curr. Pharm. Des. 23, 3699–3712. doi: 10.2174/1381612823666170622095324
Orlich, M. J., Singh, P. N., Sabate, J., Fan, J., Sveen, L., Bennett, H., et al. (2015). Vegetarian dietary patterns and the risk of colorectal cancers. JAMA Intern. Med. 175, 767–776.
Osterlund, P., Ruotsalainen, T., Korpela, R., Saxelin, M., Ollus, A., Valta, P., et al. (2007). Lactobacillus supplementation for diarrhoea related to chemotherapy of colorectal cancer: a randomised study. Br. J. Cancer 97, 1028–1034. doi: 10.1038/sj.bjc.6603990
Palko-Labuz, A., Maksymowicz, J., Sobieszczanska, B., Wikiera, A., Skonieczna, M., Wesolowska, O., et al. (2021). Newly obtained apple pectin as an adjunct to irinotecan therapy of colorectal cancer reducing E. coli adherence and beta-glucuronidase activity. Cancers 13:2952. doi: 10.3390/cancers13122952
Panebianco, C., Andriulli, A., and Pazienza, V. (2018). Pharmacomicrobiomics: exploiting the drug-microbiota interactions in anticancer therapies. Microbiome 6:92. doi: 10.1186/s40168-018-0483-7
Pennisi, E. (2013). Biomedicine. Cancer therapies use a little help from microbial friends. Science 342:921. doi: 10.1126/science.342.6161.921
Petra, A. I., Panagiotidou, S., Hatziagelaki, E., Stewart, J. M., Conti, P., and Theoharides, T. C. (2015). Gut-microbiota-brain axis and its effect on neuropsychiatric disorders with suspected immune dysregulation. Clin. Ther. 37, 984–995.
Phipps, O., Al-Hassi, H. O., Quraishi, M. N., Kumar, A., and Brookes, M. J. (2020). Influence of iron on the gut microbiota in colorectal cancer. Nutrients 12:2512.
Pi, H., Huang, L., Liu, H., Liang, S., and Mei, J. (2020). Effects of PD-1/PD-L1 signaling pathway on intestinal flora in patients with colorectal cancer. Cancer Biomark. 28, 529–535. doi: 10.3233/CBM-201606
Pineiro, M., Asp, N. G., Reid, G., Macfarlane, S., Morelli, L., Brunser, O., et al. (2008). FAO technical meeting on prebiotics. J. Clin. Gastroenterol. 42 Suppl 3(Pt. 2), S156–S159. doi: 10.1097/MCG.0b013e31817f184e
Ping, Q., Zheng, M., Dai, X., and Li, Y. (2020). Metagenomic characterization of the enhanced performance of anaerobic fermentation of waste activated sludge with CaO2 addition at ambient temperature: fatty acid biosynthesis metabolic pathway and CAZymes. Water Res. 170:115309. doi: 10.1016/j.watres.2019.115309
Pool-Zobel, B. L., and Sauer, J. (2007). Overview of experimental data on reduction of colorectal cancer risk by inulin-type fructans. J. Nutr. 137, 2580S–2584S. doi: 10.1093/jn/137.11.2580S
Popa, C. M., Ianosi, S. L., Dorobantu, S. C., and Saftoiu, A. (2022). Gut microbiota imbalance in metastatic colorectal patients treated with EGFRI and long-term antibiotic therapy for cutaneous toxicity: a pilot study. Cureus 14:e25007. doi: 10.7759/cureus.25007
Prizment, A. E., Staley, C., Onyeaghala, G. C., Vivek, S., Thyagarajan, B., Straka, R. J., et al. (2020). Randomised clinical study: oral aspirin 325 mg daily vs placebo alters gut microbial composition and bacterial taxa associated with colorectal cancer risk. Aliment. Pharmacol. Ther. 52, 976–987. doi: 10.1111/apt.16013
Rad, A. H., Aghebati-Maleki, L., Kafil, H. S., and Abbasi, A. (2021). Molecular mechanisms of postbiotics in colorectal cancer prevention and treatment. Crit. Rev. Food Sci. Nutr. 61, 1787–1803. doi: 10.1080/10408398.2020.1765310
Ridlon, J. M., Wolf, P. G., and Gaskins, H. R. (2016). Taurocholic acid metabolism by gut microbes and colon cancer. Gut Microbes 7, 201–215.
Rivera-Huerta, M., Lizarraga-Grimes, V. L., Castro-Torres, I. G., Tinoco-Mendez, M., Macias-Rosales, L., Sanchez-Bartez, F., et al. (2017). Functional effects of prebiotic fructans in colon cancer and calcium metabolism in animal models. Biomed. Res. Int. 2017:9758982. doi: 10.1155/2017/9758982
Rong, J., Liu, S., Hu, C., and Liu, C. (2019). Single probiotic supplement suppresses colitis-associated colorectal tumorigenesis by modulating inflammatory development and microbial homeostasis. J. Gastroenterol. Hepatol. 34, 1182–1192. doi: 10.1111/jgh.14516
Sakkas, H., Bozidis, P., Touzios, C., Kolios, D., Athanasiou, G., Athanasopoulou, E., et al. (2020). Nutritional status and the influence of the vegan diet on the gut microbiota and human health. Medicina 56:88.
Sankaranarayanan, R., Kumar, D. R., Altinoz, M. A., and Bhat, G. J. (2020). Mechanisms of colorectal cancer prevention by Aspirin-A literature review and perspective on the role of COX-dependent and -independent pathways. Int. J. Mol. Sci. 21, 9018. doi: 10.3390/ijms21239018
Sankaranarayanan, R., Sekhon, P. K., Ambat, A., Nelson, J., Jose, D., Bhat, G. J., et al. (2021). Screening of human gut bacterial culture collection identifies species that biotransform quercetin into metabolites with anticancer properties. Int. J. Mol. Sci. 22:7045. doi: 10.3390/ijms22137045
Seesaha, P. K., Chen, X., Wu, X., Xu, H., Li, C., Jheengut, Y., et al. (2020). The interplay between dietary factors, gut microbiome and colorectal cancer: a new era of colorectal cancer prevention. Future Oncol. 16, 293–306. doi: 10.2217/fon-2019-0552
Shadnoush, M., Shaker Hosseini, R., Mehrabi, Y., Delpisheh, A., Alipoor, E., Faghfoori, Z., et al. (2013). Probiotic yogurt Affects Pro- and anti-inflammatory factors in patients with inflammatory bowel disease. Iran J. Pharm. Res. 12, 929–936.
Shang, H., Niu, X., Cui, W., Sha, Z., Wang, C., Huang, T., et al. (2022). Anti-tumor activity of polysaccharides extracted from Pinus massoniana pollen in colorectal cancer- in vitro and in vivo studies. Food Funct. 13, 6350–6361. doi: 10.1039/d1fo03908c
Shao, S., Jia, R., Zhao, L., Zhang, Y., Guan, Y., Wen, H., et al. (2021). Xiao-Chai-Hu-Tang ameliorates tumor growth in cancer comorbid depressive symptoms via modulating gut microbiota-mediated TLR4/MyD88/NF-kappaB signaling pathway. Phytomedicine 88:153606. doi: 10.1016/j.phymed.2021.153606
Shi, X., Zhao, Y., Jiao, Y., Shi, T., and Yang, X. (2013). ROS-dependent mitochondria molecular mechanisms underlying antitumor activity of Pleurotus abalonus acidic polysaccharides in human breast cancer MCF-7 cells. PLoS One 8:e64266. doi: 10.1371/journal.pone.0064266
Si, H., Yang, Q., Hu, H., Ding, C., Wang, H., and Lin, X. (2021). Colorectal cancer occurrence and treatment based on changes in intestinal flora. Semin. Cancer Biol. 70, 3–10.
Silveira, D. S. C., Veronez, L. C., Lopes-Junior, L. C., Anatriello, E., Brunaldi, M. O., and Pereira-Da-Silva, G. (2020). Lactobacillus bulgaricus inhibits colitis-associated cancer via a negative regulation of intestinal inflammation in azoxymethane/dextran sodium sulfate model. World J. Gastroenterol. 26, 6782–6794. doi: 10.3748/wjg.v26.i43.6782
Sivan, A., Corrales, L., Hubert, N., Williams, J. B., Aquino-Michaels, K., Earley, Z. M., et al. (2015). Commensal Bifidobacterium promotes antitumor immunity and facilitates anti-PD-L1 efficacy. Science 350, 1084–1089. doi: 10.1126/science.aac4255
Smith, K., Mccoy, K. D., and Macpherson, A. J. (2007). Use of axenic animals in studying the adaptation of mammals to their commensal intestinal microbiota. Semin. Immunol. 19, 59–69.
So, W. K. W., Chan, J. Y. W., Law, B. M. H., Choi, K. C., Ching, J. Y. L., Chan, K. L., et al. (2021). Effects of a rice bran dietary intervention on the composition of the intestinal microbiota of adults with a high risk of colorectal cancer: a pilot randomised-controlled trial. Nutrients 13:526. doi: 10.3390/nu13020526
Sobhani, I., Tap, J., Roudot-Thoraval, F., Roperch, J. P., Letulle, S., Langella, P., et al. (2011). Microbial dysbiosis in colorectal cancer (CRC) patients. PLoS One 6:e16393. doi: 10.1371/journal.pone.0016393
Sokol, H., Landman, C., Seksik, P., Berard, L., Montil, M., Nion-Larmurier, I., et al. (2020). Fecal microbiota transplantation to maintain remission in Crohn’s disease: a pilot randomized controlled study. Microbiome 8:12. doi: 10.1186/s40168-020-0792-5
Song, M., Garrett, W. S., and Chan, A. T. (2015). Nutrients, foods, and colorectal cancer prevention. Gastroenterology 148, 1244–1260.e16.
Song, Q., Wang, Y., Huang, L., Shen, M., Yu, Y., Yu, Q., et al. (2021). Review of the relationships among polysaccharides, gut microbiota, and human health. Food Res. Int. 140:109858.
Song, W., Tiruthani, K., Wang, Y., Shen, L., Hu, M., Dorosheva, O., et al. (2018). Trapping of lipopolysaccharide to promote immunotherapy against colorectal cancer and attenuate liver metastasis. Adv. Mater. 30:e1805007.
Sui, H., Zhang, L., Gu, K., Chai, N., Ji, Q., Zhou, L., et al. (2020). YYFZBJS ameliorates colorectal cancer progression in Apc(Min/+) mice by remodeling gut microbiota and inhibiting regulatory T-cell generation. Cell Commun. Signal. 18:113. doi: 10.1186/s12964-020-00596-9
Sun, L., Yan, Y., Chen, D., and Yang, Y. (2020). Quxie capsule modulating gut microbiome and its association with T cell regulation in patients with metastatic colorectal cancer: result from a randomized controlled clinical trial. Integr. Cancer Ther. 19:1534735420969820. doi: 10.1177/1534735420969820
Sung, H., Ferlay, J., Siegel, R. L., Laversanne, M., Soerjomataram, I., Jemal, A., et al. (2021). Global cancer statistics 2020: GLOBOCAN estimates of incidence and mortality worldwide for 36 cancers in 185 countries. CA Cancer J. Clin. 71, 209–249.
Tabatabaeizadeh, S. A., Tafazoli, N., Ferns, G. A., Avan, A., and Ghayour-Mobarhan, M. (2018). Vitamin D, the gut microbiome and inflammatory bowel disease. J. Res. Med. Sci. 23:75.
Taira, T., Yamaguchi, S., Takahashi, A., Okazaki, Y., Yamaguchi, A., Sakaguchi, H., et al. (2015). Dietary polyphenols increase fecal mucin and immunoglobulin A and ameliorate the disturbance in gut microbiota caused by a high fat diet. J. Clin. Biochem. Nutr. 57, 212–216. doi: 10.3164/jcbn.15-15
Tao, J. H., Duan, J. A., Jiang, S., Feng, N. N., Qiu, W. Q., and Ling, Y. (2017). Polysaccharides from Chrysanthemum morifolium Ramat ameliorate colitis rats by modulating the intestinal microbiota community. Oncotarget 8, 80790–80803. doi: 10.18632/oncotarget.20477
Terasaki, M., Kubota, A., Kojima, H., Maeda, H., Miyashita, K., Kawagoe, C., et al. (2021). Fucoxanthin and colorectal cancer prevention. Cancers 13:2379.
Thaiss, C. A., Zmora, N., Levy, M., and Elinav, E. (2016). The microbiome and innate immunity. Nature 535, 65–74.
Tiptiri-Kourpeti, A., Spyridopoulou, K., Santarmaki, V., Aindelis, G., Tompoulidou, E., Lamprianidou, E. E., et al. (2016). Lactobacillus casei exerts anti-proliferative effects accompanied by apoptotic cell death and up-regulation of TRAIL in colon carcinoma cells. PLoS One 11:e0147960. doi: 10.1371/journal.pone.0147960
Tuncil, Y. E., Thakkar, R. D., Arioglu-Tuncil, S., Hamaker, B. R., and Lindemann, S. R. (2020). Subtle variations in dietary-fiber fine structure differentially influence the composition and metabolic function of gut microbiota. mSphere 5, e00180–20. doi: 10.1128/mSphere.00180-20
Valdes, A. M., Walter, J., Segal, E., and Spector, T. D. (2018). Role of the gut microbiota in nutrition and health. BMJ 361:k2179.
Vieira, A. T., Teixeira, M. M., and Martins, F. S. (2013). The role of probiotics and prebiotics in inducing gut immunity. Front. Immunol. 4:445. doi: 10.3389/fimmu.2013.00445
Wan, Y., Wang, F., Yuan, J., Li, J., Jiang, D., Zhang, J., et al. (2019). Effects of dietary fat on gut microbiota and faecal metabolites, and their relationship with cardiometabolic risk factors: a 6-month randomised controlled-feeding trial. Gut 68, 1417–1429. doi: 10.1136/gutjnl-2018-317609
Wang, C. Z., Zhang, C. F., Chen, L., Anderson, S., Lu, F., and Yuan, C. S. (2015). Colon cancer chemopreventive effects of baicalein, an active enteric microbiome metabolite from baicalin. Int. J. Oncol. 47, 1749–1758.
Wang, C., Yang, S., Gao, L., Wang, L., and Cao, L. (2018). Carboxymethyl pachyman (CMP) reduces intestinal mucositis and regulates the intestinal microflora in 5-fluorouracil-treated CT26 tumour-bearing mice. Food Funct. 9, 2695–2704. doi: 10.1039/c7fo01886j
Wang, C.-Z., Huang, W.-H., Zhang, C.-F., Wan, J.-Y., and Wang, Y. (2017). Role of intestinal microbiome in American ginseng-mediated colon cancer protection in high fat diet-fed AOM/DSS mice. Clin. Transl. Oncol. 20, 302–312.
Wang, S. T., Cui, W. Q., Pan, D., Jiang, M., Chang, B., and Sang, L. X. (2020). Tea polyphenols and their chemopreventive and therapeutic effects on colorectal cancer. World J. Gastroenterol. 26, 562–597.
Wang, T., Zhang, L., Wang, P., Liu, Y., Wang, G., Shan, Y., et al. (2022). Lactobacillus coryniformis MXJ32 administration ameliorates azoxymethane/dextran sulfate sodium-induced colitis-associated colorectal cancer via reshaping intestinal microenvironment and alleviating inflammatory response. Eur. J. Nutr. 61, 85–99. doi: 10.1007/s00394-021-02627-8
Wang, X., Liu, Y., Wu, Z., Zhang, P., and Zhang, X. (2022). Tea polyphenols: a natural antioxidant regulates gut flora to protect the intestinal mucosa and prevent chronic diseases. Antioxidants 11:253. doi: 10.3390/antiox11020253
Wang, Y., and Li, H. (2022). Gut microbiota modulation: a tool for the management of colorectal cancer. J. Transl. Med. 20:178.
Wang, Y., Bian, L., Chakraborty, T., Ghosh, T., Chanda, P., and Roy, S. (2019). Construing the biochemical and molecular mechanism underlying the in vivo and in vitro chemotherapeutic efficacy of ruthenium-baicalein complex in colon cancer. Int. J. Biol. Sci. 15, 1052–1071. doi: 10.7150/ijbs.31143
Wang, Y., Zhang, X., Li, J., Zhang, Y., Guo, Y., Chang, Q., et al. (2021). Sini decoction ameliorates colorectal cancer and modulates the composition of gut microbiota in mice. Front. Pharmacol. 12:609992. doi: 10.3389/fphar.2021.60999
Weng, W., and Goel, A. (2020). Curcumin and colorectal cancer: an update and current perspective on this natural medicine. Semin. Cancer Biol. 80, 73–86. doi: 10.1016/j.semcancer.2020.02.011
Windey, K., De Preter, V., Huys, G., Broekaert, W. F., Delcour, J. A., Louat, T., et al. (2015). Wheat bran extract alters colonic fermentation and microbial composition, but does not affect faecal water toxicity: a randomised controlled trial in healthy subjects. Br. J. Nutr. 113, 225–238. doi: 10.1017/S0007114514003523
Wlodarczyk, J., Ploska, M., Ploski, K., and Fichna, J. (2021). The role of short-chain fatty acids in inflammatory bowel diseases and colorectal cancer. Postepy Biochem. 67, 223–230.
Wyatt, M., and Greathouse, K. L. (2021). Targeting dietary and microbial tryptophan-indole metabolism as therapeutic approaches to colon cancer. Nutrients 13, 1189. doi: 10.3390/nu13041189
Xie, S. Z., Liu, B., Ye, H. Y., Li, Q. M., Pan, L. H., Zha, X. Q., et al. (2019). Dendrobium huoshanense polysaccharide regionally regulates intestinal mucosal barrier function and intestinal microbiota in mice. Carbohydr. Polym. 206, 149–162. doi: 10.1016/j.carbpol.2018.11.002
Xu, H., Hiraishi, K., Kurahara, L. H., Nakano-Narusawa, Y., Li, X., Hu, Y., et al. (2021). Inhibitory effects of breast milk-derived Lactobacillus rhamnosus Probio-M9 on colitis-associated carcinogenesis by restoration of the gut microbiota in a mouse model. Nutrients 13:1143. doi: 10.3390/nu13041143
Xue, M., Liang, H., Ji, X., Zhou, Z., Liu, Y., Sun, T., et al. (2020). Effects of fucoidan on gut flora and tumor prevention in 1,2-dimethylhydrazine-induced colorectal carcinogenesis. J. Nutr. Biochem. 82:108396. doi: 10.1016/j.jnutbio.2020.108396
Yammine, A., Namsi, A., Vervandier-Fasseur, D., Mackrill, J. J., Lizard, G., and Latruffe, N. (2021). Polyphenols of the mediterranean diet and their metabolites in the prevention of colorectal cancer. Molecules 26:3483.
Yang, C., Zhao, Y., Im, S., Nakatsu, C., Jones-Hall, Y., and Jiang, Q. (2021). Vitamin E delta-tocotrienol and metabolite 13’-carboxychromanol inhibit colitis-associated colon tumorigenesis and modulate gut microbiota in mice. J. Nutr. Biochem. 89:108567. doi: 10.1016/j.jnutbio.2020.108567
Yang, Q., Zhang, X., Qin, H., Luo, F., and Ren, J. (2022). Phenolic acid profiling of Lactarius hatsudake extracts, anti-cancer function and its molecular mechanisms. Foods 11:1839. doi: 10.3390/foods11131839
Yang, W., Zhao, P., Li, X., Guo, L., and Gao, W. (2022). The potential roles of natural plant polysaccharides in inflammatory bowel disease: a review. Carbohydr. Polym. 277:118821.
Yoon, L. S., and Michels, K. B. (2021). Characterizing the effects of calcium and prebiotic fiber on human gut microbiota composition and function using a randomized crossover design-a feasibility study. Nutrients 13:1937. doi: 10.3390/nu13061937
Yu, Y. N., Yu, T. C., Zhao, H. J., Sun, T. T., Chen, H. M., Chen, H. Y., et al. (2015). Berberine may rescue Fusobacterium nucleatum-induced colorectal tumorigenesis by modulating the tumor microenvironment. Oncotarget 6, 32013–32026. doi: 10.18632/oncotarget.5166
Yu, Y., Shen, M., Song, Q., and Xie, J. (2018). Biological activities and pharmaceutical applications of polysaccharide from natural resources: a review. Carbohydr. Polym. 183, 91–101.
Yu, Z., Song, G., Liu, J., Wang, J., Zhang, P., and Chen, K. (2018). Beneficial effects of extracellular polysaccharide from Rhizopus nigricans on the intestinal immunity of colorectal cancer mice. Int. J. Biol. Macromol. 115, 718–726. doi: 10.1016/j.ijbiomac.2018.04.128
Yuan, X., Xue, J., Tan, Y., Yang, Q., Qin, Z., Bao, X., et al. (2021). Albuca bracteate polysaccharides synergistically enhance the anti-tumor efficacy of 5-fluorouracil against colorectal cancer by modulating beta-catenin signaling and intestinal flora. Front. Pharmacol. 12:736627. doi: 10.3389/fphar.2021.736627
Zhang, H., Hui, D., Li, Y., Xiong, G., and Fu, X. (2019). Canmei formula reduces colitis-associated colorectal carcinogenesis in mice by modulating the composition of gut microbiota. Front. Oncol. 9:1149. doi: 10.3389/fonc.2019.01149
Zhang, J., Zhang, H., Li, F., Song, Z., Li, Y., and Zhao, T. (2020). Identification of intestinal flora-related key genes and therapeutic drugs in colorectal cancer. BMC Med. Genomics 13:172. doi: 10.1186/s12920-020-00810-0
Zhang, S. L., Mao, Y. Q., Zhang, Z. Y., Li, Z. M., Kong, C. Y., Chen, H. L., et al. (2021). Pectin supplement significantly enhanced the anti-PD-1 efficacy in tumor-bearing mice humanized with gut microbiota from patients with colorectal cancer. Theranostics 11, 4155–4170. doi: 10.7150/thno.54476
Zhang, Z., Chen, H., Xu, C., Song, L., Huang, L., Lai, Y., et al. (2016). Curcumin inhibits tumor epithelialmesenchymal transition by downregulating the Wnt signaling pathway and upregulating NKD2 expression in colon cancer cells. Oncol. Rep. 35, 2615–2623. doi: 10.3892/or.2016.4669
Zhao, Y., and Jiang, Q. (2021). Roles of the polyphenol-gut microbiota interaction in alleviating colitis and preventing colitis-associated colorectal cancer. Adv. Nutr. 12, 546–565. doi: 10.1093/advances/nmaa104
Zheng, D. W., Li, R. Q., An, J. X., Xie, T. Q., Han, Z. Y., Xu, R., et al. (2020). Prebiotics-encapsulated probiotic spores regulate gut microbiota and suppress colon cancer. Adv. Mater. 32:e2004529. doi: 10.1002/adma.202004529
Zheng, Y., You, X., Guan, S., Huang, J., Wang, L., Zhang, J., et al. (2019). Poly(Ferulic Acid) with an anticancer effect as a drug nanocarrier for enhanced colon cancer therapy. Adv. Funct. Mater. 29:1808646.
Zhong, L., Zhang, X., and Covasa, M. (2014). Emerging roles of lactic acid bacteria in protection against colorectal cancer. World J. Gastroenterol. 20, 7878–7886.
Zhou, X., Chen, C., Zhong, Y. N., Zhao, F., Hao, Z., Xu, Y., et al. (2020). Effect and mechanism of vitamin D on the development of colorectal cancer based on intestinal flora disorder. J. Gastroenterol. Hepatol. 35, 1023–1031. doi: 10.1111/jgh.14949
Zhu, H. C., Jia, X. K., Fan, Y., Xu, S. H., Li, X. Y., Huang, M. Q., et al. (2021). Alisol B 23-acetate ameliorates azoxymethane/dextran sodium sulfate-induced male murine colitis-associated colorectal cancer via modulating the composition of gut microbiota and improving intestinal barrier. Front. Cell Infect. Microbiol. 11:640225. doi: 10.3389/fcimb.2021.640225
Zhu, Q., Jin, Z., Wu, W., Gao, R., Guo, B., Gao, Z., et al. (2014). Analysis of the intestinal lumen microbiota in an animal model of colorectal cancer. PLoS One 9:e90849. doi: 10.1371/journal.pone.0090849
Zhu, Y., Michelle Luo, T., Jobin, C., and Young, H. A. (2011). Gut microbiota and probiotics in colon tumorigenesis. Cancer Lett. 309, 119–127.
Zhu, Y., Shi, X., Lin, X., Ye, K., Xu, X., Li, C., et al. (2017). Beef, chicken, and soy proteins in diets induce different gut microbiota and metabolites in rats. Front. Microbiol. 8:1395. doi: 10.3389/fmicb.2017.01395
Keywords: gut microbiota, colorectal cancer, diet, probiotic, prebiotic
Citation: Chen M, Lin W, Li N, Wang Q, Zhu S, Zeng A and Song L (2022) Therapeutic approaches to colorectal cancer via strategies based on modulation of gut microbiota. Front. Microbiol. 13:945533. doi: 10.3389/fmicb.2022.945533
Received: 16 May 2022; Accepted: 14 July 2022;
Published: 04 August 2022.
Edited by:
Hui Zhang, South China Agricultural University, ChinaReviewed by:
Shenhai Gong, Southern Medical University, ChinaBahman Mirzaei, Zanjan University of Medical Sciences, Iran
Dengke Yin, Anhui University of Chinese Medicine, China
Copyright © 2022 Chen, Lin, Li, Wang, Zhu, Zeng and Song. This is an open-access article distributed under the terms of the Creative Commons Attribution License (CC BY). The use, distribution or reproduction in other forums is permitted, provided the original author(s) and the copyright owner(s) are credited and that the original publication in this journal is cited, in accordance with accepted academic practice. No use, distribution or reproduction is permitted which does not comply with these terms.
*Correspondence: Linjiang Song, songlinjiang@cdutcm.edu.cn; Anqi Zeng, zeng6002aq@163.com