- 1Departamento de Microbiología, Escuela Nacional de Ciencias Biológicas, Instituto Politécnico Nacional, Mexico City, Mexico
- 2Departamento de Zoología, Escuela Nacional de Ciencias Biológicas, Instituto Politécnico Nacional, Mexico City, Mexico
- 3Institute of Agricultural Resources and Regional Planning, Chinese Academy of Agricultural Sciences, Beijing, China
- 4State Key Laboratory for Agro-Biotechnology and Ministry of Agriculture Key Lab of Soil Microbiology, College of Biological Sciences, China Agricultural University, Beijing, China
Species belonging to the genus Rahnella are dominant members of the core gut bacteriome of Dendroctonus-bark beetles, a group of insects that includes the most destructive agents of pine forest in North and Central America, and Eurasia. From 300 isolates recovered from the gut of these beetles, 10 were selected to describe an ecotype of Rahnella contaminans. The polyphasic approach conducted with these isolates included phenotypic characteristics, fatty acid analysis, 16S rRNA gene, multilocus sequence analyses (gyrB, rpoB, infB, and atpD genes), and complete genome sequencing of two isolates, ChDrAdgB13 and JaDmexAd06, representative of the studied set. Phenotypic characterization, chemotaxonomic analysis, phylogenetic analyses of the 16S rRNA gene, and multilocus sequence analysis showed that these isolates belonged to Rahnella contaminans. The G + C content of the genome of ChDrAdgB13 (52.8%) and JaDmexAd06 (52.9%) was similar to those from other Rahnella species. The ANI between ChdrAdgB13 and JaDmexAd06 and Rahnella species including R. contaminans, varied from 84.02 to 99.18%. The phylogenomic analysis showed that both strains integrated a consistent and well-defined cluster, together with R. contaminans. A noteworthy observation is the presence of peritrichous flagella and fimbriae in the strains ChDrAdgB13 and JaDmexAd06. The in silico analysis of genes encoding the flagellar system of these strains and Rahnella species showed the presence of flag-1 primary system encoding peritrichous flagella, as well as fimbriae genes from the families type 1, α, β and σ mainly encoding chaperone/usher fimbriae and other uncharacterized families. All this evidence indicates that isolates from the gut of Dendroctonus-bark beetles are an ecotype of R. contaminans, which is dominant and persistent in all developmental stages of these bark beetles and one of the main members of their core gut bacteriome.
Introduction
The genus Rahnella belongs to the family Yersiniaceae and comprises 14 taxonomically valid species1: Rahnella aquatilis (Izard et al., 1979), R. bruchi, R. inusitata, R. victoriana, R. variigena, R. woolbedingensis (Brady et al., 2014), R. aceris (Lee et al., 2020), R. contaminans, R. laticis (Jeon et al., 2021), R. bonaserana, R. ecdela, R. perminowiae, R. rivi (Brady et al., 2022), and R. sikkimica (Kumar et al., 2022). Rahnella species reside in a broad range of environments such as soil (Kim et al., 1997), seeds (Kämpfer, 2005), phloem and bark (Gonzalez-Escobedo et al., 2018), roots (Izumi et al., 2006), water (Izard et al., 1979), food (Kämpfer, 2005), human clinical samples (Alballaa et al., 1992), and extremophile environments (Mukhia et al., 2022). In addition, several studies have revealed the presence of this genus in association with insects such as bark beetles (Briones-Roblero et al., 2017a; Fabryová et al., 2018), cockroaches (Akbari et al., 2014), honeybees (Kačániová et al., 2020), and mosquitoes (Charan et al., 2013).
The bark beetles Dendroctonus spp. (Curculionidae: Scolytinae) are phloeophagous insects that spend most of their life cycle under the tree’s bark and are one of the most destructive pine forest agents in North and Central America, and Eurasia (Six and Bracewell, 2015). Rahnella strains have been isolated from the exoskeleton, galleries, and guts of these and other bark beetles (Morales-Jiménez et al., 2013; Durand et al., 2015; Mason et al., 2015; Hernández-García et al., 2017; Briones-Roblero et al., 2017a,b; Fabryová et al., 2018; Chakraborty et al., 2020). Rahnella is a member of the core gut bacteriome of Dendroctonus spp. (Hernández-García et al., 2017), and it has been persistently reported in all developmental stages of Dendroctonus rhizophagus (Briones-Roblero et al., 2017a). Furthermore, it has been shown that Rahnella strains from bark beetles play a fundamental role in the digestive and detoxification processes, since they are capable of degrading different substrates such as esters, lipids, and xylan (Briones-Roblero et al., 2017b; Pineda-Mendoza et al., 2022), recycling uric acid (Morales-Jiménez et al., 2013), and degrading or transforming different monoterpenes of the host trees of these insects (Boone et al., 2013; Xu et al., 2015, 2016).
In this study, Rahnella isolates associated with the gut of Dendroctonus-bark beetles were characterized following a polyphasic approach based on genotypic, phenotypic, genomic, and fatty acid assays. Our results show that these strains are an ecotype of the nominal species Rahnella contaminans, whose capabilities and ecological role in benefit of these insects are being explored.
Materials and methods
Isolation and preliminary molecular identification
Bacteria were isolated from 10 homogenized gut sets from larvae, pupae and adults of some Dendroctonus species collected in different geographical sites in Mexico. Prior to dissection, insects were superficially disinfected according to Briones-Roblero et al. (2017a). Insects were grouped and processed by location; thus, individuals of the same species from different locations were not mixed. Guts were homogenized with sterile polyethylene pestles into Eppendorf tubes containing phosphate-buffered saline (PBS) solution. Thereafter, serially diluted solutions were prepared and spread onto tryptic soy agar (TSA; 15 g L−1 of tryptone, 5 g L−1 of soytone, 5 g L−1 of sodium chloride, and 15 g L−1 of agar; BD Difco, United States) plates, which were incubated at 28°C for 48 h. Individual colonies were subsequently streaked on TSA plates and incubated under previous conditions. A total of 500 isolates were recovered from the gut of Dendroctonus species, which were stored in a sterile 30% (v/v) glycerol solution at −70°C.
Bacterial DNA was extracted from axenic colonies following the method reported by Hoffman and Winston (1987). Amplifications of the 16S rRNA gene were performed according to Briones-Roblero et al. (2017b). Amplicons were purified with GeneJET PCR Purification Kit (Thermo Fisher Scientific, Waltham, MA), and then sequenced in Macrogen Inc. (Seoul, Korea). All sequences were compared with sequences deposited in GenBank and EzBioCloud2 databases. A total of 300 sequences from our isolates matched with those from Rahnella species. These sequences and other phylogenetically closely related species were aligned using Clustal X v.2.1 (Larkin et al., 2007). A phylogenetic characterization based on the 16S rRNA gene was done using maximum likelihood in PhyML.3 The best nucleotide substitution model was determined using jModelTest v.2.1.7 (Darriba et al., 2012) under the Akaike information criterion. The nodes’ reliability was evaluated using a bootstrap test after 1,000 pseudoreplications.
Based on the 16S rRNA gene phylogeny of 300 isolates (Figure 1), 10 Rahnella strains were selected for their physiological and molecular characterization (Table 1). The strains ChDrAdgB13 (accession number CDBB-2057 = NRRL B-65604) and JaDmexAd06 were selected for genome sequencing and observation of morphological characters.
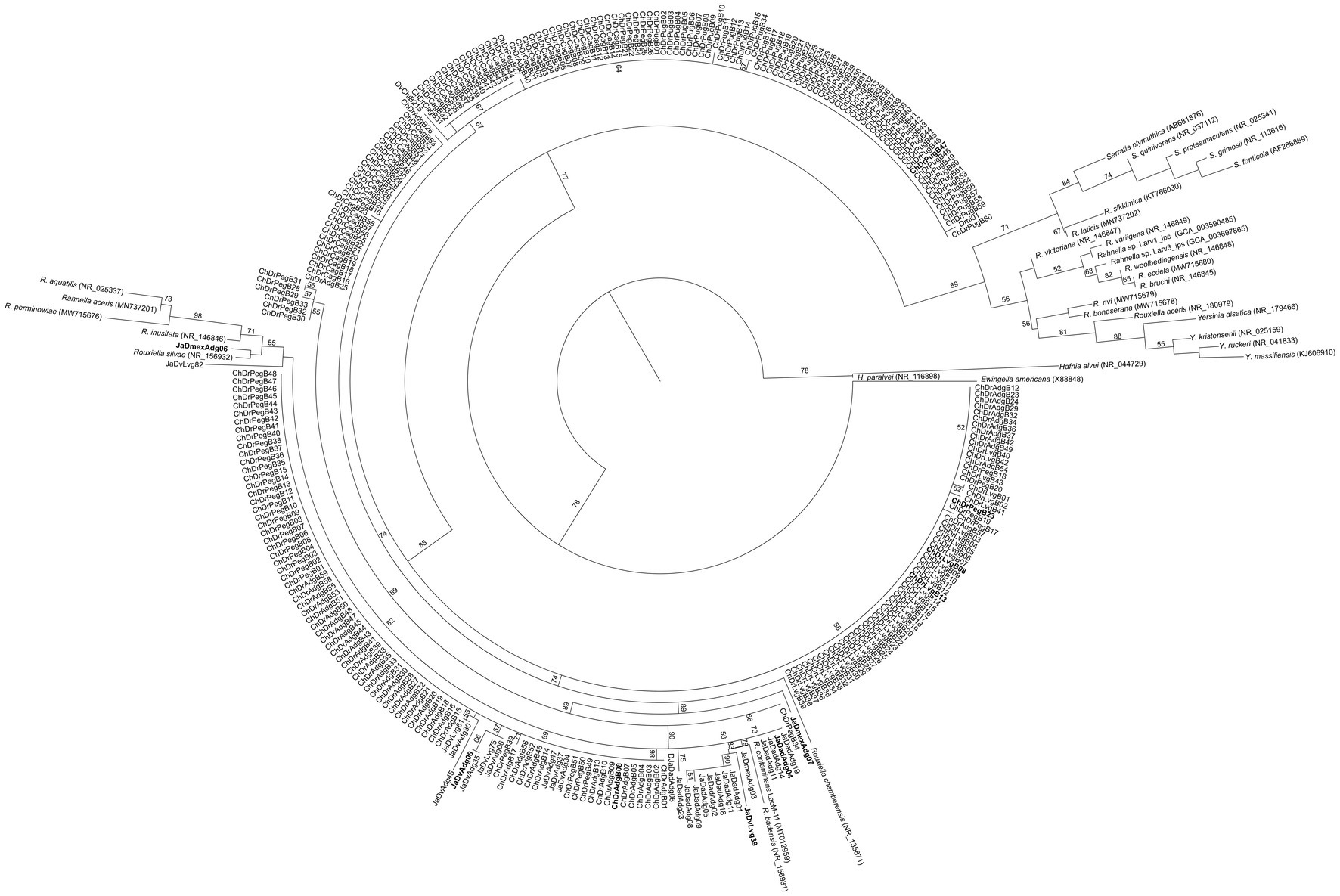
Figure 1. Maximum likelihood phylogeny of the 16S rRNA sequences of 300 Rahnella isolates and type strains of the genus Rahnella. The nucleotide substitution model used was GTR with a gamma parameter of 0.693. Bootstrap values >50% are shown at nodes. In bold are the sequences selected for other analyses in this study.
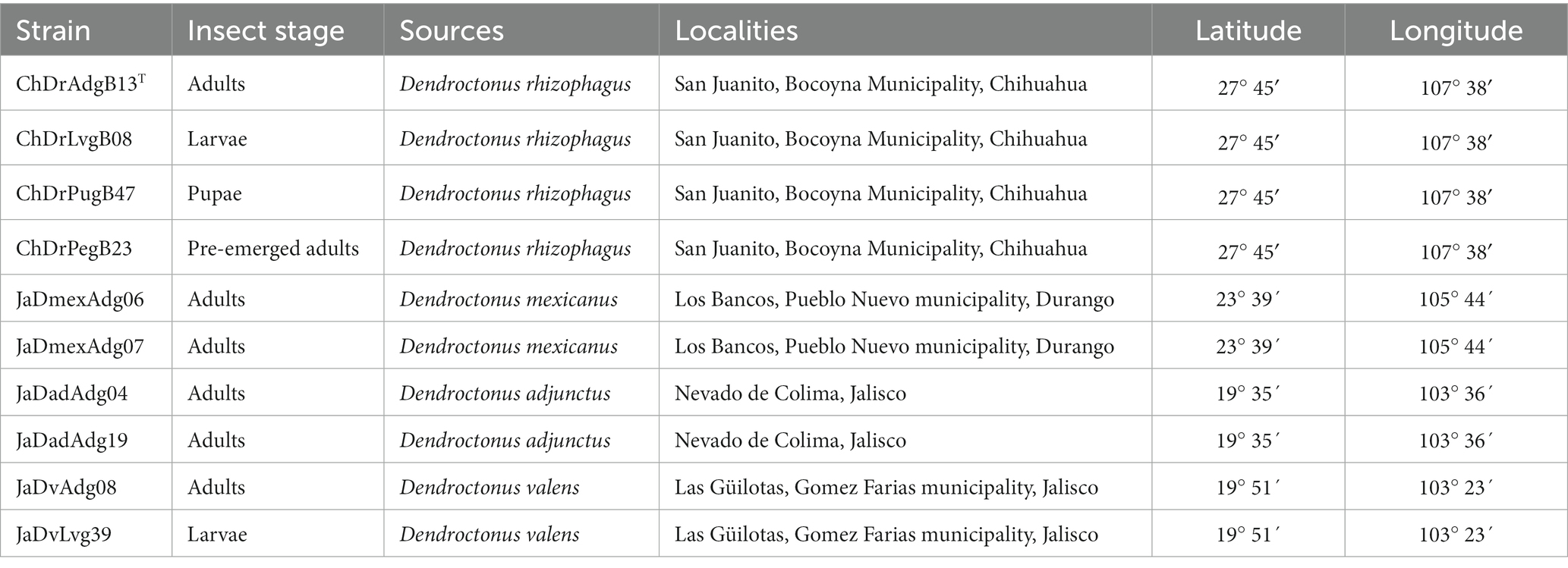
Table 1. Bacterial isolates used in this study, Dendroctonus species, localities, and geographic references where they were collected.
Phenotypic characterization
Ten isolates were cultured on TSA at 28°C for 48 h. Cellular morphology was observed in detail by transmission electron microscopy (JEOL JEM-2100) after negative staining with phosphotungstic acid hydrate (Sigma-Aldrich, United States). Gram staining was performed using the Gram stain kit (bioMérieux, Inc., United States) according to the manufacturer’s instructions. Oxidase and catalase activities were determined with N,N-dimethyl-p-phenylenediamine dihydrochloride and 3% (v/v) H2O2 solutions, respectively. Strains were cultured on TSA plates at 4, 10, 20, 28, 37, and 44°C to determine the growth temperature range for 48 h. Tryptic soy broth (TSB, Difco) medium was used to test the growth capability of the strains at different pH values. The medium was adjusted to a final pH of 4–10 using NaOH and HCI 1.0 M. Cultures were incubated at 28°C for up to 48 h. Growth was determined by measuring the absorbance at 600 nm using a Multiskan™ GO Microplate Spectrophotometer (Thermo Fisher Scientific, Waltham, MA, United States). The physiological and biochemical capacities of the strains were evaluated visually using the API 50CH and API 20E systems (bioMérieux Inc., United States) and Biolog GN2 Microplates (Biolog, Inc., United States) following the manufacturers’ instructions, and adjusting the inoculum to 95% T in GN/GP Inoculating Fluid (Biolog, Inc., United States) and incubated at 28°C for 24–48 h.
Chemotaxonomic analysis
For the determination of cellular fatty acids, the isolates were grown in TSA at 28°C for 24 h. The extraction and methylation of fatty acids were performed according to the standard protocol of the Sherlock Microbial Identification System (MIS) (Sasser, 1990), and later identified and quantified using MIDI System software (MIDI Inc., United States).
Genotypic characterization
16S rRNA gene and multilocus sequence analysis (MLSA)
The bacterial DNA of 10 isolates was extracted from axenic colonies following the method reported by Hoffman and Winston (1987). Phylogenetic inference analysis of these isolates was carried out as described above, including all nominal species of Rahnella and some species of the family Yersiniaceae. The 16S rRNA gene sequence pairwise similarity among these taxa was estimated in MatGAT v.2.01 (Campanella et al., 2003). In contrast, the amplification and partial sequencing of gyrB, rpoB, infB, and atpD housekeeping genes (MLSA) were carried out according to Brady et al. (2008, 2014). PCR products were purified with a GeneJET PCR Purification Kit (Thermo Scientific, Waltham, MA) according to the manufacturer’s protocol and sequenced in Macrogen Inc. (Seoul, Korea). All sequences were compared to those available from the GenBank database.4 For phylogenetic analysis, sequences of the housekeeping genes, including sequences of phylogenetically close bacteria species, were aligned independently in Clustal X v.2.1 and edited in Seaview v.5.0.4 (Gouy et al., 2010). Sequences of these genes were concatenated to perform a maximum likelihood phylogenetic analysis in PhyML.5 The best nucleotide substitution model for concatenated sequences was determined using jModelTest v.2.1.7 under the Akaike information criterion. The nodes’ reliability was evaluated by means of a bootstrap test after 1,000 pseudoreplications. Housekeeping gene sequences were deposited in the GenBank database under the accession number (MW648385–MW648424).
Genome analysis
The isolatesxs ChDrAdgB13 and JaDmexAd06 were grown overnight in 10 ml of TSA broth at 28°C, with orbital shaking at 150 rpm. The genomic DNA was isolated using DNeasy® Blood and Tissue kit according to the manufacturer’s protocol (QIAGEN, United States). The whole-genome sequencing was performed on the HiSeq2000 Illumina platform by Otogenetics Corporation (Norcross, GA, United States). The paired-end data were quality checked using FastQC v.0.11.3 (Babraham Institute, Cambridge, United Kingdom). Reads were filtered using a sliding window quality cutoff of Q4:15 in Trimmomatic v.036 (Bolger et al., 2014), and then high-quality reads were de novo assembled using Velvet v.1.2.10 (Zerbino and Birney, 2008). Genome completeness and potential contamination were estimated using RAST server v.2.06 and KBase,7 respectively. Protein, rRNA, and tRNA-coding genes were identified using Prokka v.2.88 (Seemann, 2014) and the Kyoto Encyclopedia of Genes and Genomes (KEGG) Automatic Annotation Server (KAAS).9 The genome sequences were submitted to GenBank under the BioProject number JAEMGT000000000 for ChDrAdgB13 and JARBHC000000000 for JaDmexAd06.
The G + C content of DNA was determined directly from the complete genome. The average nucleotide identity (ANI) and digital DNA–DNA hybridization (dDDH), using formula d4 among genomes of two strains and Rahnella species, were estimated with the ANI Calculator at Kostas lab10 and GGDC 2.1,11 using the recommended settings in both servers, respectively.
Phylogenomic analysis
Genome-based phylogeny from the isolates ChDrAdgB13, JaDmexAd06, nominal species of Rahnella, and other related species of Yersiniaceae was performed using the Type Strain Genome Server (TYGS)12 (Meier-Kolthoff and Göker, 2019). The phylogenomic tree was inferred using FastME13 (Lefort et al., 2015) and Genomic BLAST Distance Phylogeny (GBDP), which provides pairwise intergenomic distances generated using algorithm “trimming” and the distance formula d4 (Meier-Kolthoff et al., 2013). The robustness of the relationships was evaluated with a bootstrap test after 100 pseudoreplicates. The tree was visualized using FigTree v.1.4.4 (Rambaut, 2012).
Flagellar characterization
Given that there is a discrepancy between the type of flagella, peritrichous, or amphitrichous (polar), presented by the species of the genus Rahnella (Jeon et al., 2021; Brady et al., 2022), observations by TEM of isolates ChDrAdgB13 and JaDmexAd06 were performed to clarify the flagella arrangement of these isolates. In addition, based on the genomes of the 14 taxonomically valid species of Rahnella, we performed a comprehensive in silico analysis of genes encoding flagella. The annotation was performed using the Bacterial and Viral Bioinformatics Resource Center (BV-BRC) v.3.28.914 and RAST server.
Results
Phenotypic features
The colonies were white, round, and convex with the entire margin being smooth and bright. They did not produce a diffusible pigment on the TSA medium after incubation at 28°C for 48 h. The cells were Gram-negative rods (0.4–0.6 × 2–3.5 μm), motile with fimbriae, and peritrichous flagella (Figure 2). The growth occurred in a temperature range of 20–28°C and a pH range from 4 to 10, with an optimum temperature of 28°C and a pH 7.
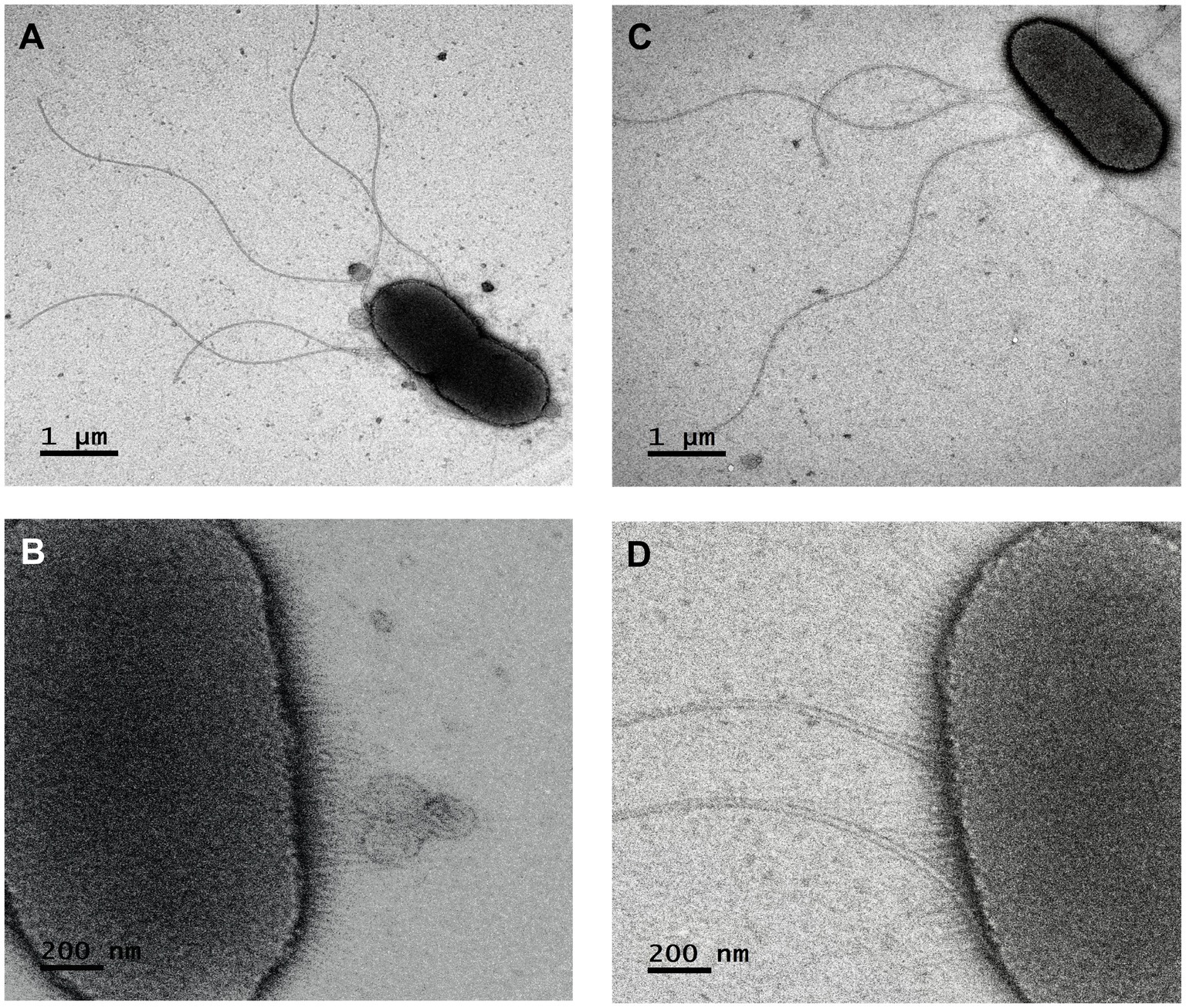
Figure 2. Scanning electron micrograph of the Rahnella contaminans ecotype. (A,B) flagella and fimbriae of strain ChDrAdgB13, and (C,D) of strain JaDmexAd06 (4,000×).
The phenotypic characteristics of 10 isolates were similar in acid production and enzyme activities, but different in the carbon sources assimilated (Supplementary Table S1). Comparison of the phenotypic characteristics of the strains ChDrAdgB13 and JaDmexAd06, and type strains of Rahnella species were different in acid production and carbon sources assimilated, but similar in the enzyme activities except in the activity tryptophan deaminase (TDA) (Supplementary Table S1).
Chemotaxonomic analysis
The cellular fatty acids of the 10 isolates were similar to those of the type strains of the Rahnella genus. The main fatty acid components of 10 isolates were similar among them [C12:0 (2.8–2.9%), C14:0 (5.5–5.6%), C16:0 (26.4–26.6%), C17:0 cyclo (22.7%), iso-C16:1, and/or C14:0 3-OH (8.3–8.4% summed feature 2), and C16:1 ω7c and/or C16:1ω6c (15.4–15.5%, summed feature 3)] (Supplementary Table S2). Unfortunately, this comparison was not possible to perform with R. contaminans and R. laticis because both species were grown under anoxic conditions.
Molecular characterization
The phylogenetic inference analysis with the 16S rRNA gene showed that the 10 selected isolates are distributed in a consistent clade (bootstrap value >70%) with species of Rouxiella and Rahnella including R. contaminans, R. inusitata, R. aceris, R. aquatilis, R. perminowiae, and some Rouxiella species. The rest of Rahnella type species formed another clade separate from it (Supplementary Figure S1). Pairwise similarity values were higher among 10 isolates and R. contaminans (≥99.92%) than between the isolates and type strains of Rahnella species (97.32–99.75%) (Supplementary Table S3).
The maximum likelihood phylogenetic tree inferred using concatenated housekeeping genes showed that the 10 selected isolates and R. contaminans formed an independent cluster (bootstrap value >97%) close to R. laticis within the genus clade (Figure 3). Pairwise similarity values of concatenated housekeeping genes were higher, also among the 10 isolates and R. contaminans (98.98–99.67%), than between isolates and other Rahnella species (91.20–98.88%) (Supplementary Table S3).
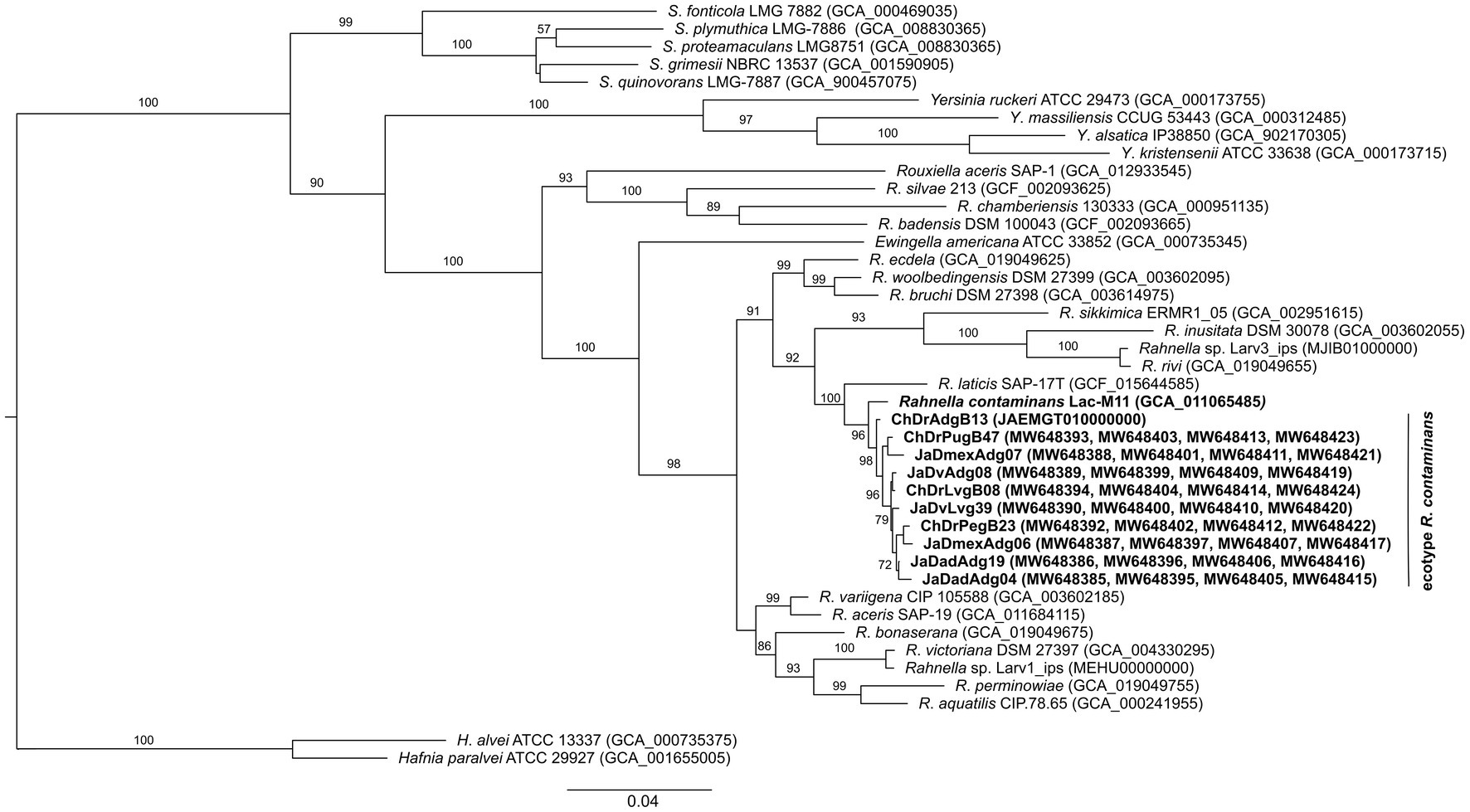
Figure 3. Maximum likelihood phylogenetic tree based on concatenated gyrB, rpoB, infB, and atpD gene sequences of 10 isolates and the type strains of the Rahnella species. The nucleotide substitution model was GTR + R according to Akaike criterion. Bootstrap values >50% after 1,000 pseudoreplicates are shown at nodes.
Genomic features
The assembled genomes of strains ChDrAdgB13 and JaDmexAd06 were 5.74 Mb (5,732,748 bp) in length, consisting of 257 contigs with a N50 length of 0.58 Mbp, including only one copy of 16S rRNA, 54 tRNAs, 538 duplicate genes, and 26 functional categories based on the KEGG pathway database; for the second, of 5.54 Mb (5,544,148 bp) in length, 67 contigs with a N50 of 0.78 Mbp, one copy 16S rRNA, 70 tRNAs, 511 duplicate genes, and 26 functional categories. CheckM-estimated completeness for both genomes was 99.98% for ChDrAdgB13 and 94.54% for JaDmexAd06. The coverage depth of these strains was 104x for ChDrAdgB13 and 100x for JaDmexAd06. The genomic DNA G + C content for ChDrAdgB13 and JaDmexAd06 was similar to R. contam inans (53.1%) and other Rahnella species whose percentage varied from 51.9% (R. bruchi DSM 27398T) to 53.7% (R. victoriana DSM 27397T). The pairwise ANI values of the strains ChDrAdgB13 and JaDmexAd06, with respecto to Rhanella species, varied from 84.02–84.07% (R. inusitada DSM 30038T) to 99.13–99.18% (R. contaminans LacM11T)(Table 2), meanwhile dDDH values of both strains respect to R. contaminans was >93.0, but <60.0 (27.5–60.9) with respect other Rahnella species (Table 2). The phylogenomic tree (Figure 4) showed that both strains integrate, together with R. contaminans, a consistent and well-defined cluster (98% bootstrap value) within the Rahnella genus.
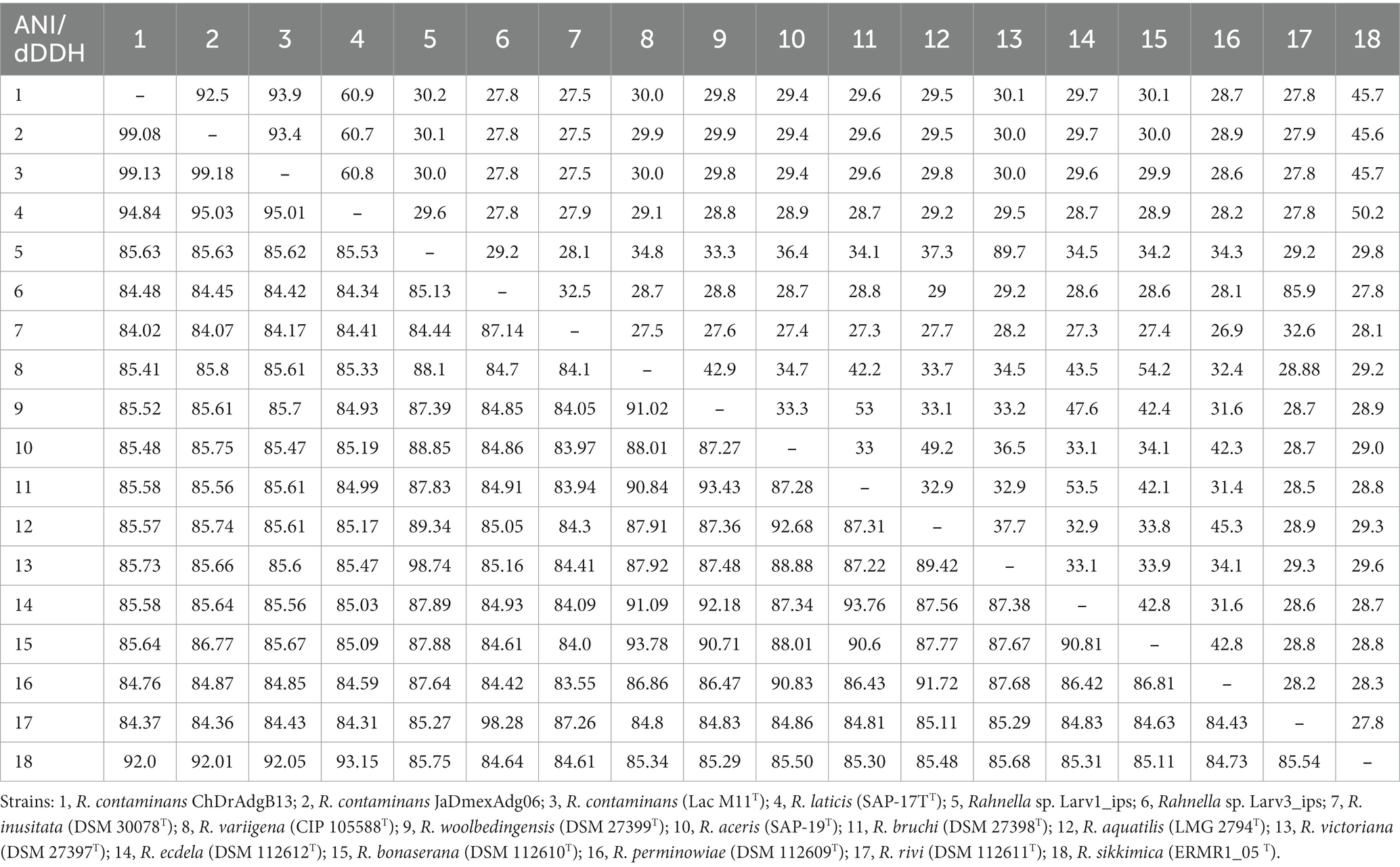
Table 2. Pairwise average nucleotide identity (ANI, bottom left) and digital DNA-DNA hybridization (d4DDH, top right) among ChDrAdgB13 and JaDmexAdg06 strains, and nominal Rahnella species.
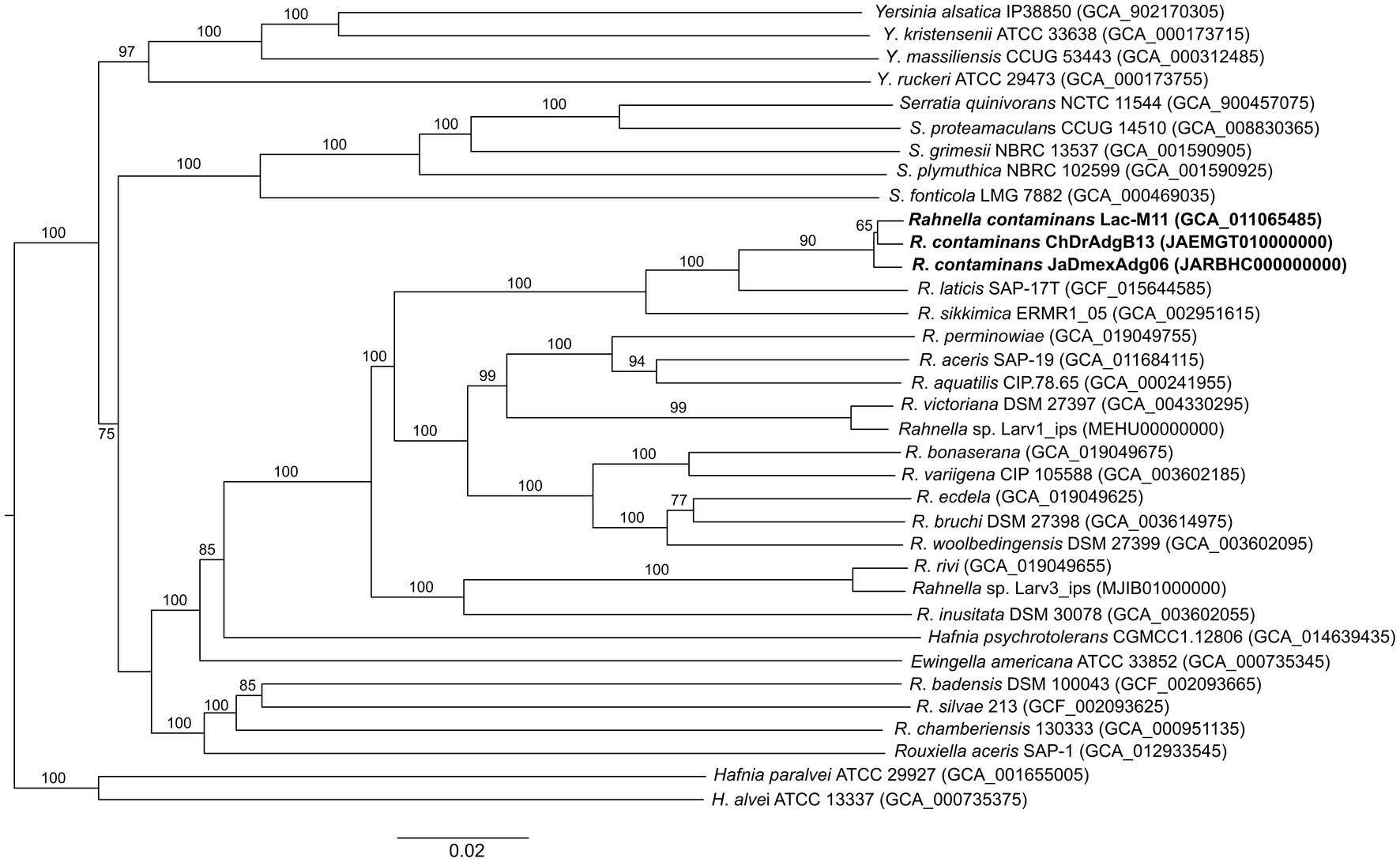
Figure 4. Phylogenetic tree of all Rahnella nominal species, including ChDrAdgB13 and JaDMexAdg06 strains. The tree was constructed using GBDP distances among genomes. Bootstrap values >57% are shown at the nodes.
The overview of genomes of both strains showed genes associated with carbohydrate metabolism (17.29–17.47%), amino acids and derivatives (12.6–12.7%), cofactors, vitamins, prosthetic groups and pigments (6.8–6.9%), protein metabolism (6.38–6.46%), cell wall and capsule (5.75–6.05%), membrane transport (5.5–6.7%), RNA metabolism (5.3–5.37%), stress response (4.57–4.89%), and detoxification (4%). The genomes of R. contaminans and R. laticis show similar percentages to R. contaminans ecotype strains in these and other KEGG-functional categories, except for protein metabolism where a higher number of genes were found in both species (7.27% R. contaminans and 6.86% R. laticis), and a lower number of genes for carbohydrate degradation in R. laticis (16.48%) (Figure 5 and Supplementary Table S4).
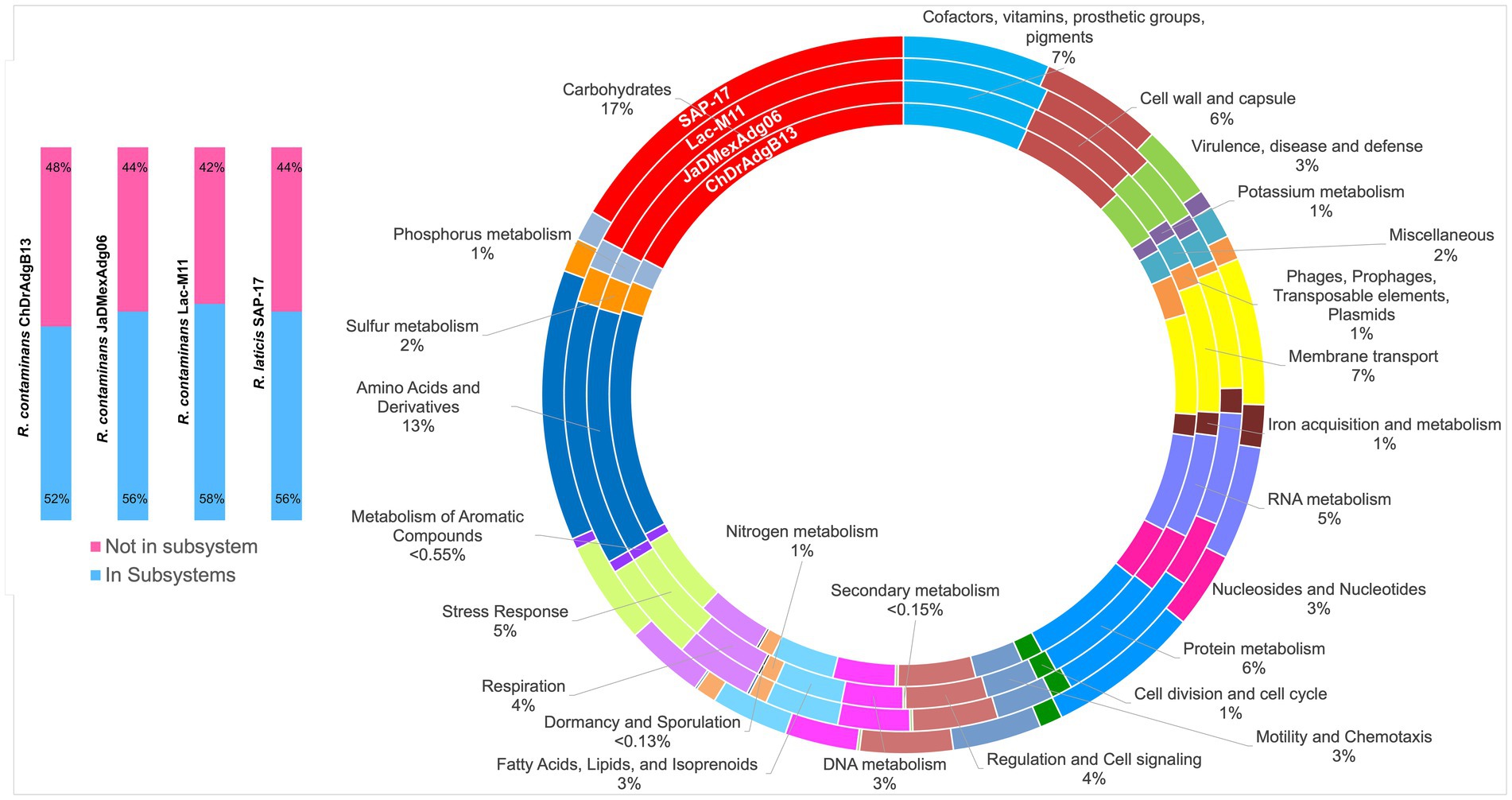
Figure 5. Genome features of Rahnella contaminans ecotype (ChDrAdgB13 and JaDMexAdg06 strains) and, type strains R. contaminans and R. laticis. The circles show the 26 functional categories annotated in Rapid Annotation System Technology Server. The blue color corresponds to the percentage of proteins associated with subsystems, and the pink to proteins not associated.
In particular, the genomes of ChDrAdgB13 and JaDmexAd06 have genes involved in xylan hydrolysis (endo-1,4-β-xylanase, α-xylosidases, α-L-arabinofuranosidase and a partial sequence of one β-xylosidase) and other carbohydrates such as cellulose (β-glucosidases) and starch (cytoplasmic α-amylase, α-glucosidases, and β-glucosidases), which might contribute to the nutrition of host insects. These same genes were found in the genomes of R. contaminans and in R. laticis.
The in silico analysis of the flagellar system of the strains ChDrAdgB13, JaDmexAd06, and Rahnella species showed the presence of flag-1 primary system encoding for peritrichous flagella (Supplementary Table S5). This system is integrated by 50 genes encoding proteins involved in the biosynthesis, regulation, maintenance, and chemotaxis of this flagella type. The only exceptions are R. variigena (CIP 105588), which incorporates a second system, the flag-3, which also encodes for peritrichous flagella, and R. laticis (SAP-17), which presents the fla-A gene, a member of the flag-5 system encoding for amphitrichous (polar) flagella (Supplementary Table S5).
Discussion
The genus Rahnella includes species capable of living in diverse aquatic and terrestrial habitats (Izard et al., 1979; Brady et al., 2014; Lee et al., 2020; Jeon et al., 2021; Brady et al., 2022), including extremophile environments (Kumar et al., 2022). In this study, a comprehensive attempt to clarify the taxonomic status of Rahnella isolates associated with the gut of Dendroctonus-bark beetles was carried out using phenotypic, molecular, and phylogenetic evidence.
Based on the recommended criteria in the International Code of Nomenclature of Bacteria for naming species, genomospecies, or subspecies (Parker et al., 2019), the differences observed between isolates and nominal species of Rahnella do not allow arguing in favor of some of these categories for our isolates. Yet, ANI and dDDH values and phylogenetic inference analyses with the 16S rRNA, MLSA, and genomes indicate that the gut-associated Rahnella of these beetles is an ecotype of R. contaminans.
Rahnella contaminans was isolated and described as a contaminant of refrigerated MRSA agar plates after the isolation of Lactobacillus strains using raw rice wine; however, re-isolation of strains of this species using the same isolation conditions was not possible (Jeon et al., 2021). By contrast, R. contaminans ecotype associated with Dendroctonus species has been recurrently isolated from the gut of these bark beetles; its persistence across the different development stages of these insects and their geographical localities (Hernández-García et al., 2017; Briones-Roblero et al., 2017a,b) indicates that it is a persist symbiont whose isolation source and habitat are common. The bark beetles’ gut is a distinctive habitat by its morphology, toxic environment, and physicochemical properties, which influence and determine, in combination with the interactions between microbes and between these and their host, the species that make up the microbial assemblage.
Considering that the sources of isolation were very different, it was amazing to find a great phenotypic similarity and close phylogenetic relationship between this ecotype and R. contaminans. Yet, various aspects must be highlighted. The first is that MLSA and phylogenomic analyses, either with whole genomes or with the core genome, recover the genus Rahnella as a monophyletic group independent of other genera of the family Yersiniaceae, where R. contaminans and the ecotype integrate a well-defined cluster (Figures 3, 4). This group was also recovered with 16S rRNA, despite low phylogenetic resolution (Supplementary Figure S1). Second, the flagellar system of ChDrAdgB13, JaDmexAd06 and all Rahnella species is flag-1, except in R. variigena which in addition to flag-1 also has flag-3; yet, both systems encode for peritrichous flagella (Supplementary Table S5). This result is in agreement with the type of flagellum observed in the ecotype by TEM and with observations made in Rahnella species in other studies, which report the same flagella type (Izard et al., 1979; Brady et al., 2014, 2022; Kumar et al., 2022). However, Lee et al. (2020) and Jeon et al. (2021) reported and showed images of amphitrichous flagella in R. contaminans, R. aceris, and R. laticis, suggesting that all Rahnella species have this type of flagella. This consideration does not agree with the flagellar loci present in the genome of R. contaminans, as well as other Rahnella species, or with the peritrichous flagella observed in the ecotype and Rahnella species. Finally, a noteworthy observation in this study is the presence of fimbriae in the ecotype (Figure 2), which could be an adaptation to the intestinal environment. Fimbriae are mainly involved in adhesion to different inerts or living surfaces, which favors colonization (Thanassi et al., 2007). In the gut, these structures could help bacteria to adhere to epithelial cells, which is essential for the colonization or invasion of host tissue. This explains the dominance and persistence of this ecotype along all developmental stages in bark beetles (Briones-Roblero et al., 2017a). Unfortunately, this feature has been overlooked in the description of Rahnella species, which does not allow us to know whether these structures are expressed by all species, regardless of growth conditions or habitat. Yet, an overview of the genome of Rahnella species showed that they have fimbriae genes from the family types 1, α, β, and σ mainly encoding chaperone/usher fimbriae, as well as other uncharacterized families (Thanassi et al., 2007; Supplementary Table S6).
In brief, this study shows that Rahnella isolates associated with the gut of Dendroctonus-bark beetles are an ecotype of R. contaminans. This ecotype is a recurrent, dominant, and persistent symbiont in the gut, which plays a fundamental ecological role in the benefit of these bark beetles, due to its capacity to hydrolyze esters, lipids, and xylans (Briones-Roblero et al., 2017b; Pineda-Mendoza et al., 2022), as well as recycle uric acid (Morales-Jiménez et al., 2013), and degrade or transform different toxic monoterpenes for insects (Boone et al., 2013; Xu et al., 2015, 2016).
Emended description of the Rahnella contaminans (Jeon et al., 2021)
The following characteristics are added or modified in the description of Rahnella contaminans given by Jeon et al. (2021). All these features were obtained under toxic growth conditions according to Brady et al. (2014, 2022).
Cells were rods (0.4–0.6 × 2–3.5 μm), single or in pairs, motile by peritrichous flagella and fimbria. Colonies are white, round, smooth, convex with entire border, bright, and do not produce diffusible pigment after 48 h of growth at 28°C in TSA medium. Cultures grow well between 20 and 28°C and pH range from 4 to 10; optimal growth at 28°C and pH 7. Positive for β-galactosidase, tryptophan deaminase, and indole and acetoin production. Negative for arginine dihydrolase, lysine decarboxylase, ornithine decarboxylase, citrate utilization, H2S, and urease. Nitrate is reduced to nitrite. Acid is produced from L-arabinose, D-ribose, D-xylose, D-galactose, D-glucose, D-fructose, D-mannose, L-rhamnose, dulcitol, D-mannitol, N-acetylglucosamine, arbutin, esculin, ferric citrate, salicin, D-cellobiose, D-maltose, D-lactose, D-melibiose, D-saccharose, D-trehalose, D-raffinose, gentiobiose, and D-fucose (API 50CHB/E). Dextrin, glycogen, tweens 40 and 80, N-acetyl-D-glucosamine, L-arabinose, D-arabitol, D-cellobiose, D-fructose, L-fucose, D-galactose, gentiobiose, α-D-glucose, α-D-lactose, maltose, D-mannitol, D-mannose, D-melibiose, D-raffinose, L-rhamnose, D-sorbitol, D-trehalose, sucrose, turanose, pyruvic acid methyl ester, acetic acid, citric acid, formic acid, D-galactonic acid lactone, D-galacturonic acid, D-gluconic acid, malonic acid, quinic acid, D-saccharic acid, glucuronamide, L-alaninamide, D-alanine, L-alanine, L-alanylglycine, L-asparagine, L-aspartic acid, L-glutamic acid, glycyl-L-aspartic acid, glycyl-L-glutamic acid, D-serine, L-serine, inosine, uridine, thymidine, glycerol, D, L-α-glycerol phosphate, α-D-glucose-1-phosphate, and α-D-glucose-6-phosphate (Biolog) are oxidized. Reactions for cis-aconitic acid and bromosuccinic acid are negative. The main fatty acids include C12:0, C14:0, C16:0, C17:0 cyclo, iso-C16:1 and/or C14:0 3-OH (summed feature 2) and C16:1 ω7c and/or C16:1ω6c (summed feature 3). The G + C content of the ecotype was 52.8 mol%. The ecotype of R. contaminans showed the presence of flag-1 primary system encoding peritrichous flagella, as well as fimbriae genes from the families type 1, α, β, and σ, mainly encoding chaperone/usher fimbriae. The ecotype of R. contaminans was recurrently isolated from the gut of Dendroctonus-bark beetles, where it is persistent across the different development stages of these insects and geographical localities.
Data availability statement
The datasets presented in this study can be found in online repositories. The names of the repository/repositories and accession number(s) can be found in the article/Supplementary material.
Author contributions
GZ and FR-O conceived the research. BV-C performed the experiment. XZ and WC performed the chemotaxonomic analysis. FR-O, RP-M, ML, CC-R, and GZ performed the in silico analysis, interpreted the result, and performed the draft and final edition of the manuscript. All authors contributed and approved the final manuscript.
Funding
This research was partially funded by Consejo Nacional de Ciencia y Tecnología (CONACyT-Ciencia de la Frontera 1311330) and Secretaría de Investigación y Posgrado del IPN (20221751). This study was part of the BV-C MsC thesis. BV-C was fellow CONACYT (576556) and the Member of the Program of Beca de Estímulo Institutional de Formación de Investigadores del Instituto Politécnico Nacional (BEIFI-IPN).
Acknowledgments
We would like to thank En Tao Wang Hu (Instituto Politécnico Nacional) and Carrie Brady (University of West England) for their comments.
Conflict of interest
The authors declare that the research was conducted in the absence of any commercial or financial relationships that could be construed as a potential conflict of interest.
Publisher’s note
All claims expressed in this article are solely those of the authors and do not necessarily represent those of their affiliated organizations, or those of the publisher, the editors and the reviewers. Any product that may be evaluated in this article, or claim that may be made by its manufacturer, is not guaranteed or endorsed by the publisher.
Supplementary material
The Supplementary material for this article can be found online at: https://www.frontiersin.org/articles/10.3389/fmicb.2023.1171164/full#supplementary-material
Footnotes
1. ^https://lpsn.dsmz.de/genus/rahnella
3. ^http://atgc.lirmm.fr/phyml/
4. ^https://blast.ncbi.nlm.nih.gov/Blast.cgi
5. ^http://www.atgc-montpellier.fr/phyml/
7. ^https://narrative.kbase.us
8. ^http://exon.gatech.edu/GeneMark/gmhmm2_prok.cgi
9. ^http://www.genome.jp/kegg/
10. ^http://enve-omics.ce.gatech.edu/ani/index
11. ^http://ggdc.dsmz.de/ggdc.php
References
Akbari, S., Oshaghi, M. A., Hashemi-Aghdam, S. S., Hajikhani, S., Oshaghi, G., and Shirazi, M. H. (2014). Aerobic bacterial community of American cockroach Periplaneta americana, a step toward finding suitable paratransgenesis candidates. J. Arthropod. Borne Dis. 9, 35–48.
Alballaa, S. R., Qadri, S. M., Al-Furayh, O., and Al-Qatary, K. (1992). Urinary tract infection due to Rahnella aquatilis in a renal transplant patient. J. Clin. Microbiol. 30, 2948–2950. doi: 10.1128/jcm.30.11.2948-2950.1992
Bolger, A. M., Lohse, M., and Usadel, B. (2014). Trimmomatic: a flexible trimmer for Illumina sequence data. Bioinformatics 30, 2114–2120. doi: 10.1093/bioinformatics/btu170
Boone, C. K., Keefover-Ring, K., Mapes, A. C., Adams, A. S., Bohlmann, J., and Raffa, H. F. (2013). Bacteria associated with a tree-killing insect reduce concentrations of plant defense compounds. J. Chem. Ecol. 39, 1003–1006. doi: 10.1007/s10886-013-0313-0
Brady, C., Asselin, J. A., Beer, S., Brurberg, M. B., Crampton, B., Venter, S., et al. (2022). Rahnella perminowiae sp. nov., Rahnella bonaserana sp. nov., Rahnella rivi sp. nov. and Rahnella ecdela sp. nov., isolated from diverse environmental sources, and emended description of the genus Rahnella. Int. J. Syst. Evol. Microbiol. 72:005190. doi: 10.1099/ijsem.0.005190
Brady, C., Cleenwerck, I., Venter, S. N., Vancanneyt, M., Swings, J., and Coutinho, T. (2008). Phylogeny and identification of Pantoea species associated with plants, humans and the natural environment based on multilocus sequence analysis (MLSA). Syst. Appl. Microbiol. 31, 447–460. doi: 10.1016/j.syapm.2008.09.004
Brady, C., Hunter, G., Kirk, S., Arnold, D., and Denman, S. (2014). Rahnella victoriana sp. nov., Rahnella bruchi sp. nov., Rahnella woolbedingensis sp. Nov., classification of Rahnella genomospecies 2 and 3 as Rahnella variigena sp. Nov. and Rahnella inusitata sp. Nov., respectively and emended description of the genus Rahnella. Syst. Appl. Microbiol. 37, 545–552. doi: 10.1016/j.syapm.2014.09.001
Briones-Roblero, C. I., Hernández-García, J. A., González-Escobedo, R., Soto-Robles, L. V., Rivera-Orduña, F. N., and Zúñiga, G. (2017a). Structure and dynamics of the gut bacterial microbiota of the bark beetle, Dendroctonus rhizophagus (Curculionidae: Scolytinae) across their life stages. PLoS One 12:e0175470. doi: 10.1371/journal.pone.0175470
Briones-Roblero, C. I., Rodríguez-Díaz, R., Santiago-Cruz, J. A., Zúñiga, G., and Rivera-Orduña, F. N. (2017b). Degradation capacities of bacteria and yeasts isolated from the gut of Dendroctonus rhizophagus (Curculionidae: Scolytinae). Folia Microbiol. 62, 1–9. doi: 10.1007/s12223-016-0469-4
Campanella, J., Bitincka, L., and Smalley, J. (2003). MatGAT: an application that generates similarity/identity matrices using protein or DNA sequences. BMC Bioinformatics 4:29. doi: 10.1186/1471-2105-4-29
Chakraborty, A., Ashraf, M. Z., Modlinger, R., Synek, J., Schlyter, F., and Roy, A. (2020). Unravelling the gut bacteriome of Ips (Coleoptera: Curculionidae: Scolytinae): identifying core bacterial assemblage and their ecological relevance. Sci. Rep. 10:18572. doi: 10.1038/s41598-020-75203-5
Charan, S. S., Pawar, K. D., Severson, D. W., Patole, M. S., and Shouche, Y. S. (2013). Comparative analysis of midgut bacterial communities of Aedes aegypti mosquito strains varying in vector competence to dengue virus. Parasitol. Res. 112, 2627–2637. doi: 10.1007/s00436-013-3428-x
Darriba, D., Taboada, G. L., Doallo, R., and Posada, D. (2012). jModelTest 2: more models, new heuristics and parallel computing. Nat. Methods 9, 772–777. doi: 10.1038/nmeth.2109
Durand, A. A., Bergeron, A., Constant, P., Buffet, J. P., Déziel, E., and Guertin, C. (2015). Surveying the endomicrobiome and ectomicrobiome of bark beetles: the case of Dendroctonus simplex. Sci. Rep. 5:17190. doi: 10.1038/srep17190
Fabryová, A., Kostovčík, M., Díez-Méndez, A., Jiménez-Gómez, A., Celador-Lera, L., Saati-Santamaría, Z., et al. (2018). On the bright side of a forest pest-the metabolic potential of bark beetles’ bacterial associates. Sci. Total Environ. 619-620, 9–17. doi: 10.1016/j.scitotenv.2017.11.074
Gonzalez-Escobedo, R., Briones-Roblero, C. I., Pineda-Mendoza, R. M., Rivera-Orduña, F. N., and Zúñiga, G. (2018). Bacteriome from Pinus arizonica and P. durangensis: diversity, comparison of assemblages, and overlapping degree with the gut bacterial community of a bark beetle that kills pines. Front. Microbiol. 9:77. doi: 10.3389/fmicb.2018.00077
Gouy, M., Guindon, S., and Gascuel, O. (2010). SeaView version 4: a multiplatform graphical user interface for sequence alignment and phylogenetic tree building. Mol. Biol. Evol. 27, 221–224. doi: 10.1093/molbev/msp259
Hernández-García, A., Briones-Roblero, I., Rivera-Orduña, F., and Zúñiga, G. (2017). Revealing the gut bacteriome of Dendroctonus bark beetles (Curculionidae: Scolytinae): diversity, core members and co-evolutionary patterns. Sci. Rep. 7:13864. doi: 10.1038/s41598-017-14031-6
Hoffman, C. S., and Winston, F. (1987). A ten-minute DNA preparation from yeast efficiently releases autonomous plasmids for transformation of Escherichia coli. Gene 57, 267–272. doi: 10.1016/0378-1119(87)90131-4
Izard, D., Gavini, F., Trinel, P. A., and Leclerc, H. (1979). Rahnella aquatilis, nouveau member de la famille des Enterobacteriaceae. Ann. Microbiol. 130, 163–177.
Izumi, H., Anderson, I. C., Alexander, I. J., Killham, K., and Moore, E. R. (2006). Endobacteria in some ectomycorrhiza of scots pine (Pinus sylvestris). FEMS Microbiol. Ecol. 56, 34–43. doi: 10.1111/j.1574-6941.2005.00048.x
Jeon, D., Kim, I. S., and Lee, S. D. (2021). Rahnella laticis sp. nov. and Rahnella contaminans sp. nov., and emended description of the genus Rahnella. Int. J. Syst. Evol. Microbiol. 71:004893. doi: 10.1099/ijsem.0.004893
Kačániová, M., Terentjeva, M., Žiarovská, J., and Kowalczewski, P. Ł. (2020). In vitro antagonistic effect of gut bacteriota isolated from indigenous honeybees and essential oils against Paenibacillus larvae. Int. J. Mol. Sci. 21:6736. doi: 10.3390/ijms21186736
Kämpfer, P. (2005). “Genus: Rahnella, the proteobacteria, part B, the gammaproteobacteria” in Bergey’s manual of systematic bacteriology. eds. D. J. Brenner, N. R. Krieg, and J. T. Staley (New York, NY: Springer), 759–763.
Kim, K. Y., Jordan, D., and Krishnan, H. B. (1997). Rahnella aquatilis, a bacterium isolated from soybean rhizosphere, can solubilize hydroxyapatite. FEMS Microbiol. Lett. 153, 273–277. doi: 10.1111/j.1574-6941.2005.00048.x
Kumar, A., Le Flèche-Matéos, A., Kumar, R., Lomprez, F., Fichenick, F., Singh, D., et al. (2022). Rahnella sikkimica sp. nov., a novel cold-tolerant bacterium isolated from the glacier of Sikkim Himalaya with plant growth-promoting properties. Extremophiles 26:35. doi: 10.1007/s00792-022-01283-y
Larkin, M. A., Blackshields, G., Brown, N. P., Chenna, R., McGettigan, P. A., McWilliam, H., et al. (2007). Clustal W and Clustal X version 2.0. Bioinformatics 23, 2947–2948. doi: 10.1093/bioinformatics/btm404
Lee, S. D., Jeon, D., Kim, I. S., Choe, H., and Kim, J. S. (2020). Rahnella aceris sp. nov., isolated from sap drawn from Acer pictum. Arch. Microbiol. 202, 2411–2417. doi: 10.1007/s00203-020-01961-5
Lefort, V., Desper, R., and Gascuel, O. (2015). Fast ME 2.0: a comprehensive, accurate, and fast distance-based phylogeny inference program. Mol. Biol. Evol. 32, 2798–2800. doi: 10.1093/molbev/msv150
Mason, C. J., Hanshew, A. S., and Raffa, K. F. (2015). Contributions by host trees and insect activity to bacterial communities in Dendroctonus valens (Coleoptera: Curculionidae) galleries, and their high overlap with other microbial assemblages of bark beetles. Environ. Entomol. 45, 348–356. doi: 10.1093/ee/nvv184
Meier-Kolthoff, J. P., Auch, A. F., Klenk, H. P., and Göker, M. (2013). Genome sequence-based species delimitation with confidence intervals and improved distance functions. BMC Bioinformatics 14:60. doi: 10.1186/1471-2105-14-60
Meier-Kolthoff, J. P., and Göker, M. (2019). TYGS is an automated high-throughput platform for state-of-the-art genome-based taxonomy. Nat. Commun. 10, 2182–2110. doi: 10.1038/s41467-019-10210-3
Morales-Jiménez, J., Vera-Ponce de León, A., García-Domínguez, A., Martínez-Romero, E., Zúñiga, G., and Hernández-Rodríguez, C. (2013). Nitrogen-fixing and uricolytic bacteria associated with the gut of Dendroctonus rhizophagus and Dendroctonus valens (Curculionidae: Scolytinae). Microb. Ecol. 66, 200–210. doi: 10.1007/s00248-013-0206-3
Mukhia, S., Kumar, A., Kumari, P., and Kumar, R. (2022). Psychrotrophic plant beneficial bacteria from the glacial ecosystem of Sikkim Himalaya: genomic evidence for the cold adaptation and plant growth promotion. Microbiol. Res. 260:127049. doi: 10.1016/j.micres.2022.127049
Parker, C. T., Tindall, B. J., and Garrity, G. M. (2019). International code of nomenclature of prokaryotes. Int. J. Syst. Evol. Microbiol. 69, S1–S111. doi: 10.1099/ijsem.0.000778
Pineda-Mendoza, R. M., Zúñiga, G., López, M. F., Hidalgo-Lara, M. E., Santiago-Hernández, A., López-López, A., et al. (2022). Rahnella sp., a dominant symbiont of the core gut bacteriome of Dendroctonus species, has metabolic capacity to degrade xylan by bifunctional xylanase-ferulic acid esterase. Front. Microbiol. 13:911269. doi: 10.3389/fmicb.2022.911269
Rambaut, A. (2012). FigTree v1.4. Molecular evolution, phylogenetics and epidemiology. Edinburgh. Available at: http://tree.bio.ed.ac.uk/software/figtree.
Sasser, M. (1990). Identification of bacteria by gas chromatography of cellular fatty acids. MIDI technical MIDI note #101. MIDI Inc., Newark, DE.
Seemann, T. (2014). Prokka: rapid prokaryotic genome annotation. Bioinformatics 30, 2068–2069. doi: 10.1093/bioinformatics/btu153
Six, D. L., and Bracewell, R. (2015). “Dendroctonus” in Bark beetles: biology and ecology of native and invasive species. eds. F. E. Vega and R. W. Hofstetter (San Diego, CA: Academic Press Elsevier), 305–350.
Thanassi, D. G., Nuccio, S. P., Shu Kin, S. S., and Bäumler, A. J. (2007). Fimbriae: classification and biochemistry. EcoSal Plus 2, 1–27. doi: 10.1128/ecosalplus.2.4.2.1
Xu, L., Lou, Q., Cheng, C., Lu, M., and Sun, J. (2015). Gut-associated bacteria of Dendroctonus valens and their involvement in verbenone production. Microb. Ecol. 70, 1012–1023. doi: 10.1007/s00248-015-0625-4
Xu, L. T., Lu, M., and Sun, J. H. (2016). Invasive bark beetle-associated microbes degrade a host defensive monoterpene. Insect Sci. 23, 183–190. doi: 10.1111/1744-7917.12255
Keywords: Enterobacteriaceae, gut symbiont, bark beetles, Dendroctonus, gut bacteriome
Citation: Rivera-Orduña FN, Pineda-Mendoza RM, Vega-Correa B, López MF, Cano-Ramírez C, Zhang XX, Chen WF and Zúñiga G (2023) A polyphasic taxonomy analysis reveals the presence of an ecotype of Rahnella contaminans associated with the gut of Dendroctonus-bark beetles. Front. Microbiol. 14:1171164. doi: 10.3389/fmicb.2023.1171164
Edited by:
Amparo Latorre, University of Valencia, SpainReviewed by:
Toni A. Chapman, NSW Government, AustraliaImen Nouioui, German Collection of Microorganisms and Cell Cultures GmbH (DSMZ), Germany
Copyright © 2023 Rivera-Orduña, Pineda-Mendoza, Vega-Correa, López, Cano-Ramírez, Zhang, Chen and Zúñiga. This is an open-access article distributed under the terms of the Creative Commons Attribution License (CC BY). The use, distribution or reproduction in other forums is permitted, provided the original author(s) and the copyright owner(s) are credited and that the original publication in this journal is cited, in accordance with accepted academic practice. No use, distribution or reproduction is permitted which does not comply with these terms.
*Correspondence: Gerardo Zúñiga, capotezu@hotmail.com