- 1Department of General and Specialized Medicine, Sleep Disorders Center, University Hospital of Parma, Parma, Italy
- 2Szentagothai János School of Ph.D Studies, Clinical Neurosciences, Semmelweis University, Budapest, Hungary
- 3Department of Behavioral Sciences, National Institute of Clinical Neurosciences, Semmelweis University, Budapest, Hungary
- 4Division of Pulmonary, Critical Care and Sleep, Department of Medicine, Beth Israel Deaconess Medical Center and Harvard Medical School, Boston, MA, United States
- 5Department of Medicine and Surgery, Unit of Neurology, University of Parma, Parma, Italy
Sleep medicine is an ambitious cross-disciplinary challenge, requiring the mutual integration between complementary specialists in order to build a solid framework. Although knowledge in the sleep field is growing impressively thanks to technical and brain imaging support and through detailed clinic-epidemiologic observations, several topics are still dominated by outdated paradigms. In this review we explore the main novelties and gaps in the field of sleep medicine, assess the commonest sleep disturbances, provide advices for routine clinical practice and offer alternative insights and perspectives on the future of sleep research.
The evolution of sleep medicine is simultaneously uneven, turbulent, exuberant, exciting, disappointing, confusing, and exhilarating. There is a continuous push and pull between the several forces, which typically sculpt a field, especially a relatively new one. For instance, the hurry of science leads the evolution of formal treatment guidelines. This is amplified by the fact that the boundaries of sleep medicine and science are nearly infinite, with inputs from a range of specialties and the confluence of views of individuals and entities with very divergent visions. This article attempts to capture the excitement of clinical and translational sleep medicine, explore the existing gaps and needs, integrate cutting edge technologies, and propose some solutions.
Insomnia and the disturbed, unhappy sleeping brain
In 2005, the inaugural issue of the Journal of Clinical Sleep Medicine reviewed the history of sleep research in the United States, where Sleep Medicine had recently been recognized as a specialty of medicine (1). The article chronicled the development of sleep science and sleep organizations over the previous 70 years, highlighting the progressive increase of knowledge concerning the physiology of sleep, circadian biology and the pathophysiology of sleep disorders. In the closing session, entitled The Future of Sleep Medicine, the authors pointed out the major challenges linked to the technological advances of our society: The development of artificial lighting has contributed greatly to the problem of sleep deprivation. We are now able to run factories, stores and the Internet 24/7; thereby, increasing the efficiency of economic activity. However, we have not been able to adapt human circadian rhythms and need for sleep to meet either the economic demands of society or the socially desired preferences of individuals that result in insufficient sleep. “On demand” sleep and/or alertness will likely become a major goal and challenge for the field of Sleep Medicine. Pharmacological treatments to promote sleep predisposition or, conversely, to stimulate wakefulness, are available but have limited efficacy. Adjusting the timing of light/dark exposure effectively shifts circadian rhythms but it is slow, difficult to regulate and relies on patients' compliance. New methods, technologies and treatments must be developed to meet the demands for alertness and sleep.
Fifteen years after the acceptance of sleep medicine as a distinct speciality, the interplay between sleep and society remains an open and unsolved dilemma. In particular, insomnia remains a common disorder in the general population, with variable prevalence-estimates in the different age groups and in relation to socio-demographic, clinical, and psychosocial parameters. A systematic review published in 2019 (2) established that: (a) approximately one third of adults (>18 years of age) reported dissatisfaction with their sleep and at least one symptom of insomnia. It has also been shown, that: (a) the use of prescription sleep aids, particularly non-benzodiazepines and (off-label) antidepressants, has risen significantly over the last 20 years; (b) 70% of patients using a prescription sleep aid continue to do so at 1-year follow-up despite lack of significant improvements in sleep compared to non-users; (c) up to 60% of sleep aids used by adults for insomnia, are over-the counter products (3–5). According to the European guideline for the diagnosis and treatment of insomnia (6), there is very little information in terms of the management and persistence of chronic insomnia. Moreover, the prevalence of insomnia and of hypnotic usage (benzodiazepines and benzodiazepine receptor agonists) varies largely from one European country to the other. Several national surveys in general practice (GP) or medical specialty settings have been conducted. In Italy (7), insomnia was reported by 64% of all interviewed patients; more than 50% of GP patients had insomnia in Norway (8) and in Germany (9) where the prevalence of having taken a hypnotic drug at least once, increased from 4.7 to 9.2% from 2009 to 2016 (10). A recent analysis on the assessment and management of insomnia illustrates numerous gaps in research carried out to date (11): (a) inadequate information on the specific effects of various components of Cognitive Behavioral Therapy (CBT-I) which might allow greater treatment efficiency and tailoring; (b) lack of double-blind, placebo-controlled, randomized trials demonstrating the efficacy of pharmacological treatments in children or adolescents with insomnia; (c) lack of rigorous investigations on a number of agents commonly used to treat insomnia in clinical practice, including trazodone, quetiapine and gabapentin; (d) lack of pharmacological treatment of insomnia in the setting of fragile medical conditions such as dementia, mild cognitive impairment and substance use disorders; (e) necessity to move to greater personalization in clinical practice and therapy. These considerations, combined with the extension of the daytime due to electric lighting exposure, internet connection, digital revolution, shift work, geographical and social jet-lag; suggest that, at least in the field of insomnia, the future of sleep medicine is still unclear. With the arrival of SARS-Cov 2, the picture has become even more complicated.
Insomnia in the time of COVID
The COVID-19 pandemia has enhanced the difficulty to find a set-point between the laws of physiology and the rules of modern life. The first lockdown triggered worldwide a sharp increase in sleeping problems. In a survey conducted in Italy and Belgium (12), sleep timing was significantly delayed, time spent in bed increased, and sleep quality was markedly impaired. The most vulnerable individuals appeared to be women, subjects experiencing more negative mood and those perceiving the pandemic situation as highly stressful. Sleep quality and timing underwent significant modifications especially in unemployed participants. In contrast, positive mood showed a protective effect against the risk of experiencing poor sleep quality. In a French general public sample, COVID-19-related worries and loneliness were major contributing factors to clinical insomnia (13). During the shutdown in China, a survey revealed that insomnia was highly prevalent and associated with COVID-19 outbreak–related psychological reactions and poor sleep hygiene (14). These findings indicate that both excessive social mobility (pre-COVID) and prolonged social isolation (pandemic lockdown) can jeopardize sleep quality, introducing additional caveats in the competition between Nature and Culture. According to Immanuel Kant: There is nothing more unsociable than Man, and nothing more sociable: unsociable by his vice, sociable by his nature. A relevant association between insomnia severity and confinement, loneliness, perceived stress, anxiety and/or depressive symptoms have been confirmed by numerous studies worldwide, highlighting the need for large-scale social intervetion against dramatic socio-health crises.
The semantic shades of insomnia
In the Latin dictionary, the definition of insomnia is not confined to the difficulty of starting and/or maintaining sleep, but also includes the inability to sleep well due to nightmares or agonizing dreams. Dream is said somnium (neuter), but there is also the word insomnia (feminine) to mean sleeplessness. In the Aeneid, Dido reveals to her sister Anna: these dreams stir and frighten me (insomnia terrent). Virgil uses insomnium as a plural noun—insomnia—to signify frightening (terrent) dreams. Accordingly, individuals frequently experiencing nightmares, report compromised sleep quality, poor daytime mood and functioning. The last 5 min of REM (rapid eye movements) sleep before awakening were analyzed individuals experiencing nightmares, and a comparison of REM sleep was made subjects with non-nightmare dream episodes and non-dreaming control individuals (15). Overall, there was no general difference in autonomic activation of nightmare sufferers compared to control individuals. However, when nightmare persones experienced nightmares, the autonomic activation was markedly increased compared to their own non-nightmare dreams and, to some extent, to control's dreams demonstrating an increased autonomic activation associated to nightmares. These findings support the role of vegetative responses in impaired self-reported sleep quality.
Insomnia phenotypes: The sleep train
Insomnia definition has dynamically evolved throughout the years. Insomnia can be either acute or chronic; organic or non-organic; initial, middle and late-night in terms of occurrence; paradoxycal or psychophysiological, associated with misperception or objectively confirmed, isolated or associated with coexistent sleep disorders. Insomnia may affect patients suffering from various neurodegenerative disorders, likely reflecting differential pathogenetic mechanisms and frequently impacting on patients's caregivers as well. Sleep fragmentation is also commonly observed in the acute/subacute phase of neurological disorders such as stroke, traumatic brain injury, encephalitis.
The scenario is far from homogeneous and the subgrouping of insomnia disorder is an ongoing challenge in sleep research. Research investigation should harmonize definitions and categories of the heterogeneous field of insomnia disorder. In the effort of “phenotyping” the insomnia disorder, a practical schematic representation for both clinicians and patients can be portrayed by the “sleep train”. With the sleep histogram, the succession of stages and cycles can be imagined as a train composed of 5 carriages (Figure 1). Each wagon, represented by the complete sleep cycle, lasts about an hour and a half. The first three carriages, which constitute the so-called “core sleep”, are mainly controlled by the gamma-aminobutyric acid (GABA), a sedative neurotransmitter. The last two wagons compose the traditionally defined “optional or complementary sleep” modulated by activating neurotransmitters including acetylcholine, which prepares the brain to morning awakening. The transition point between the two types of sleep, placed between the third and fourth carriage, coincides with a delicate phase of sleep continuity and often represents the middle-of-the-night awakening for many insomniacs. During the first 3–4 h of the night the GABA carriages recharge the brain battery attenuating the tendency to doze-off during the day. Lack of excessive daytime sleepiness (EDS) is often found in insomniacs who sleep soundly for at least 4 h in the first part of the night. However, detachment of the hook between the 3rd and 4th wagon can derail the last two cars of sleep, which travel under the dominion of acetylcholine, fundamental for memory consolidation and muscle activity. Therefore, when a patient complains of sleep maintenance insomnia, it becomes crucial to clarify the time at which nocturnal awakenings occur. If these prevail in the early part of the night, the diurnal repercussions (excessive sleepiness) will be different from what happens when only the last 2–3 h of sleep are compromised (fatigue, difficulties in attention and concentration, poor or absent dream activity). Therapeutic strategies will differ according to the sleep wagons that need stronger protection. For example, GABA-ergic drugs, which sustain sleep continuity when the initial wagons are frail, become unsuitable for late sleep disruption and their administration under the acetylcholine dominance (wagons 4 and 5) represents a neurochemical inconsistency.
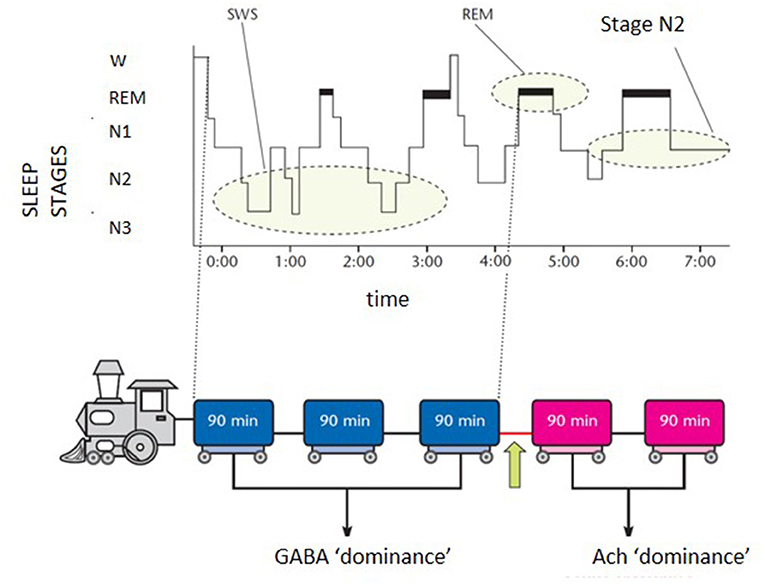
Figure 1. Schematic representation of the “sleep train”. GABA, gamma-aminobutyrate; Ach, acetylcholine.
Insomnia and sleep-disordered breathing
Sleep and breathing are rarely at peace with each other, disordered breathing being a common stressor of the sleep system. While the official guidelines promote the idea that apnea and insomnia are separate, there is mounting evidence that the two can coexist and interact in complex ways. One term surfacing increasingly is “COMISA”, Comorbid Insomnia and Sleep Apnea. This is especially true for central/high loop gain/NREM-dominant sleep apnea, with very prominent unstable NREM (non rapid eye movements) sleep. Low arousal threshold and arousals in general tend to destabilize NREM sleep, contributing to sleep-breathing instability and worsening of sleep apnea. The time is here to carefully and consistently estimate the state of sleep-breathing in the evaluation and management of chronic insomnia.
Insomnia as a chamaleontic condition
Although in many cases chronic insomnia represents the main sleep disorder, there are many other cases where insomnia is only the tip of the iceberg, being the most visible consequence of a more complex sleep disturbance.
Basically, any sleep disorders can lead to clinically significant insomnia: from periodic limb movement disorder to NREM sleep parasomnias or sleep related epilepsies.
A detailed sleep anamnesis, enriched with instrumental evaluation (e.g., cardio-respiratory recording, video-polysomnography, actigraphy.) is essential to avoid misinterpretation.
According to the European guidelines the diagnostic procedure for insomnia should always include a sleep history inclusive of sleep habits, sleep environment, work schedules, circadian factors, the use of validated sleep questionnaires and/or sleep diaries, evaluation of somatic and mental health and a physical examination (6).
Insomnia and CBT-I
Insomnia has frequent medical and mental health comorbididities, including post-traumatic stress disorder, depression and psychosis. Cognitive behavioral therapy for insomnia (CBT-I) is a safe and effective treatment for chronic insomnia in the context of various comorbid conditions. CBT-I is considered the first-line treatment for chronic insomnia in adults. A pharmacological intervention should be considered when CBT-I is not sufficiently effective or not available, mainly based on either short-acting benzodiazepines, benzodiazepine receptor agonists and/or antidepressants. Short term protocols with medications are recommended (ideally ≤ 4 weeks). It is effective in improving sleep and functioning in military settings including active duty-service members to older veterans with complex presentations including post-traumatic stress disorder, depression, sleep apnea, and chronic pain. Data evaluating CBT-I frequently focus on women service-members or individuals with substance abuse. While meta-analyses demonstrate the value of CBT-I, they also note significant heterogeneity. The variability in CBT-I components across trials makes it difficult to determine which aspects are the most responsible for the observed benefits. Future research is needed to better establish the effectiveness of CBT-I in patients with comorbid conditions, as well as on treatment sequencing and alternative methods of CBT-I delivery. In the treatment of insomnia, CBT-I and hypnotic drugs carry advantages and limitations of their own. Medications with specific indications for insomnia produce rapid symptomatic relief, but there is little to no evidence that sleep improvements are maintained after drug discontinuation or during long term use. Conversely, CBT takes longer than drugs to produce sleep improvements, but benefits are well-sustained over time. In the acute phase of CBT-I, adding pharmacotherapy may have a slightly better effect compared with CBT-I alone, if the medication is discontinued in the maintenance phase of CBT-I. Moreover, pharmacotherapy is not indicated for chronic use.
Unfortunately, CBT-I is not available in several countries, even though it carries multifold contradictions.
Several sleep disturbances are caused by wrong habits (busy lifestyles, sedentarism and weight gain, malnutrition and junk food, smoking, alcohol and drug abuse, shift-work, social jet-lag), and the so-called “organic” sleep disturbances, i.e., nocturnal breathing disorders, can co-occur with insomnia related to post-traumatic stress disorder, chronic painful conditions or even cancer. All such combinations are in favor of CBT-I treatment, which aims at reframing misconceptions and dysfunctional thoughts. In many cases, patients with primary insomnia experience subjective improvement with CBT-I: less time to fall asleep, more time spent asleep and waking up less during sleep. Treatment generally takes 6–8 lessons and may be as short as two sessions when given by a primary care doctor. In addition, online resources and smartphone applications offering digital CBT-I are available. Despite these promising opportunities, the question remains: is the therapeutical power of CBT-I really effective against labor constraints, global mobility, internet connection, round-the-clock consumption and production, daily menances against life rhythms and enhancement of waking hours (16). In other words, how effectively can CBT-I stand the challenge of a non-stop sleep-shrinking society? The uneven conflict between dwarfs (CBT-I) and giants (cultural forces) sends a sign of weakness to drug companies seeking for new neurochemical approaches to treat sleeplessness.
If insomnia symptoms can be effectively solved by easy-to-apply and short-lasting techniques of relaxation combined with educational and cognitive interventions, then why huge investments should be dedicated to discover new agents for a sleep disorder which can be managed by a professional psychological approach? Mutatis mutandis, would the managers and stakeholders of pharmaceutical industry dedicate a substantial research and development budget for a new medication against cancer if the latter could be treated by psychological interventions? These questions do not dampen or mortify the positive role of CBT-I, but query the field of sleep experts and international societies who continue to publish guidelines establishing that objective measures, i.e., polysomnography, neuroimaging, biomarkers are useless or unnecessary for the diagnosis and management of insomnia.
Finally, CBT-I present some disadvantages: its efficacy strongly depends on patients' cooperation, it does not work for all types of chronic insomnia disorder, it is time consuming, it is not easily available everywhere and is an expensive approach. Finally, as for any kind of counseling, it strongly depends on therapists' ability.
New wine in old wineskins
Compounds that people take to treat insomnia include dietary supplements and over-the-counter sleep aids (for which prescription is not required), off-label sedating medications and approved medications for insomnia. Agents used for insomnia promote sleep by means of different mechanisms: enhancing GABAergic neurotransmission, antagonizing receptors for the wake-promoting monoamines, or binding the melatonin receptors (17). Orexin receptor antagonists comprise a new class of hypnotic drugs, which promote sleep by decreasing orexin-associated CNS arousal (18). The mechanism of action targets a region of the hypothalalmus involved in the regulation and sleep and wakefulness. An important decrease or total loss of orexin-containing neurons has been reported in brains of patients with narcolepsy. Among orexin-receptor antagonists, Suvorexant is available on the US market. Compared to placebo, 1 month of treatment with Suvorexant improves sleep to a greater extent as assessed by the insomnia severity index (ISI) with dose-dependent effects. In particular, remission is reported in 30% of patients using 20/15 mg (non-elderly/elderly) and in 35.5% of patients using 40/30 mg (non-elderly/elderly) of the active compound. The ratio of improving patiens increase to 48 and 56%, respectively when medication is used for 3 months. Although better than placebo, these findings indicate that Suvorexant warrants a clearcut improvement only in 30–56% of patients with insomnia. Furthermore, there is considerable variability among individuals and there may be a delay following a high-fat meal. Finally, the elimination half-life is ~12 h. After decades of recommendations promoting the use of hypnotic agents with short half-life in order to avoid residual hangover effects, the new wine of pharmacotherapy offers an old wineskin perspective. Hopefully, new anti-orexinergic compounds (daridorexant) with shorter half-lives and limited side-effects will be soon available to explore alternative pathophysiological and neurochemical pathways in the treatment of insomnia.
Another potential approach for chronic insomnia is the use. Data on pediatric patients are still fragmentary, adult insomniac patients seem to benefit from short-term protocols with nabilone and dronabinol, although it seems that long-term therapy might impair sleep quality (19).
Poor sleepers: Orphaned patients
There is a category of orphaned patients who do not belong to a specific category of sleep disorders. They are people who generally complain of sleeping badly and feeling tired and poorly rested during the day. However, if they carry out a PSG recording, the examination shows no significant alterations, at least for the official standards. They are generally adults and their conventional objective measures (sleep efficiency, sleep stages) remain within the age-related ranges. Sometimes, an increased amount of nocturnal awakenings are recorded but we're not talking about classical imsomniacs with difficulty initiating or maintaing sleep. These subjects are all-night sleepers but they wake up poorly refreshed and with the sensation of a restless night. Still, the apena-hypopnea index is < 5/h and the periodic limb movement index remains < 15/h. Both the 3rd International Classification of Sleep Disorders and DSM-V include “non-restorative sleep” in the category of insomnia, but so far an approved marker of sleep quality is lacking. Unfortunately, in several countries, if you are not affected by sleep apnea or PLM you are addressed to a psychologist/psychiatrist and not to a sleep specialist. In some cases, a CBT-I, when available, can improve the symptoms, but once again we are neglecting the core message of the issue: what are the organic bases of poor sleep? In a study conducted on 385,292 British men and women, “sleep scores” were assigned on a scale of zero to five giving one point for having each of five indicators of healthy sleep: being an early bird, sleeping 7–8 h a night, having no insomnia, not snoring and not being sleepy during the day. The scores depended on self-reports of sleep behavior. Over the following 8 years, researchers found that the lower the sleep score, the higher the person's risk for coronary heart disease or stroke. Compared to the poorest sleepers, those who scored 5 had a 34% reduced risk for both coronary heart disease and stroke (20). If subjective poor sleep may increase the risk for cardiovascular disease, then there must be underlying mechanisms promoting a shift toward autonomic activation. A reliable candidate is the arousal system during sleep. Sudden drops in pulse wave amplitude (PWA) measured by pulse oximetry during sleep are commonly associated with simultaneous arousals. When a total of 1,085 PWA drops (below 20%) from 10 consecutive sleep recordings were analyzed, a significant increase in EEG power density in all frequency bands was found during PWA drops (P < 0.001) compared to before and after drop. Even in the absence of conventional EEG arousals, drops in PWA were associated with a significant increase in EEG power density, suggesting that these events can be used as surrogate markers for changes in cortical activity during sleep (21). As a confirmation, analyzing the PSG recordings of 20 male individuals with obstructive sleep apnea (OSA) the pulse wave amplitude drops (below 30%) during respiratory events were quantified and the combined or separate occurrence with the A phases of CAP (cyclic alternating pattern) was measured (22). A dual response (A-phase associated with a pulse wave amplitude drop) was the most frequent finding (71.8% in total sleep time) for all types of respiratory events, with a progressive reduction from apneas to hypopneas and flow limitation events. The highly significant correlation between CAP A-phases and relevant pulse wave amplitude drops (p < 0.0001) suggests a possible role of autonomic arousals as a marker of cerebral response to respiratory events and confirms the significance of PWA drops as indirect electrophysiological biomarkers of sleep instability. Thus, it would be interesting to explore the advantage of PWA (and CAP) in the classification of insomnia phenotypes.
CAP represents a pivotal component of the dynamic sleep structure and can be considered a polysomnographic hallmark of sleep instability. CAP is organized in cycles, with subsequent cycles composing a CAP sequence. Depending on the presence or absence of CAP cycles, sleep itself can be divided into “CAP sleep” and “non-CAP sleep”. Each CAP cycle can be subdivided in a phase A (activation phase) and a following phase B (de-activation phase). Phase A can be further classified in three subtypes: A1, A2 and A3, according to reciprocal proportion of slow waves and fast rhythms (see Figure 2). The distinction is not trivial as CAP subtypes A1 typically boost SWS and reinforce sleep propensity, whilst CAP subtypes A2 and A3 usually translate a tendency toward REM sleep and/or prepare the brain for an awakening. The utilization of CAP metrics ensures a deeper understanding of sleep kinetic troughtout the night, however, as CAP analysis is highly demanding and time-consuming, not all the sleep laboratories can guarantee its measurement in everyday clinical practice.
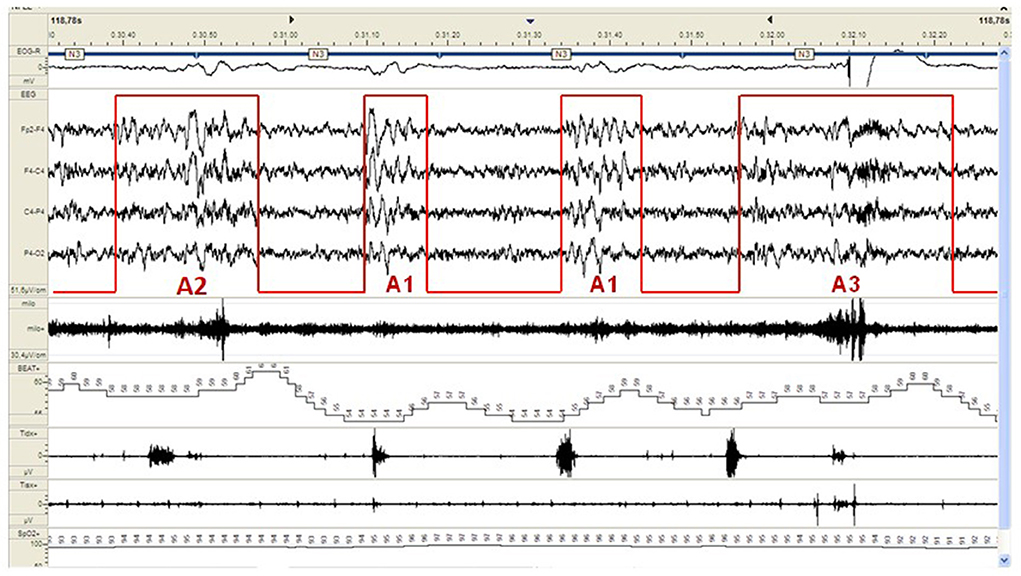
Figure 2. Example of a CAP sequence (CAP phases A highlighted in red) during stage N3 of NREM sleep in a patient affected by periodic limb movement disorder (PLMD). Note that the more disturbing leg movements (right part of the figure) are associated with CAP subtype A3. CAP subtypes A1 have a milder cardiovascular impact.
Novel methods to measure the level of NREM sleep instability, including cardiopulmonary coupling (CPC) spectrogram, have been developed and proved useful in tracking the effect of treatment (23). According to a recently published review of the available portable systems (2021), the EEG-based systems are the most accurate, while the photoplethysmography-based systems are simpler and better suited for wearable monitoring. Perhaps, time is ripe to entrust poor sleepers to a clinical family including CAP metrics and vegetative monitoring in the conventional sleep scoring procedures.
Literature is still lacking of clear “endoptype models” for chronic insomnia disorder, as pathophysiological mechanisms related to this condition are currently largely unknown and, so far, diagnosis is based on clinical evaluation. In this perspective, we suggest that the utilization of CAP metrics might help to reveal endotypes and subendotypes of this condition, while, concurrently, it might help to categorize various insomnia phenotypes.
Restless leg syndrome and periodic limb movement disorder
Sleep, at any age, can be perturbed by numerous sleep-related movement disorders: a group of conditions characterized by simple, sometimes stereotyped, movements that can hamper sleep continuity and quality. The category of sleep-related movement disorders of the International Classification of Sleep Disorders (24) includes restless leg syndrome (RLS), periodic limb movement disorder (PLMD), propriospinal myoclonus at sleep onset, sleep-related leg cramps, bruxism, sleep-related rhythmic movement disorder, benign sleep myoclonus of infancy and the so-called “isolated symptoms and normal variants” comprehensive of excessive fragmentary myoclonus, hypnagogic foot tremor, alternating leg muscle activation and sleep starts. More recently a novel independent pediatric sleep-related movement disorder named restless sleep disorder (RSD) has been categorized (25).
PLMD and RLS diagnostic criteria
RLS and PLMD are the commonest, frequently overlapping, sleep-related movement disorders in adulthood, with a prevalence estimated 5–15% and 4–11%, respectively (26, 27).
PLMs are repetitive, stereotyped, non-epileptiform, involuntary movements during sleep, usually involving the lower limbs and ending with a spinal cord flexor reflex-like motor pattern (28). The movements last 0.5–10 s, repeating at 5–90 s intervals. At least 4 consecutive movements define a PLM sequence (24). The PLM index is the total number of PLMs/total sleep time, and the cut-off values are fixed at >5/h in children and >15/h in adults. The definition of PLMD requires the instrumental motor findings associated with clinical consequences (insomnia and/or excessive daytime sleepiness) (29). In 2016, a task force of the Word Association of Sleep Medicine (WASM) revised the PLMD criteria to prevent “over-diagnosis” and promote the development of reliable automatic scoring (30). According to the WASM criteria either shorter (< 10 s) or longer (>90 s) intermovement intervals interrupt a series of PLMs (“periodicity criteria”), while the morphology of Leg Movements (LMs) needs to be evaluated to discard irrelevant or irregular movements; and in conditions involving predominantly one side e.g., hemiparesis, a separate unilateral analysis is recommended (30). The current rules for PLMs scoring suggest to exclude those movements from LM count linked (within 0.5 s) to a phasic respiratory event (29). Application of these criteria markedly reduces the frequency of PLMD diagnosis, confirming the risk for overestimation of the condition applying traditional scoring rules (31). An alternate view is that ALL movements should be accurately identified and tabulated to estimate the burden of motor activation. Clearly there are “kickers and non-kickers” for any given degree of sleep apnea, and we should better study these associations and the impact of concomitant neural subsystem activation, on physiological and clinical outcomes.
RLS diagnosis is based on four core clinical criteria (instrumental confirmation is not required): (1) an urge to move limbs with a typical unpleasant sensation; (2) symptoms worsening with inactivity; and (3) partially or totally relieved by movement; (4) worsening in the evening/night (32). Common mimics include restlessness of other sorts (psychiatric disorders, drugs) and sensory discomfort due to peripheral neuropathy, myelopathy or vascular diseases (33).
Circadian/ultradian distribution of PLM and RLS
PLMs are largely prevalent during stage N1 and N2 of NREM sleep (34), progressively declining in deeper sleep and, even more, in REM sleep. The majority of LMs occur during the first sleep cycles and progressively declines overnight. This overnight/ultradian pattern might correlate with the circadian rhythm of core body temperature, melatonin dynamics, hormonal changes and dopamine activity (34–36). Similar circadian variations in RLS severity have been observed, significantly correlating with subjective vigilance level, core body temperature and salivary melatonin (37). Based on these findings, an inhibitory effect of melatonin toward dopamine secretion has been hypothezed. Intringuingly, the predictable circadian distribution of PLMs progressively disappears with aging; after the age of 75 years, the involuntary movements typically persist throughout the entire night, losing their ultradian pattern (38).
Age-dependent dynamics of PLM and RLS
Recent data demonstrate a para-physiological age-related increase of PLM index across lifespan: its value starts to grow after age 10 years (mean value 4.5 ± 4.46/h), rises up to 25.7 ± 10.9/h at age 40 years, reaches 35.6 ± 23.3/h at 60 with a final increase over the age of 80 years (39).
However, when additional features are taken into account - such as the Periodicity Index (PI) - lower values of PLM index emerge. This point suggests a distinct (maybe not pathological) significance of these “non-periodic” movements during sleep, that are probably influenced by numerous external of internal factors perturbing sleep (e.g., coexistent sleep disturbances, changes in sleep stages that take place with aging), while the more “periodic” movements might reflect, in the youngest, the maturation of brain connections in parallel with daytime experiences (40, 41). Hence, to ensure a reliable clinical evaluation, PLM index should always be related to patient's age and additional features such as PI may ease the recognition of the more disturbing LMs.
The lifetime course of PLM index (PLMI) and PI does not overlap, as PLMI progressively increases with aging; PI reaches a plateau at around 15–25 years of age and remains somewhat stable up to 65 years. These “ontogenetic” differences suggest that the two phenomena mirror distinct neurobiological mechanisms (38).
Conversely, there is an impressive overlap between the typical PLMI curve and the nocturnal distribution of sleep instability, which can be measured using the CAP metrics (42). PLM sequences trigger powerful arousal intrusions during sleep, represented by CAP subtypes A2 and A3, and, at the same time, CAP cycles associated with PLMs last usually longer (42), suggesting a reciprocal influence between CAP dynamics and PLM.
Interestingly, in some cases, pharmacological interventions can markedly reduce LMs with no effect on sleep instability (e.g., dopaminergic drugs) and, vice-versa, some other drugs (e.g., clonazepam) can ameliorate CAP rate with no variations of PLMI (43).
RLS can either coexist with PLM or occur independently, especially in older people. RLS may affect children, adults and the elderly. Various lifetime changes (including pregnancy and breastfeeding) may enhance or provoke RLS symptoms' exacerbation. Both early-onset (frequently related to genetic predisposition) or late-onset variants exist (44). RLS diagnosis in the elderly may be particularly challenging due to the coexistence of cognitive disorders, making it hard to describe symptoms, and/or conditions acting as RLS mimics such as drug-induced akatisia, myelopathy, myopathy, neuropathy or vascular claudicatio (45). Patients with comorbidities and polytherapies represent the most complex scenario, as many drugs can exacerbate RLS severity (e.g., antihistamines, selective serotonin reuptake inhibitors). Moreover, reduced iron adsorption and reduced mobility/sarcopenia may worsen pain/sensory disturbance (44).
RLS, PLM and sleep fragmentation
According to current diagnostic criteria, PLMS are considered to be associated with cortical arousals (the two events are separated by < 0.5 s), regardless of which appears first (29). Around 17–55% of PLMs are associated with arousals, with longer LMs associated with a higher risk and being more disturbing than shorter ones (46). PLMs may also trigger abnormal arousal reactions evoking NREM sleep parasomnia episodes (47) and/or sleep-related epileptic seizures (48).
Sleep macrostructure in PLMD is characterized by a relevant reduction of slow wave sleep (SWS) with parallel increase of lighter sleep stages (49). A pathological increase of microarousals is also typical of this condition, with a rise of CAP subtypes A2 and A3, the more “disturbing” CAP subtypes, that in many cases (around 95%) are strongly synchronized with limb movements (50). CAP has a well-known double significance: exerting a protective and “sleep promoting” function on the descending slopes of the first sleep cycles and a “wake/REM promoting” effect on the ascending slopes of them. The “buffer system” aspect is a main feature of the dynamics of A1 events whilst A2-A3 events are related to true “sleep fragmentation”, the commonest sleep microstructural fluctuation in symptomatic PLMD.
The relationship between PLM and sleep microstructure is not always predictable and, in some cases, the sleeping brain activity can keep its periodic oscillatory pattern, while limb jerks might disappear with medications (dopaminergic drugs) or, vice-versa, LMs can persist with minor impact on brain activity (clonazepam), suggesting a form of complementarity between these medications. Thereafter, the decision to focus on the limb motor phenomena rather than ensuring a more stable sleep continuity, is always a puzzling challenge for the clinician. The combination of both clonazepam and dopaminergic medication, if well-tolerated by patients, is probably the most adequate choice to guarantee a more comprehensive approach to this “multidimensional/multilevel” condition.
So far, the relationship between isolated RLS and sleep fragmentation is still blurry, as most studies analyze sleep texture in patients with coexistent RLS/PLMD. However, RLS alone can provoke chronic sleep onset insomnia and (subsequent) sleep deprivation: the two conditions potentially associated with metabolic impairment (reduction in insulin sensitivity, glucose intolerance), vascular dysfunction (impaired endothelial function and vascular stiffness) and increased sympathetic activity (51). Patients with moderate-to-severe RLS present higher intensity of sympathetic activation following LMs compared to mild RLS, showing around 6-times more episodes per night of movement-associated transient heart rate increase (52).
As the majority of polysomnographic studies so far enrolled patients with overlapping RLS/PLMD, the impact of isolated RLS on sleep microstructure remains to be studied.
The management of RLS focuses on non-pharmacological interventions (sleep hygiene; avoidance of caffeine stimulating beverages, smoking, alcohol and excessive physical activity), reserving chronic medications only for strongly symptomatic patients. RLS drugs include dopaminergic, opioids, alpha-2-ligands, benzodiazepines and iron supplementation. No instrumental finding is required for RLS diagnosis, clinical evaluation is considered sufficient to both diagnosis and to attest the efficacy of medications.
Reasonably, the goal of PLMD and RLS therapy should be to ameliorate subjective symptoms while stabilizing sleep structure and limiting the dangerous autonomic consequences of these conditions (If so, instrumental sleep recording should not be suggested for both diagnosis and follow-up?).
PLM and RLS pathogenesis
Little is known about the pathophysiological differences between RLS and PLMD, frequently coexisting in the same patients and, sometimes intermingled with other sleep disorders (53, 54). Abnormal threshold arousability has already been hypothesized in RLS patients', presenting unusually high cerebro-spinal fluid (CSF) concentration of the wake-promoting hypocretin-1 (55), increased reciprocal connections between sensory-motor cortices and subcortical nuclei (56) brain iron deficiency and hyperexcitability of the spinal cord (57). On the background of genetic predisposition, various external “acquired” factors may modulate the appearance and severity of the disease (58, 59). Accordingly, RLS is considered a “network circadian disorder” with complex multifactorial pathophysiology. So far, less is known regarding PLMD relationship with individuals' arousal threshold and/or pathophysiological mechanisms. It is assumed that PLMD reflects a supra-tentorial/spinal hyperexcitability, maybe favored by dopaminergic dysfunction (60), however given the complex embedment of the disorder within manifold axes it probably depends on the derailment of multiple circuits.
PLMs and the human “inner oscillatory rhythm”
This interesting result is in line with previous observations suggesting that PLMs may be part of a series of normal rhythmic oscillatory events with 4–90 s wave-lengths in humans including heart rate, blood pressure, respiration and cerebral blood flow dynamics that may find a comprehensive synthesis in CAP (61, 62). In this framework, PLMs have been interpreted as the result of a gradual decrease of cortical inhibition in the brainstem during sleep, in other words, periodic motor movements would be motor epiphenomena of the intrinsic human oscillatory network. Thus, rather than being responsible for sleep fragmentation themselves, they appear in reaction to internal or external perturbative factors (61). Indeed, PLM semiology is known to vary according to sleep stage, body position and external stimuli (63). The former hypothesis is somehow confirmed by the observation that the time-relationship between micro-arousal and PLMs is not always predictable: arousals may precede or follow leg movements or even occur simultaneously (64); accordingly, PLM may both be the cause or consequence of sleep instability (42).
The amount and periodicity of PLMs are probably modulated by the individual's arousal threshold, and, reasonably, LMs take place when disturbing stimuli are not powerful enough to trigger a complete awakening. PLMs are deeply associated to the sleep capacity to protect itself against arousing stimuli; which is a feature of brain resilience that progressively decreases with age, leading to a “para/physiological” increase of PLMs in the elderly. The reason why some individuals, with similar PLMIs, are or are not affected by PLM-related clinical consequences, is still largely unknown. Sleep might be perturbed by at least three types of “arousal reaction” including: cortical arousal, autonomic arousal and movement arousal, each involved in the fight-or-flight response of the brain toward danger, lastly causing sleep fragmentation. Instead of being classified as a sleep-movement disorder PLMD should rather be considered as an arousal disorder. This question is not purely academic, because if PLMD is an epiphenomenon representative of low arousal threshold, then therapy should focus on stabilizing and consolidating sleep itself rather than working on limiting the motor manifestations.
PLM/RLS and the autonomic system
Current diagnostic criteria surprisingly disregard the impact of PLM on the autonomic system. A higher PLMI has been associated to a higher risk for atrial fibrillation (65), hypertension (66) and poor cardiovascular outcome (67). The coexistence of PLMD with OSA increases the risk of severe systolic hypertension, important vessel-wall stiffness and systemic inflammation (68), raising the issue on whether PLMD should be considered a cardiovascular risk factor (69). RLS/PLMS patients present decreased parasympathetic tone and an increased sympathetic tone mainly during N2 sleep (70), when PLM index is higher. Autonomic activations with PLMs are detectable even without concomitant arousal reactions (71). LMs with shorter IMI (< 10 s) are associated with stronger cardiac activation and more severe autonomic impact (72). Heart rate variability (HRV) represents an indirect and non-invasive way to measure autonomic nervous system balance (73). RLS patients with a more severe disease phenotype, present stronger sympathetic activation associated with leg movements, a phenomenon which has been suggested as predictive of adverse cardiovascular consequences (52). However, the relationship between RLS and cardiovascular risk is still a matter of intense debate and both longitudinal studies and animal models investigations are desirable (74).
HRV signals are assessed with linear measurements (75). Heart rate spectral analysis leads to the estimation of frequency domain indices including: very low frequency (VLF: 0–0.04 Hz), low frequency (LF: 0.04–0.15 Hz), and high frequency (HF: 0.15–0.4 Hz). Physiologically NREM stages are characterized by a progressive increase of the parasympathetic tone, followed by transient sympathetic predominance during REM sleep. However, NREM sleep stages are not stable; they areinterrupted by phasic EEG activations (including CAP fluctuations, spindles and other electrical phenomena when explored at smaller levels), which are typically associated with concomitant autonomic instability. In detail, during periods of unstable NREM sleep, HRV analysis shows an increase in low frequency and low frequency/high frequency ratio, suggesting a transient shift toward a sympathetic predominance (76). Extensive data have been collected with respect to sleep-related HRV dynamics in patients suffering from insomnia (77), OSA (78) or epilepsy (79). RLS/PLMD has been associated with increase in VLF, LF and LF/HF ratio during all NREM sleep stages (70). More recently, several complex non-linear analyses of HRV, including entropy-driven indices, have been developed to detect subtle changes in the autonomic system dynamics, yielding to better mortality prediction (80). Further studies with either linear or more advanced non-linear measures, exploring the autonomic impact of PLM in both symptomatic and asymptomatic patients are advisable. Given the potential role of the adrenergic system in the RLS/PLMD pathogenesis, some medications acting on the autonomic nervous system have been occasionally tested. In detail, Clonidine, a centrally acting alpha-2 adrenergic agonist, demonstrated a weak effect on the subjective sleep measures, with no relevant variations in the PLM metrics. So far only few controlled studies analyzed the risk/benefit ratio of adopting clonidine for RLS/PLMD (81). Further investigations are required to clarify the role of this molecule in the disease treatment protocols.
Future directions
There is need for a revision of PLMD diagnostic criteria. The raw cut-off threshold of PLM index >15 events/hours is poorly informative (especially in the elderly) and a more “adaptive” cut-off value, corrected for patients' age, is desirable. Other parameters such as the PI may support a deeper understanding of the condition. Perhaps, different PLM index cut-off values could be set for patients with higher cardiovascular risks or with coexistent sleep disorders potentially worsened by PLM motor manifestations. Similarly, high-risk patients with RLS may deserve a polysomnographic exam to check for coexistent PLMD. Furthermore, a comprehensive measurement of PLM dysfunction inclusive of the evaluation of both autonomic impact and sleep fragmentation, would hopefully help in the identification of patients requiring dedicated medical attention. Finally, polysomnographic studies on “asymptomatic” PLMD are advisable.
Sleep disordered breathing
Sleep breathing disorders are multi-systemic conditions with widespread impact on cardiovascular health, mental status, metabolic balance, quality of life, daytime sleepiness, sleep depth and stability, circulating inflammatory biomarkers (82, 83).
OSA is characterized by periodic partial or complete obstruction of the upper airways during sleep associated with intermittent oxygen desaturation, hypercapnia and cardiovascular dysfunction. It is highly prevalent in adults (84). Recently, OSA has been associated with pulmonary embolism (85), proliferative retinal diseases (86) and non-alcoholic fatty liver disease (87). Sleep apneas are extremely common (and frequently overlooked) in patients with cardiac arrhythmia (88) and they increase the risk for adverse maternal outcome in pregnancy (89). Children experiencing sleep apnea are at higher risk for cognitive deficit, its reversibility is not completely understood (90). A strong bidirectional relationship between sleep apnea and neurodegenerative diseases, such as Alzheimer's disease (AD), has been demonstrated (91). OSA and AD share a progressive cerebral accumulation of beta-amyloid (Ab) in the framework of chronic low-grade inflammation and oxidative stress. Apnea-related intermittent hypoxia fuels beta-secretase activity, enhancing amyloid synthesis and accelerating AD pathology (92). In addition, sleep fragmentation in OSA patients hampers the paravascular clearance of toxic molecules, such as Ab and tau, through the night-dependent glympathic system (93).
Given the multiplicity and complexity of OSA affecting several organs and systems, its proper management requires the involvement of a multidisciplinary team with strong expertise in the field. The involved specialists should share a common treatment work-flow, tailoring the treatment of the individual patient and taking into account all indirect implications. Untreated sleep apnea may cause dangerous consequences in peripheral organs and the central nervous system (CNS). OSA features vary according to the degree of physical frailty, sometimes leading to a life-thretening vicious cycle. For example, patients with severe OSA and concomitant cardiovascular impairment may experience the overnight development of central-type respiratory events, reflecting functional exhaustion (94). Patients with COPD (chronic obstructive pulmonary disease) experience a higher risk for decompensation during sleep because of their higher inspiratory muscle tone, increased airway resistance and ventilation-perfusion mismatch (aggravated by the supine position) and frequently suffer from a co-existent sleep-breathing disorder including, above all, OSA. This condition, named “overlap syndrome”, is associated with poorer outcome, increased risk for hospitalization, pulmonary hypertension and cardiovascular events (95). Therefore, OSA patients should always be evaluated in their complexity, focusing on coexistent pathologies, which may impact their outcome and prognosis.
OSA epidemiology
Recently published world-wide investigations, using AASM (American Academy of Sleep Medicine) 2012 diagnostic criteria for OSA (29), estimated that around 1 billion adults (30–69 years) suffer from mild-to-severe obstructive sleep apnea, with higher rates in China, USA, Brazil and India. Nearly 425 million individuals may be affected by moderate-to-severe OSA (AHI, apnea—hypopnea index > 15/h) (96).
The increasing burden of obesity, diabetes, physical inactivity and aging likely support the continuous growth in the global prevalence of the condition.
Notably, a wide community-based longitudinal cohort study in Louisiana (the Bogalusa Study), that followed 844 children through their middle-age for 35 years, confirmed the increased risk for OSA in the adulthood of children experiencing obesity in their younger ages. The longer the duration of obesity, the higher the risk for OSA in the middle age (97). Targeted educational interventions to prevent obesity in children are of paramount importance for blocking the escalation of OSA.
The pathophysiology of OSA
Those mechanisms favoring the appearance of sleep apneas can be divided to “anatomical factors” and “functional factors”. Besides the well-known role of pharyngeal anatomy and craniofacial factors, additional, non-anatomical features need to be considered in the pathogenesis of OSA (98). In synthesis, main contributors of OSA encompass: oxygen saturation level in restful wakefulness, anatomical predisposition, upper airway reflexes, body position, sleep stages, sleep instability, arousal threshold, loop gain and concomitant sleep disorders. Non-anatomical traits are increasingly recognized as predictors of treatment outcomes including residual apnea and adherence to therapy, and can be targets of treatment. The improved understanding of upper airway neurochemical control is seeing potential application in pharmacotherapy of OSA with norepinephrine re-uptake inhibitors combined with cholinergic antagonists or sedatives.
Basal oxygen saturation level
The oxygen-hemoglobin curve, representing the proportion of hemoglobin saturated by oxygen, has a well-known sigmoid shape. This means that, moving to the left, the curve becomes steeper and hemoglobin de-oxygenation can occur easily. Each factor promoting this left-side shift (lower PO2%, increased PCO2%, decrease in temperature) will reduce the hemoglobin affinity to oxygen (99). This “left-sided” scenario is typical in patients with chronic respiratory diseases and explains the higher risk for marked and deeper nocturnal desaturation in this group. Basal wakefulness O2% saturation level helps to predict how far the patient is from the critical threshold for hemoglobin desaturation.
Anatomical predisposition
Anatomical predisposition to upper airway obstruction in OSA patients includes: brevity of the mandible and uvula; enlargement of the tongue, uvula or pharyngeal walls; retropalatal/retroglossal obstruction. Obesity may also promote upper airway collapse due to fat accumulation in the soft tissue. Pharynx in OSA patients is typically smaller in diameter and face greater variations during sleep due to its higher compliance (100). All the listed variables may increase the risk for phasic collapse during nocturnal breathing. The collapsibility of the upper airways during sleep can be quantified and is named Pharyngeal critical closing pressure (Pcrit) (101). Typically, OSA patients differ from healthy sleepers by a greater tendency of upper airway collapse, as mirrored by higher values of Pcrit (~ +5 cmH20). Patients with higher Pcrit values are those who will probably benefit from PAPs therapies (“anatomical predisposition dominant”). Conversely, OSA patients with normal nocturnal Pcrit are probably strongly influenced by non-anatomical factors that should be investigated to tailor the most effective therapy (“non-anatomical predisposition dominant”) (102). As Pcrit measurement is not available everyday, newer non-invasive tolls are needed for categorizing OSA patients with respect to their upper airways' anatomical characteristics.
Body position
In the last years it has been suggested that overnight rest in clinostatism could lead the fluid shift from the lower part of the body, where the fluid accumulate in the daytime in fluids overload condition, to the upper body compartments “cause of gravity effect, raising the neck circumference during the night, increasing the collapsibility of the upper airways and, in OSA patients, exceeding the critical closing pressure that finally leads to sleep apnea (103). Furthermore, obese patients experience an increased risk for nocturnal sleep breathing disorders at bed rest, due to their reduction in diaphragmatic activity, thoracic compliance and higher risk for air trapping (104).
The fluid shift reduction through salt and fluids restriction, diuretics and physical activity combined with positive airways pressure and oral appliances could improve the airways obstruction and, consequently, the OSA severity.
Loop gain
Loop gain refers to the reactivity of the respiratory control system to external or internal perturbations. Loop gain is a system composed of three components: (1) plant gain (lungs), (2) time of delay in circulation and (3) controller gain (chemoceptors). Higher loop gain may induce over or undershooting of ventilation to disturbing stimuli (105). Hence, a patient with “high loop gain” reacts massively to minimal perturbations and is at higher risk for iatrogenic central breathing patterns during non-invasive ventilation, while patients with low loop gain, maintain a stable ventilation (106). Although this difference may influence CPAP efficacy and patients' compliance (107), it is hard to measure or even recognize abnormal loop gain in the standard clinical setting. Simple breath-holding maneuvers have been proposed as an indirect index for loop gain estimation: shorter maximal breath-hold duration and larger post-apnea ventilatory response are commonly associated with higher loop gain during sleep (108).
Loop gain can be reduced with O2-therapy, carbonic anhydrase inhibitors, hypnotics or zonisamide (109, 110). Once again, personalized approaches are desirable.
Sleep stages
Sleep influences OSA dynamics through various modalities. Some patients are affected by a CAP-dominant sleep apnea, presenting significant prevalence of phasic respiratory events during NREM sleep, with a strong temporal correlation with NREM sleep CAP fluctuations (111). In these cases, the sleep clinician should dedicate major efforts in improving (NREM) sleep stability. Conversely, other patients show a relatively stable breathing pattern during NREM sleep and are more affected by the muscle atonia and autonomic chaos typical of REM sleep, the so-called REM dominant OSA. In REM-OSA, upper airways are typically more collapsible, while loop gain is lower and arousal threshold is higher during NREM sleep (112).
Sleep instability
Sleep apnea can profoundly disturb sleep stability: affected patients typically present lower amounts of stage N3 with a parallel increase of superficial sleep and an impressively high amount of sleep instability, as demonstrated by higher CAP rate. To what extent OSA can hamper sleep continuity strongly depends on disease severity. Indeed, it is well-known that in moderate-to-severe OSA, sleep texture is characterized by a higher percentage of the more disturbing CAP subtypes A2 and A3 (113).
More recently, it has been shown that in mild OSA the CAP subtypes A1 (slow wave arousals) still prevail and “try” to reinforce sleep continuity. Conversely, in moderate-to-severe OSA, the more disturbing/arousing subtypes A2 and A3 prevail and reverse the sleep-stabilizing role of CAP system, evolving into an intrusive mechanism that finally disrupts sleep continuity (114). Different arousal routes/pathways, depending on the underlying severity of body distresses have also been hypothesized. In this framework the hub-region of the pontine parabrachial nucleus can evoke both “milder” arousal reactions and “stronger” arousal reactions, as mirrored by the appearance of K-complex/delta bursts (CAP subtypes A1) rather than higher frequenciy bands activities (alpha/beta bursts), according to singular conditions (115). CAP dynamics may be used to analyze the dynamics of respiratory-related arousals.
Arousal threshold
The occurrence of transient arousals from sleep after small changes in ventilatory drive is termed “low arousal threshold” and may predispose to obstructive sleep apnea. Not all respiratory events are followed by phasic arousals and, occasionally, arousals occur after the resolution of the sleep apnea (116). Physiologically, the ventilatory drive activates the pharyngeal dilator muscles, preventing sleep apneas. OSA patients with low arousal threshold experience premature arousal intrusion during sleep, increasing the risk for unstable breathing patterns (117). These arousal intrusions can frequently yield to abrupt ventilatory responses with fluctuations in CO2 concentration, lastly fueling the nocturnal instability of respiratory control. Few medications had been proposed to treat OSA in patients with lower arousal threshold (benzodiazepines, Z-drug, trazodone), however their administration might worsen nocturnal hyopxiemia and AHI, as some of them can enhance pharyngeal muscle relaxation and delay arousal, thus worsening hypoxemia. A detailed and personalized evaluation of the risk-benefit ratio is mandatory before trying this strategy.
Apnea duration
According to current guidelines, a nocturnal breathing event must last at least 10 s or more to be deemed as apneas or hypopneas. Mean apnea duration is a frequently used parameter to estimate respiratory events' length. Debate is still ongoing on whether longer or rather shorter apneas should be considered at higher risk for cardiovascular consequences (118, 119). In their investigation Sarac et al. revealed that morning tiredness, sleep fragmentation, reduced blood oxygenation and hypertension were more frequent and severe in patients with longer apnea duration (119). Longer apneas probably reflect a progressive increase in the arousal threshold, which may partly occur with age, and, in parallel, a delayed response to oxygen desaturation. Conversely, shorter breathing events may rapidly accumulate resulting in higher AHI scores per hour, automatically worsening the severity of the condition.
Concomitant sleep disorders
Routine clinical practice is often complicated by the overlap of sleep apnea with other sleep disorders (PLMD, NREM sleep parasomnia, epilepsy, RBD, narcolepsy). Coexistent sleep pathologies may lower patients' compliance to OSA treatment, and untreated sleep apneas may trigger abnormal arousals leading to NREM sleep parasomnias or nocturnal seizures (120). The relationship between OSA and epilepsy is a complex and bidirectional one: epileptic patients with untreated OSA experienced more nocturnal seizures and, in drug-resistant epilepsy the prevalence of OSA is estimated around 33% (121, 122). The two conditions share the reduction of REM sleep that had been proposed as a biomarker for epilepsy severity/drug-refractiveness (120, 123). Accordingly, the restoration of REM sleep should be included as one of the main goals of OSA and epilepsy therapies (124). Current recommendations for non-invasive ventilation management in OSA patients state that 4-h of nocturnal CPAP therapy can be considered “enough”. However, as REM sleep physiologically predominates in later parts of the night, we are wondering if this could guarantee sleep and health amelioration in OSA patients.
PLMD may also be associated with phasic respiratory events, mostly hypopneas. These respiration- related movements, according to current guidelines, should not be included in the final computation of AHI index. The two conditions (OSA and PLMD) are modulated by the same permissive “windows” for cerebral and autonomic activation, mirrored by the CAP phases A (motor activation) and B (respiratory inhibition). A recent multicenter randomized controlled trial explored the prevalence of PLMS in a large cohort of 1,105 OSA patients (125). According to their results around 19.7% of OSA patients had PLM index (PLMI) ≥10/h and 14.8% had PLMI ≥ 15/h, with higher risk among the elderly and in those patients taking antidepressants or higher doses of caffeine. Patients with higher PLMI presented worse sleep quality and, notably, 6-months of CPAP therapy did not modulate PLM severity, suggesting that great efforts should be dedicated to the diagnosis and the concurrent management of the condition (123).
Central sleep apnea (CSA) can coexist in OSA patients for several reasons: CSA, especially when a Cheyne Stokes breathing pattern is recognizable, can indicate an underlying cardiovascular disorder (e.g., congestive heart failure); in other cases CSA can be associated to opiod intake, the alternation of hyperventilation and underbreathing with central-type respiratory pattern can appear at very high altitude or be associated to chronic disorders (e.g., end-stage kidney disease). Lastly, CSA can be iatrogenic in OSA patients treated with PAPs devices, the so-called treatment-emergent central sleep apnea (TECSA), which can be associated to various potential mechanisms including ventilatory control instability, low arousal threshold, activation of lung stretch receptors, and prolonged circulation time.
Clinicians must dedicate efforts in the understanding of the simultaneous presence of OSA and CSA, as this binomius can be associated to numerous heterogenous conditions.
OSA and the autonomic system
OSA patients present nocturnal autonomic dysregulation, which can be worsened by coexistent somatic (e.g., hypertension, diabetes) or psychological (e.g., stress, depression) conditions similarly associated with an altered sympatho-vagal balance (126). Sleep exerts direct influence on vegetatitive dynamics shifting between a parasymphatetic (NREM sleep) and sympathetic (REM sleep) dominance (127). Apneic episodes lead to lung inflation, favoring sympathetic activation, which promotes phasic increase of blood pressure and heart rate. Through a baro-reflex mechanism, the post-apnea re-opening of the upper airways determines a transient withdrawal of sympathetic stimulation (78). HRV is one of the most widely used markers of autonomic functioning. HRV high frequency (HF) power (0.15–0.4 Hz) reflects a vagal dominance, whilst low and very low HRV frequency (0.04–0.15 Hz) are associated with both sympathetic and parasympathetic activation. Sleep apneas are associated with increase in LF and decrease in the complexity of heart rhythm (128).
OSA complexity and subtypes
The current denomination of OSA is partially misleading, referring, as major determinant of the condition, to the focal and phasic obstruction of the upper airways during sleep. Accordingly, sleep apnea management is commonly attributed to the expertise of pulmonologists or ENT specialists. Although the role of these specialists is beyond questions, the multi-facet aspects must never be neglected. The disease might better be defined by the inclusive term “sleep disordered breathing”, where the complexity of sleep mechanisms are taken into account.
As explained before, sleep apneas and hypopneas can be heterogeneous events. Whereas, the term “phenotype” typically refers to different clinical manifestations, the concept of endotype tries to include more details on the disease pathogenesis. Distinct sleep apnea endotypes have been proposed, depending on numerous features such as anatomical characteristics, arousal threshold and ventilatory control stability (129).
The multinational ESADA (Sleep Apnea Network/European Sleep Apnea Database) group (a pan-European, multi-center research group) dedicated great efforts in the characterization of different OSA clusters (130). Applying the latent class analysis to large data sets they were able to describe eight distinct phenotypes: Four based on gender and four based on a combination of age-bands, BMI (body max index), AHI and burden of comorbidities (see Bailly et al. for details). Once again, the authors emphasized the importance of a tailored, gender-based and comorbidities-based evaluation of OSA patients.
OSA can also be “sub-classified” according to symptom-severity or to coexistent cardiovascular comordities (131). The role of comorbidities in the “OSA scenario” (named CoSA or Comorbidities of Sleep Apnea) have recently been proposed to stratify patients according to their global clinical burden (132). It seems that most OSA patients suffer from at least 3–4 comorbidities and, the number of comorbidities may help to predict outcome and mortality.
Disregarding the complexity of the disease raises the risk of oversimplifiing pathology, overlooking important features, and lastly, augmenting the risk of treatment failures.
OSA therapies: A multidimensional approach
OSA complexity also reflects in its management. As for many other chronic conditions it is key to guarantee periodic patients' re-evaluation, essential to guide a tailor approach according to individuals' condition, that might largely change with variations in body weight, cardio-vascular comorbidities, medications. This also requires a multidimensional approach, with a dialogue between numerous specialists: neurologists, pneumologist, otolaryngologist, maxillofacial surgereons, cardiologists and endocrinologists.
OSA patients should always be encourage to pursue on some life-style changes (physical exercise, weight loss, alcohol and smoke avoidance) and must pay attention to sleep hygiene. The condition then might be treated using positive airways pressure (including auto titrating-CPAP, fixed CPAP, BPAP or ASV in selected cases), oral devices, surgical removal of tissue (commonly uvulopalatopharyngoplasty, nasal surgery), hypoglossal nerve stimulation, maxillomandibular advancement or bariatric surgery. Few medications can be adopted in OSA treatment, including the recently approved Solriamfetol to tackle with residual excessive daytime sleepiness, as detailed below. Some other drugs can worsen the condition (e.g., sedative medications) and therefore should be discontinuated, when possible according to patients' condition.
OSA severity: Time to move beyond the AHI
Currently, sleep apnea diagnosis relies on simplified data such as AHI, with no consideration of the direct or indirect signs of sleep fragmentation, comorbidities and autonomic consequences each infuencing outcome.
In recent years worldwide, major research efforts have been cowed out to “move OSA beyond the AHI index”.
Respiratory events may reflect different pathogenetic mechanisms and variable clinical implications: central periodic breathing is typically less intrusive, with lower autonomic stress. Obstructive events are typically abrupt and disturbing, leading to an increase of negative intra-thoracic pressure and powerful autonomic activation. Respiratory events are usually longer during REM sleep, with more severe and longer lasting desaturations, while mixed apneas may mirror the fatigue of the cardio-respiratory system (133). Central sleep apneas must be distinguished from the “treatment-emergent central sleep apnea”, appearing during CPAP therapy (134). Finally, patients with pre-existent pulmonary diseases will experience deeper oxygen desaturation in relation to milder respiratory events, due to their lower basal SatO2% level. Intuitively, such a complex scenario cannot be summarized in a raw index such as AHI.
Recently some novel biomarkers for OSA severity have been proposed including: AHI in REM sleep stage (REM-AHI), nocturnal hypoxic burden, the pulse rate response to apneas/hypopneas (ΔHR) and others (135–137). The hypoxic burden, calculated as the oxygen desaturation “area under the curve” in association with individual apneas and hypopneas, appears to associate with incident heart failure. Thus, it could be a useful marker identifying patients with higher cardiovascular risk (137).
Recent proteomic investigation reveals distinct protein signature in obstructive and central sleep apnea: OSA presenting disturbances in the expression of various proteins involved in coagulation, inflammation, growth factors and hemostasis; central sleep apnea associating with an abnormal proteomic profile in molecules involved in the balance of the pre-Bötzinger complex (a cluster of interneurons in the medulla), essential for the generation of the spontaneous respiratory drive in mammals (138). Metabolomic and microbioma investigations provided confirmations for these findings highlighting the roles of certain metabolites related to fatty acid, carbohydrate and amino acid metabolism in the pathophysiology and cardiovascular complications of OSA (139).
In summary, AHI oversimplifies the characterization of OSA severity and could not be used alone to predict patients prognosis.
The importance of the follow-up plans
OSA severity typically progresses with patients' aging. Compliance may decline with time and the adherence to therapy is the major limitation of CPAP therapy. A minimum of 4 h per night for at least 70% of nights is considered essential to ensure therapeutic efficacy of non-invasive ventilation. There is a dose-response relationship between CPAP utilization and benefits on symptoms (daytime sleepiness) and other health consequences (hypertension) (140). As REM sleep typically prevails during the second part of the night, the 4-hours' cut-off of nocturnal ventilation may not be entirely sufficient to guarantee a proper management of OSA as discussed above. REM sleep is frequently curtailed in OSA patients and there is a rapid REM-rebound after the introduction of CPAP (or BIPAP) therapy. It is likely, that for preserving REM sleep integrity, a longer nocturnal PAP-use should be required.
Regular follow-up visits to guarantee patients' compliance are also of pivotal importance in the clinical assessment of OSA patients. As already outlined, OSA is a chronic and dynamic condition, warranting periodic re-evaluation. For exemple, the nocturnal breathing pattern might change after acute or chronic cardiovascular events, with the appearance of central-type/mixed-type of events, imposing variations of PAPs parameters and, sometimes, the shift from a CPAP to an adaptive servo-ventilation (ASV) machine or to a BiLevel positive airway pressure (BiLevel) therapy. The activity and involvement of a sleep-team with doctors and technicians is necessary. CPAP prescription is the very first therapeutic acion of a long-lasting clinical dialogue between sleep centers and OSA patients.
OSA and hypersomnolence
The reason why EDS is common in patients with severe OSA is still under debate. In 1993, a higher prevalence of hypersomnolence was found in individuals with an AHI ≥ 5 compared to those with AHI < 5, and, in individuals with an AHI < 5, a higher prevalence was found than in habitual snorers (141).
In a recent debate on the relation between sleep fragmentation and hypersomnolence in OSA, Punjabi and Lim (142) declared that arousals are the causative elements for daytime sleepiness in sleep apnea. Airway collapse or closure can lead to hypercapnia and increase the breathing work, which may contribute to impairment in daytime alertness. However, both are likely to mediate their effects through arousals from sleep and disruption of sleep continuity. Murine models have shown that exposure to long-term intermittent hypoxia, in the absence of sleep fragmentation, results in oxidative neural injury to wake promoting neurons and this injury could also be responsible for daytime sleepiness (143). With such evidence, it is reasonable to conclude that both sleep fragmentation and intermittent hypoxemia contribute to the development of daytime sleepiness in sleep apnea.
In contrast Gold and Gold (144) stated that the sleep fragmentation paradigm accounts for only some of the hypersomnolence identified in OSA patients: at any level of AHI, snorers are generally more somnolent than non-snorers. In other words, snoring frequency is an independent correlate of hypersomnolence, unrelated to sleep fragmentation by arousals. Both AHI and snoring frequency reflect a single underlying pathophysiologic factor, inspiratory flow limitation (IFL), which is expressed both in the OSA subgroup and in the snoring subgroup. Combining the two groups into one population there is only one fundamental predictor: IFL. Investigators automatically equate increasing AHI with increasing sleep fragmentation rather than choosing to equate it with increasing time spent in IFL. If the most direct causes of sleep fragmentation are arousals, wouldn't one expect to easily demonstrate a correlation between hypersomnolence and arousal frequency?
Punjabi and Lim (139) reply that scoring of arousals based on conventional criteria completely neglects an entire family of subcortical physiological events that reflect central nervous system activation such as the autonomic surges and the appearance of delta bursts or K-complexes in the electroencephalogram (EEG). To focus only on arousals is akin to only seeing the tip of the iceberg and neglecting the complexity of events that characterize sleep instability. Because sleep fragmentation is commonly operationalized using arousals, the omission of other, more sensitive, measures of sleep state instability (CAP) could certainly explain the poor association between arousal frequency and daytime sleepiness in sleep apnea. In large population based samples, individuals with OSA can be hypersomnolent and yet not have lighter sleep than those without hypersomnolence. Comparing polysomnographic parameters between sleepy and non-sleepy subjects with moderate to severe OSA (n = 1,115) Kapur et al. showed identical total sleep time, sleep efficiency, arousal index and sleep stage distribution in the sleepy and non-sleepy groups (145). In 2007, Guilleminault et al. (146) have showed that patients with UARS (upper airway resistance syndrome) have higher EEG arousal indexes and important NREM sleep disturbances that correlate with subjective symptoms of sleepiness and fatigue. These disturbances are identifiable with sensitive measures such as CAP analysis but not with traditional diagnostic and scoring systems. All 30 patients with UARS reported chronic fatigue, 28 reported non-refreshing sleep, 26 reported disrupted nocturnal sleep, 17 reported morning headache, and 29 reported daytime performance impairment. The mean ESS was 8.5 in UARS patients compared to four in healthy controls. According to the UARS criteria outlined by Bao et al. (147), syptomatic subjects must have an apnea-hypopnea index of 5 or less, a minimum oxygen saturation >92% during nocturnal sleep and mild upper airway collapsibility. In 2018, Korkmaz et al. (148) divided 38 male patients with moderate to severe OSA into two subgroups, with and without daytime sleepiness (ES) based on the ESS. While there was no difference in clinical characteristics and sleep macrostructure between the two groups; OSA patients with ES had increased CAP measures compared to those without ES: more disruption of sleep continuity is associated with more ES. In 20 male individuals with OSA, the occurrence of CAP A-phases (cortical activation) and PWA drops (below 30%) was quantified in relation to apneas, hypopneas or flow limitation events. A dual response (A-phase associated with a PWA drop) was the most frequent response for all types of respiratory events, with a progressive reduction from apneas to hypopneas and IFL. The highly significant correlation in total sleep time (r = 0.9351; P < 0.0001) between respiratory events combined with A-phases and respiratory events combined with PWA drops was confirmed both in NREM (r = 0.9622; P < 0.0001) and REM sleep (r = 0.7162; P < 0.0006). In conclusion, a dual cortical and autonomic activation is the most common manifestation at the recovery of airway patency. These findings indicate a close temporal connection between events upstairs (EEG) and downstairs (autonomic system). The problem is the tools we use to investigate the body language during sleep. Vegetative microevents (apneas, hypopneas, IFL, PWA drops) require a repertoire of neurophysiological microevents (CAP phase A and B). EEG arousals can provide useful information but they carry two major inconsistencies: (1) they are the iceberg tips of a wide spectrum of activation features which include also K-compelxes and delta bursts; (2) they offer a static and limited picture (< 15 s) of a more dynamic condition of sleep instability (CAP sequences) which can last several minutes and translate the alternating double-face oscillation of activation (phase A) and inhibition (phase B).
In conclusion, in OSA patients, unstable sleep and the multifold features of EEG activation (A phases of CAP) play a topical role in ES, while IFL reflects milder disruption of sleep consolidation and continuity. However, ES is a multifactorial condition, which can be independent of AHI, arousal frequency or CAP parameters. OSA is not a prerequisite of hypersomnolence, which, it can be triggered by a trivial drop of blood sugar levels. In narcoleptic patients, ES is attributed to reduced amounts of hypocretin in CSF but whether hypersomnolence is also fueled by nocturnal sleep disruption and a compared to healthy controls remains an open question. A patient with Kleine-Levin syndrome showed small intenstine overgrowth and the recurrent episodes of ES vanished after regular treatment with local-acting antibiotics (149). These considerations do not clarify the puzzling nature of hypersomnolence, but certainly indicate that the pathways pursued so far by the community of sleep experts need novel and alternative routes.
OSA and COVID-19
Sleep breathing disorders (SBD) are considered risk factors for COVID-19 severity, with prevalence reaching up to 28% of patients in ICU cohort (150).
Untreated SBD, especially when associated with severe nocturnal hypoxemia, may lead to chronic oxydative and inflammatory damage, increases the risk for acute vascular disease and might contribute to worsen the clinical outcome in post-COVID cohort (151).
There is an impressive clinical overlap between subjects affected by severe COVID-19 disease and OSA patients (middle-aged, obese male patients affected by metabolic syndrome).
Although epidemiologically the two conditions are associated, it is still unclear whether the utilization of non-invasive ventilation devices (PAPs) in moderate-to-severe OSA may or may not prevent from COVID-19 life-thretening consequences. The issue is not trivial as in many countries, during the peak of the pandemia, the utilization of PAPs was discouraged by numerous international societies due to concerns on virus diffusion. Furthermore, the outbreak reduced the connection between patients and healthcare providers, reducing OSA patients' compliance to therapy. However, PAP devices are known to promote numerous cardiovascular protective effects, reduce hypercoagulability decreasing platelet activation, clot strength, hematocrit and lowering blood viscosity while increasing functional residual capacity and thereby improving gas exchange.
Further studies are advisable to assess the relationship between OSA, COVID-19 and well-performed PAP therapy (152).
Future directions
Sleep teams should evaluate OSA patients in their complexity, trying to embed them into phenotypes and endotypes for tailored therapy. A dialogue between all involved specialists is essential to guarantee effective management, monitoring co-existent or developing systemic and sleep-related comorbidities. Regular follow-up is essential, for ensuring compliance and update the dynamics of the condition.
DOA: NREM parasomnias
DOA are prevalent conditions: they affect up to 13–39% of children and around 1.6–4% of adults, where they can appear “de novo” or persist since childhood (153, 154).
Whereas, NREM parasomnias are usually harmless during childhood, violent behaviors, with even forensic implications, can occur in their adult counterparts (155–160).
Sleep deprivation as well as any factors leading to sleep fragmentation (via increasing the homeostatic pressure) are considered the most important triggers for these conditions.
DOA episodes typically start with an abrupt arousal from NREM sleep during the first sleep cycle, when it turns from the descending to the ascending slope. As a consequence, the frontal motor regions partially arise, while the dorso-frontal areas continue sleeping, yielding to a dissociated sleep/wake condition. Several studies have evidenced this brain state dissociation during NREM parasomnias (161–163).
DOA therapy
DOA should be treated focusing on comorbid sleep disorders and eliminating potential external triggers (e.g., factors increasing sleep fragmentation such as noise or pain, or factors augmenting sleep inertia such as circadian misalignement). Numerous drugs have also been associated with the condition (sodium oxybate; various antidepressants including amitriptyline and buproprion; antipsychotics drugs; metoprolol and topiramate) and, therefore, they should be discontinuated when possible. Clinicians must always educate patients on the importance of a safety bedroom environment, in order to reduce the risk for sleep-related injuries. Usually DOA patients do not require specific therapies. However, when clinically necessary (high frequency of episodes, sleep-related injuries, excessive daytime sleepiness, psychological consequences), few approaches can be pursued, either with cognitive-behavioral strategies or using pharmacotherapy. Clonazepam is still the most commonly adopted first-line agent, alternatively some antidepressant medication can be tried, preferring those with a strong serotoninergic effect (e.g., paroxetine) (164).
Sleep-related hypermotor epilepsy, the epileptic counterpart of DOA
SHE incorporates any sleep related epilepsy with hypermotor seizures, irrespectively of etiology or types of movements. Most SHE cases are sporadic, while a minority has an autosomal dominant genetic background, associated gain-of function mutations, the best known one affectingthe nicotinic acetylcholine receptor (nAChR) gene subunits (165, 166). The electro-clinical symptoms do not permit a clear-cut discrimination between the genetic and sporadic forms (167).
SHE can be particularly challenging for the clinician as interictal epileptiform discharges (IEDs) hardly occur either in wakefulness or during sleep, detected in < 50% of patients (168). Movement artifacts can cover IEDs and ictal EEGs and the scalp EEG may not be able to reveal a deep-located seizure onset zone. Conversely, when SHE is associated with a structural etiology, abundant IEDs may emerge easing the diagnostic work-up; this is typically the case with focal cortical dysplasias (169).
In 2017, Licchetta et al. (170) studied the etiology and long-term outcome in a large cohort of SHE patients. In this analysis SHE was sporadic in 86% of cases, familial in 14% and associated with structural etiology in 17% of cases. At 30-years of follow-up the overall remission rate was 28.4%.
There is an interesting overlap between NREM parasomnias and SHE. Literature data highlighted frequent positive family histories for both epilepsy and parasomnias in SHE and DOA patients (155, 168), and the lifetime prevalence of DOA episodes seems higher among SHE patients' relatives compared to healthy subjects' families.
SHE therapy
SHE cases associated with cortical malformations should always be directed to a pre-surgical evaluation. Genetic etiology does not represent a contraindication for surgery. SHE cases with unknown origin are usually treated pharmacologically. Low doses of carbamazepine still represent the most commonly adopted pharmacological strategy for SHE patients. As an alternative, oxcarbazepine, lacosamide or other antiepileptic medications can be used, especially in cases non-respondent to carbamazepine. Fenofibrate, a lipid-modulating drug acting as an agonist of peroxisome proliferator-activated receptor-alpha, demonstrated some benefits in drug-resistant cases (171).
Commonalities between DOA and SHE
The clinical similarities and differences between DOA and SHE were well shown by the Derry study (172) comparing the semiology of 63 SHE seizures with 57 DOA episodes based on video-EEG monitoring. Three main patterns appear; usually in mixed forms: (1) Simple arousal behavior: eye opening, head elevation, staring, face rubbing, yawning, stretching, moaning, and mumbling; (2) Non-agitated motor behavior: sitting forward, manipulation of nearby objects with a passive or perplexed facial expression. Coherent speech-fragments were frequent; (3) Distressed emotional behavior: fear marked by facial expression or speech content; screaming, violent movements. Most of parasomnia-related events and nocturnal seizures began with an arousal, but only 25% of DOA vs. 88% of seizure terminated in waking. SHE episodes ranged from minor motor events, paroxysmal arousals and major attacks.
The semiological overlaps and major differences between DOA and SHE have been explored by Loddo et al. recently (173). The most important finding of these studies, is the semiological similarity between the two groups, both presenting with an increasing complexity in terms of motor pattern, raising the possibility of a continuum between the two conditions.
The well-known major differences are represented by the number of episodes/night (multiple in SHE vs. one or few in DOA); stereotypy, dystonia and hypermotor features, which are distinctive for SHE; a stronger correlation with external triggers (e.g., noise) in DOA. Both types of manifestations link to NREM sleep microarousals (174). This is in accordance with the finding that DOA episodes occur at the turning point of the first NREM sleep cycle, where deepening turns to rising, while seizures link to CAP A phase, paralleling the overnight homeostatic decay. This implies, that both DOA and SHE strongly correlate with the first sleep cycle occurring within a few hours after sleep initiation.
Notably, both DOA and SHE share a relevant increase of CAP fluctuations: in the two conditions there is a significant increase of CAP subtypes A1, whose appearance frequently precidict the beginning of an (either epileptic or parasomniac) episode. Indeed CAP A1 can be described as permissive framework for highly synchronized nocturnal episodes.
In summary, the two conditions share similar mechanisms (disordered arousal during NREM sleep stages), involving the derailment of sleep-related brain circuits yielding to the development of similar hypermotor symptoms, and manifest intra-individual and family overlaps (170, 175–177) (Figure 3).
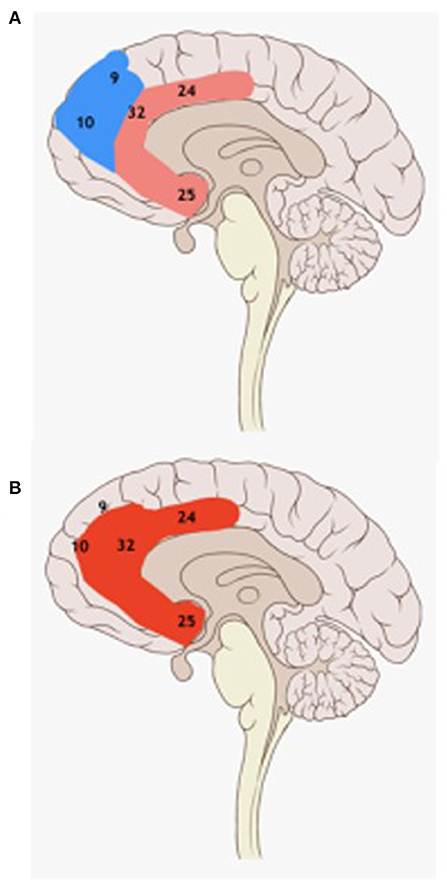
Figure 3. Schematic drawing of the medial brain surface with Brodmann areas. Pink: prefrontal lobe, light red: the pyramidal system (A); red: the anterior cingulate area (Br. 24, 25, 32) (B). There is a dissociation during DOA episodes between the blue fronto-dorsal cortex (in partial sleep) and the red anterior cingulate cortex (in partial wakefulness). SHE seizure onset zones of successfully operated SHE patients frequently overlap with DOA episodes' activated red anterior cingulate area.
Open questions
There are still various unanswered questions regarding both DOA and SHE.
First of all, the definition of SHE has loose boundaries: it involves several types of etiologies, the EEG is highly dishomogeneous and the level of sleep relatedness also appears variable: the spikes of Taylor type focal cortical dysplasia link strongly to sleep, while other forms are less sleep-related (178). Thus, a doubt has persisted on the uniformity of the SHE groups (120, 176, 179).
Also, the definition of “sleep relatedness” needs an extension. Traditionally, only seizures were taken into account, classifying SHE into the sleep-related group. However, the significance of IEDs embedment within sleep texture seems similarly important. Following such approach MTLE (high level of spiking in sleep), and/or absences (rhythmic spike-waves promoted by sleepiness/sleep transition into NREM sleep) can be considered sleep-related epilepsies.
The relationship between arousals and sleep in DOA and SHE is still a matter of debate: two recent studies (120, 159) highlighted the role of the fascinating clash between sleep and arousal forces in triggering seizures/events.
Congruent data support an augmented propensity toward arousal in both DOA episodes and seizures.
In deep NREM sleep stages, arousals can hardly evoke full awakening. In DOA and SHE, partial arousals may result in “dissociated” states. DOA episodes occur when strong ultradian forces turn deep NREM sleep toward arousal/sleep lightening, while seizures seem to link to less intense arousal-fluctuations. Thus, a stronger clash of sleep-wake forces is needed for DOA episodes to occur, whereas SHE can develop more frequently at the less intense collisions of sleep protecting and sleep promoting forces represented by CAP fluctuations.
Therefore, it is evident that the two conditions share some fascinating commonalities.
It is also interesting that there is a partial topographic overlap between brain areas involved in the DOA pathogenesis and the seizure-onset zones in successfully operated SHE patients.
Based on SPECT and electrophysiology studies on DOA patients (162–165, 180) sleep-dissociation consistently localizes to the cingulate cortex (partially awake) and the fronto-dorsal cortex (partially sleeping). Those regions may have different “arousability” thresholds (Figure 3).
Epilepsy surgery experiences show that SHE seizures originate from the anterior cingulum (181–184) and the prefrontal-medial cortex in a higher proportion of cases (185).
The involvement of similar brain areas in seizures and DOA episodes confirms the analogies in their pathophysiological mechanisms and may emphasize the key role of the medio-fronto-cingular cortex for emotional arousal from NREM sleep.
Theories about the origin of hypermotor phenomena in DOA and SHE
The origin of ictal hypermotor phenomena and panic/agitated DOA behaviors is still a matter of debate; it is historically known that the frontal lobe is likely involved. It has been supposed that SHE seizures may result from the activation of subcortical generators: when the physiological top-down frontal inhibition due to seizure activity ceases, those atavistic (animal or infant) movements arise and may disengage as described in the “music box” approach of Tassinari (186). The dissociation concept is even better seen in DOA (162, 163): the cingulate cortex is active while the fronto-dorsal cognitive field sleeps. The hypermotor semiology may have a combined, evolutionist reason: due to the evolving arousal, the half-sleeping individual experiences threat, developing an alarm reaction with the activation of innate ancient hypermotor patterns (187).
The movement patterns and complexities in SHE and DOA are also variable, reflecting localization, spread and propagation-speed of this phenomena from or to eloquent brain regions (188, 189).
Future directions
Advanced electrophysiological studies on polysomnographic recording and computer-aided neuroimaging protocols (morphometric brain MRI, EEG-fMRI and other techniques) might provide some novel insights into the mechanisms of DOA and SHE pathogenesis, to assess the magnitude of similarities between the two conditions. This may support a deeper understanding of the concealed link between these two sleep-related hypermotor conditions.
REM parasomnias
REM parasomnias include several peculiar conditions; REM sleep dissociation symptoms as REM sleep behavior disorder (RBD), recurrent isolated sleep paralysis, nightmare disorder; and other variable syndromes e.g., sleep related painful erection (29, 190). In REM parasomnias an admixture of wakefulness and REM sleep has been theorized, as explained below. We overview REM parasomnias focusing on REM sleep behavior disorder (RBD).
Short physiology background
The pattern of REM sleep, also called “paradoxical sleep” is made by the combination of striated muscle atonia, rapid eye movements and a wakefulness-like EEG background activity. The EEG is strongly desynchronized, “saw tooth” waves (STW) and rhythmic hippocampal slow waves had been recorded (191, 192).
Jouvet's pioneer cat-brain trans-section experiments have identified the neural circuitry of REM sleep in the brainstem, mainly in the dorso-rostral pons. A twin REM on/off system regulates REM sleep components through multiple ascending and descending trajectories (193–198) (Figure 4).
REM sleep is believed to be strongly linked with mood regulation, creative problem solving and emotional memory consolidation (199, 200). Dream reports can be elicited after awakening from any sleep stages; however, REM sleep is considered the “dream-phase” with longer dreams and bizarre dream-contents (201).
While the low level of acetylcholine in NREM sleep favors the communication of the dorsolateral prefrontal cortex and the hippocampus sustaining the transfer and consolidation of declarative memory traces (202), the high level of acetylcholine in REM sleep promotes an “emotion-driven memory-processing” involving the amygdala, the anterior cingulate and medial prefrontal cortices (203) (Figure 5). The consolidation of fear memories during REM sleep possibly contributes to post-traumatic stress disorder (PTSD) (204). Different abnormalities of REM-sleep e.g., shorter latency, increased REM-sleep density and fragmentation, may participate in additional neuropsychiatric conditions (205).
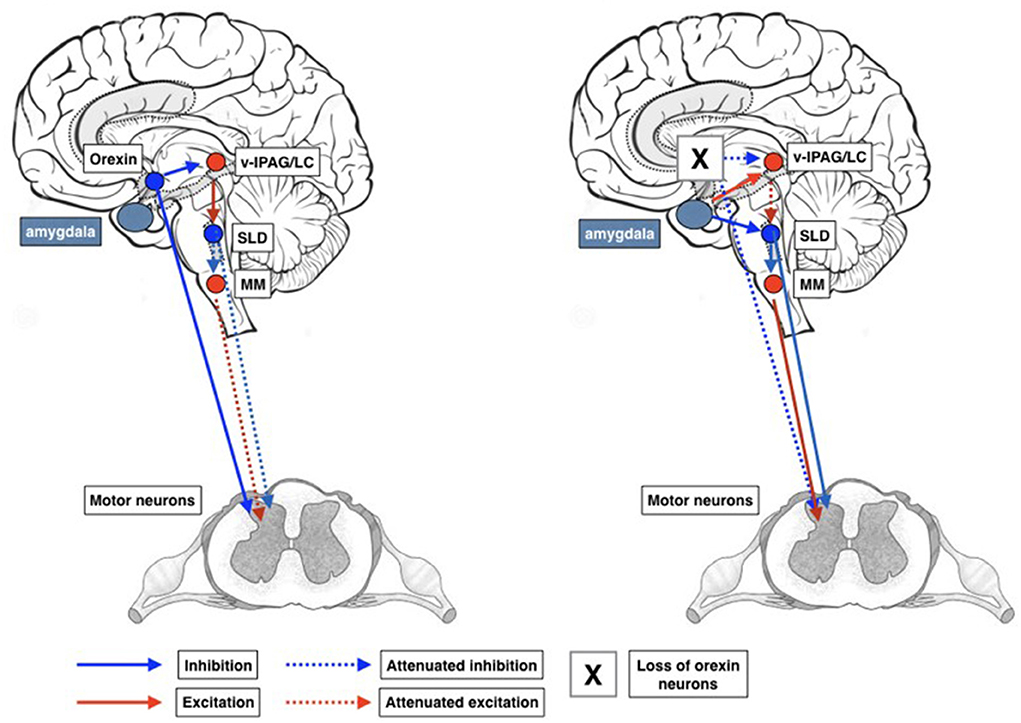
Figure 5. Schematic representation of circuits involved in the development of cataplexy. Inappropriate activation of the REM sleep atonia circuitry during wakefulness is thought to produce cataplexy. Glutamatergic REM-active SLD neurons trigger the paralysis of REM sleep via stimulation of the GABAergic/glycinergic cells in the MM. These MM neurons send inhibitory projections to skeletal motor neurons. Under normal conditions, strong positive emotions are processed via GABAergic neurons of the CeA, which then inhibit cells in the LC and vlPAG. However, in the absence of the LH hypocretinergic neurons in cataplexy, this inhibition fails, so the REM sleep atonia circuit is released from inhibition and triggers muscle paralysis while the individual remains conscious. The inhibition of LC neurons during cataplexy removes noradrenergic inputs to motoneurons, thereby enhancing the muscle paralysis of cataplexy. CeA, central nucleus of the amygdala; GABA, γ-aminobutyric acid; LC, locus coeruleus; LH, lateral hypothalamus; MM, ventral medial medulla; SubC, subcoeruleus; vlPAG, ventrolateral periaqueductal gray; MNs, motoneurons.
Muscle atonia protects the sleeper from “acting out” his/her dreams, and the suppression of motor activities can outweigh certain potentially sleep-disruptive stimuli. In this regard, REM-dependent muscle atonia can be deemed a sentinel of sleep resilience (206).
Regulation of REM sleep
The key members of the REM-related atonia network are the sublatero-dorsal nucleus (SLD) and the noradrenergic pre-coeruleus region (PC) (207) (Figure 4).
The glutamatergic activation of REM-on neurons in the ventral SLD (208) mediates REM motor atonia through two redundant trajectories: recruiting glycinergic interneurons in the spinal ventral horn and GABAergic/glycinergic neurons in the ventro-medial medulla; inhibiting spinal ventral horn motor neurons in both ways.
A direct noradrenergic pathway links the spinal motoneurons with the locus coeruleus, and a serotonergic one with the dorsal raphe (209) both inhibiting the REM-atonia generation of the SLD (Figure 5).
REM sleep parasomnias as REM dissociation phenomena
Sleep paralysis
Sleep paralysis has been described in 1664 (210). Over the centuries, it has often been attributed to the presence of “evil”: demons, the old hag in Shakespeare's Romeo and Juliet…. In this frightening state the affected person cannot move or speak experiencing minutes' lasting paralysis and chest pressure, is unable to call, experience suffocating sensations—something is sitting on his/her chest—or even feeling outside his/her own body. Sleep paralysis resolves spontaneously and can occur as part of the narcoleptic tetrad or solely.
Polymorphisms in the PER2 (Period Circadian Regulator 2) gene, a component of the circadian clock mechanism, have been associated with a genetic predisposition to sleep paralysis (211). Multimodal hallucinations and lucid dreaming co-occur in 75% of cases (212, 213).
Antidepressants (Escitalopam, Venlafaxine) have been suggested for treating the most disturbing cases.
REM sleep behavior disorder: Missing or fragmented REM muscle atonia allows dream enactment
RBD has emerged out of the big bunch of night-time confusional states and violent activities, the latter reported by 1.7% of the population (214). Firstly described in 1986 (215), Schenck et al. characterized it as a manifestation where the patient “enacts” his/her dreams due to lack of muscle atony during REM sleep (RSWA - REM sleep without atonia).
The diagnostic criteria of RBD are the followings: (1) Repeated episodes of sleep-related vocalization and/or complex motor behaviors. (2) These behaviors are documented by polysomnography to occur during REM sleep or, based on clinical history of dream enactment, are presumed to occur during REM sleep. (3) Polysomnographic recording demonstrates RSWA. (4) The disturbance is not better explained by another sleep or mental disorder, medication or substance abuse (216).
In recent years it has been demonstrated that RBD affects sleep in all aspects: sleep instability in NREM sleep, measured with CAP metrics, is significantly reduced compared to age-matched healthy controls. This information can also provide some prognostic knowledge as a CAP rate < 32.9% signalizes a higher risk and a shorter latency for conversion into a syncleinopathy (217).
RBD is categorized as “idiopathic”, or rather “isolated” when standing alone; and symptomatic or rather “combined” when associated with other neurological disorders. Those combined forms may be iatrogenic related to drug use; may associate to neurodegenerative diseases especially α-synucleinopathies, as well as to tauopathies, TDP (TAR DNA binding protein) 43-pathies; to narcolepsy and to any dysfunction of the REM sleep network (218).
The prevalence of isolated RBD is ~0.38–2% in the population >60 years-old (219) and 5–13% in older community-dwellers doubly affecting men (219). A 1:1 gender ratio has been reported in younger groups (220). There is a high rate of autoimmune comorbidity in women affected (221).
RBD clinical features
Sudden, vehement and fragmentary movements, shouts out of sleep are key features of RBD. Injuries are frequent; caused by the patient falling out of bed or the bed partner being attacked by the half-sleeping individual enacting horrifying dreams that are remembered after (222). RBD episodes favor the second half of the night - the period of REM sleep dominance; in contrast to NREM parasomnia episodes emerging in the early hours of night sleep.
Those affected, need to be informed about the risk of injuries. Melatonin 3–12 mg or clonazepam 0.5–2.0 mg may help. Donepezil and Vortioxetin have been recently proposed as additional treatment options (223, 224). Concomitant aggravating factors must be corrected (see below: RBD and antidepressants).
RBD etiologies
Any etiology impairing the complex REM atonia network may cause RSWA/RBD. The duration (transitory or chronic) and associated features depend on the origin of the brainstem dysfunction (drug, hypoxia e.g., obstructive sleep apnea) or lesion (neurodegenerative, inflammatory etc.).
RBD and neurodegenerative conditions
There is a striking specificity of RBD converting to a synucleinopathy as Parkinson's disease (PD), diffuse Lewy body disease (DLB) or multisystemic atrophy (MSA), while the disorder links to tauopathies and TDP 43-pathies as Alzheimer's disease (AD), amyotrophic lateral sclerosis (ALS) and Huntington's disease (HD), Guadalopean Parkinsonism and progressive supranuclear palsy (PSP) as well (219–221).
RBD may precede any overt signs of these neurodegenerative disorders by several years/decades. Up to 90.9% of patients with isolated RBD convert to DLB, PD or MSA. RBD might be part of the insidious neurodegenerative process, caused by the degeneration and Lewy body deposition instructures regulating REM atonia (223). The neurodegeneration follows the Braak staging: a gradual “rise” of pathological damage from brainstem areas to more rostral regions.
Patients with “isolated RBD” showing subtle abnormalities like hyposmia, important constipation, orthostatic hypotension, and/or neuroimaging features suggestive of neurodegeneration, are likely to have prodromal synucleinopathies.
RBD-related brain changes were detected with structural magnetic resonance imaging (MRI) and diffusion tensor imaging (DTI): microstructural changes in the white matter of the brainstem, the right substantia nigra, the olfactory region, the left temporal lobe, the fornix, the internal capsule, the corona radiata, and the right visual stream (224). The progression of RBD linked with parieto-occipital and orbitofrontal thinning as well as visuospatial loss, while the cognitive decline associated with parietal degeneration (225).
Isolated RBD patients' decreased striatal DAT binding (226), the loss of nigral hyperintensity on 3.0-T MRI and transcranial echo may predict short-term progress of RBD to synucleinopathy (227, 228).
Multimodal MRI, neuro-melanin-sensitive volume-, and signal intensity measures as well as fractional anisotropy discriminate RBD patients from controls and predict a Parkinsonian progress (229).
RBD caused by autoimmunity: Anti-IgLON5 disease
Growing number of neurologic diseases have been recognized to have unexpected inflammatory or autoimmune etiologies e.g., PD and narcolepsy (230–232). A human leucocyte antigen (HLA) association is usually considered a hint to autoimmunity e.g., in narcolepsy, which is another REM sleep dysregulation syndrome sometimes overlapping with RBD and carrying the strongest HLA Class II association among all diseases. Also, RBD links with HLA class II genes: 84% of 25 RBD patients carried the DQw1 (DQB1*05,06) alleles and 28% were DR2 positive (233).
The recognition of a novel autoimmune-neurodegenerative disease-spectrum, the tauopathy anti-IgLON5 disease, indicates that an autoimmune etiology can cause RBD. Anti-IgLON5 disease is made by combinations of parasomnias, OSA syndrome, stridor, bulbar and limb movement disorders, axonal neuropathy and cognitive loss (234–241).
RBD and PTSD
There is a peculiar combination of RBD with PTSD (242–245). A motor dysfunction with increased muscle twitches during REM sleep has been early noticed in relation to stress and PTSD, and a higher rate of RBD in PTSD patients compared to controls, even to trauma-survivors without PTSD, generating a distinct term - trauma-associated sleep disorder (TASD) (244), sharing the features of the two conditions.
In PTSD, the rate of stress-related norepinephrine turn-over of the LC might change, leading to norepinephrine depletion and even cell deaths shown by neuroimaging in humans. In rats, a single acute stressor could precipitate long-lasting changes in LC function. Due to the persistent decrease of LC noradrenergic output to the REM atonia network, RSWA and RBD may evolve (246, 247).
RBD in PTSD might be a good example of a psychological effect turning to organic (248–251).
Antidepressants cause RSWA without RBD?
The association of RBD/RSWA with the use of selective serotonin reuptake inhibitors (SSRI) and selective norepinephrine reuptake inhibitors (SNRI) has been early described. Lately, several studies have shown an association of SSRI- and SNRI-use with RSWA only, curiously not accompanied by RBD (252–257). Patient manifesting episodes suspected for RBD, should discontinue all potentially related drugs, especially if taken at bedtime.
RBD treatment
In line with the concepts described above, the approach to RBD treatment should initially focus on the removal of any reversible factor, as serotoninergic antidepressants, bupropion, alcohol and sleep-fragmenting conditions.
Even though most RBD treatment data were obtained by retrospective studies, case series, or small clinical trials with no high-level evidence, in clinical experience pharmacotherapy is widely used and it has changed little over the decade. Indeed, high dose melatonin (from 2 to 18 mg nightly) and clonazepam (from 0.5 to 1 mg nightly) may be effective, with the former better tolerated especially by the elderly (258).
Sleep related painful erection
Sleep related painful erection is a rare parasomnia, occurring in 1% of men presenting with sexual problems.
It differs from the normal REM-related penile tumescence by the associated pain awakening the individual from sleep; who may have normal sexual life when awake. Local origins, a vagal dysfunction and central, especially antero-lateral hypothalamic etiologies have been raised. Baclofen has been found to be a good treatment option (259). Beta blockers, benzodiazepines and antidepressants were transitorily effective in some cases, and several additional treatments have also been used (260–262).
Future directions
REM parasomnias make an informative group of sleep disorders; most of them are consistent with REM dissociation phenomena. Neurodegenerative and autoimmune/inflammatory conditions or stroke; brainstem compression syndromes as well as antidepressants or hypoxia may transitorily or permanently compromise the REM atonia network functioning. The different RBD syndromes may manifest specific symptom-patterns (distribution of the lack of muscle atonia) offering a future discrimination tool.
RBD, as an early sign of synucleinopathy, may carry a prognostic value providing the future opportunity of preventing it. NREM sleep features, especially CAP analysis, can help to improve the characterization of disease prognosis.
The special link of RBD with autoimmunity seems more than pure chance, given the important and shared HLA association of RBD and anti-IgLON5 disease, an autoimmune tauopathy. The overlap with narcolepsy, another REM sleep-related and autoimmune condition with HLA Class II association and REM sleep disturbance, needs further scrutiny.
The association of RBD with PTSD, forming together the new entity trauma-related sleep disorder, provides an example of a psychology impact turning to brain-organic.
Further scrutiny of REM parasomnias may provide data for understanding the role of antidepressants in sleep regulation; the link of depression (characterized with short REM latency) other REM disorders, and finally, help clarifying the functions of REM sleep.
Circadian sleep-wake disorders
Humans are daily beings with inner rhythmicity bounded to the earth's day-night cycle. Circadian sleep-wake disorders (CRSWD) are caused by misalignment between internal sleep-wake rhythms and external synchronizers including, above all, the light-dark cycle. Circadian rhythm is physiologically supported by endogenously generated oscillations over a near-24-h period and continuously modulated by environmental cues and genetic predisposition (263). Individual rhythms are far from static conditions dynamically changing during the lifespan, due to hormonal changes and lifestyle habits, with a tendency for a first delay phase during adolescence followed by progressive shift into morningness in the adult age and among the elderly. The influence of parental control on sleep habits is assumed to be stronger during the early childhood, sometimes overshadowing the genetic predisposition (264).
Chronotypes variability
Multiple internal and external factors influence individual chronotypes including genetic and epigenetic features, season of birth, photo-exposition during early perinatal period (265), ambient temperature, physical activity, food intake (266) and many others.
In the latest years population-based studies identified a progressive shortening of total sleep time and a circadian preference for eveningness in the adults and elderly, with even more evident differences among the youngest (267, 268), probably reflecting the impact of complex factors including the massive use of electronic devices, shifting in television programs schedule and job's related duties (268).
The clinical spectrum of CRSWDs
The spectrum of CRSWDs include both “intrinsic” and “extrinsic” conditions. The firmer group is composed by advanced sleep-wake phase disorder (ASWPD), delayed sleep-wake phase disorder (DSWPD), non-24-h sleep-wake rhythm disorder (N24SWD), and irregular sleep-wake rhythm disorder (ISWRD). The so-called “extrinsic” disorders (or environmentally related) involved the shift work, jet lag disorder and other acquired “socially-related” irregularities of the circadian rhythm (269).
Light therapy may be used, according to individuals' phase response curves, to delay or to advance circadian timing. Typically evening light exposure, used before the core body temperature minimum (CBT-min), favors phase delays, whilst light therapy in the morning, after the CBT-min, leads to phase advances.
According to the task-force results, there is no evidence to support the use of strategic avoidance of light in patients with any CRSWD, while light exposure may be adopted only for patients with ASWPD and among patients with dementia and ISWRD (“weak for” recommendation).
Sleep promoting medications should always be avoided in patients with CRSWD, especially among patients suffering for dementia (“strong against” recommendation).
Timed oral melatonin administration could be adopted in patients with DSWPD with or without psychiatric comorbidities; N24SWD or children/adolescent with ISWRD (“weak for” recommendation), however the same strategy should be avoided in elderly with dementia and ISWRD (“weak against” recommendation).
Wakefulness promoting medications and/or somatic interventions should be avoided and combined therapy (light therapy + multicomponent behavioral interventions) could be used for children/adolescents and DSWRD (“weak for” recommendation).
Notably, the summarized practice guidelines rely on few and heterogeneous published researches on CSWRD and, so far, the scenario still appears blurred due to insufficient/absent data.
Knowledges pitfalls in CSWRD had nicely been analyzed in an international workshop (270). Among the major gaps in the field there is the absolute lack of individualized evaluation. Even if few sleep centers use the salivary dim light melatonin onset (DLMO) as indicator of circadian phase, currently no standardized protocol exist and CSWRD diagnosis is based on self-reported sleep habits and schedule. No exam or biological biomarker is required to measure circadian timing in patients with suspected CRSWD, to tailor disease strategy on patient's characteristics.
Further researches are urgently needed to investigate diseases phenotypes and their evolution with aging.
Smart working and sleep disturbances
In the latest months, as direct social consequence of the COVID-19 pandemia, a specific kind of work schedule organization impressively increased: the smart working, whose long-lasting impact on wake-sleep rhythm is to be defined. Intuitively patients suffering from pre-existent intrinsic circadian sleep disorders could have gain few benefits from this new situation, due to higher flexibility on their schedules, bedtimes schedule and spare time organization. However, the reduct exposition to external synchronizers (light, activities, sports) and the increase exposure to artificial light and electronic devices, frequently until late in the night, may have favored misalignment of individuals circadian rhythm. Data collected from the International Labor Organization (ILO) pointed out some other negative effects of smart working, including a tendency to work longer and an overlap between paid work and personal life, which can lead to high levels of stress (271). We assume that some gender-related differences may exist, being women, especially if with kids, more prone to overwhelming duties and with a tendency for multi-tasking activities.
To what extent the forced novel scenario of “smart-work” have influenced people with intrinsic CSWRDs and/or whether it has promoted novel categories of extrinsic circadian sleep-wake diseases need to be investigated.
Shift working
Another category of workers overexposed to circadian irregularity/misalignment are those practicing shift work, a condition associated with a recurring work schedule that overlaps the usual time for sleep (24). The full spectrum of shift work comprises regular evening or night schedules, early morning shift work, evening/afternoon shift work, rotating shifts, split shifts, on-call or casual shifts, 24-h shifts, irregular schedules, and other non-day schedules. Impact on sleep quality and amount could be different according to shift types, with morning shifts frequently associated to REM sleep loss and severe sleep inertia (272); night shifts usually associated with greater curtailment of total sleep time and rotating shift schedules facing additional challenges related to the speed and direction of shift rotations (273). Shift workers are at higher risk for car crashes (274), behavioral changes (275), metabolic diseases (276, 277), cardiovascular diseases (278, 279), hypertension (280, 281), infertility (282) and tumors. Abnormalities in clock genes expression had been associated to miscarriage and infertility in women, impacting hypothalamic regulation (283), with still inconclusive data regarding fertility impact in male.
Genetic differences are assumed to be involved in terms of vulnerability toward sleep deprivation (284). Resistant subjects tend to respond more efficiently to unanticipated sleep–wake states (285). Factors associated to shift work tolerance include young age, eveningness, extraversion and male sex (286).
The circadian rhyhtm scenario in the youngest and the oldest
The younger face also another form of external circadian stress, which appears strongly related to social demands. As a consequence many subjects find themselves living a novel form of jet-lag: the “social jet-lag”, due to continuous changing in sleep-wake habits from week and weekend, frequently leading to coping strategies such as soft drinks, caffeine and alcohol consumption or abuse (287). On the opposite side the modern society needs to tackle with the progressive demographic aging of the population, ending in the continuous increase of the number of subjects with major frailty toward circadian rhythms disruption, partly due to the aging-dependent functional decline in light sensitivity and sedentary lifestyle (288). Sleep among older individuals is characterized by reduced percentage of SWS, increase of light sleep stages and multiple nocturnal awakenings (289). Animals studies suggests that several clock genes expression is reduced with aging (290). The scenario is even more severe among patients with neurodegenerative disorders such as Alzheimer's disease (AD), where circadian misalignment is more extreme due to mechanisms related to the neurodegeneration process itself (loss of the circadian “pace-maker” vasoactive intestinal peptide-expressing neurons in the CNS, direct effects of amyloid on clock genes expressions and other hypothesized factors) (291), to virtually absent exposition to external synchronizers and to manifold medication side effects (292). Circadian sleep disruption can be revealed since the earlier prodromal phase of Alzheimer's disease, preceding cognitive manifestations and, in untreated, may faster the progression of neurodegeneration (293). Same derailment in sleep-wake regularity concurrently involved AD patients' caregivers, whose schedules are driven by patients' needs (294) and whose caring capability could be strongly hampered by circadian misalignment.
Health consequences of circadian rhyhtm disruption
Sleep-wake disruption may lead to increase risk for adverse mental health outcomes, with stronger impact on emotional control among women, exposed to higher risk for depressive symptoms (295) and higher risk for maladaptative behaviors, including suicidal ideation, among men (296). The relationship between circadian rhythm and mental health is complex and bidirectional, as supported by consolidated evidences confirming the role of chronotherapy as powerful treatment for unipolar and bipolar depression (297). Besides the known consequences of circadian rhythm irregularity on mood stability and cognitive functions, there are also some others regarding metabolic balance (298). Adolescent with social jet-lag are at higher risk for obesity, with female more exposed with respect to young male (299). Circadian misalignment significantly decreases the levels of circulating anorexiant hormone leptin, with an in-parallel increase of the appetite-stimulating ghrelin (300). Clocks genes are involved into cell proliferation and oncoregulation (301) and melatonin itself act as antioxydative and onco-suppressant agent and converging evidences highlighted the existence of stronger risk for certain types of tumors (breast, thyroid, lung, prostate, pancreas tumors and others) (302) in subjects experiencing circadian disruption (303). Factors involve including melatonin and estrogen fluctuations, chronic inflammation, autoimmune dysfunction, metabolic impairment and DNA oxydative damage (302). Chronodysruption is recognized as an independent pro-oncogenic risk factor (Level of evidence IARC (International Agency for Research on Cancer) (2A), with risks increase with duration of exposition to work-shifting (302), being higher after 20 years.
Future directions
In summary circadian imbalance needs to be handled in a comprehensive view, as many factors can be involved in its modulation/dynamics (304). A more individualized approach for intrinsic CSWRDs is desirable. Biomarkers for a better definition of circadian sleep-wake cycles are advisable. There is still lack of knowledge on the relationship between CAP and CSWRDs. It might be interesting to verify the utility of CAP metrics in the follow-up of CSWRDs diagnosis and follow-up.
Finally, in a more “olistic” perspective, it is probably more correct to refer to a “circadian syndrome” rather than “circadian sleep disorder”. Long-term prospective data on health consequences of smart working are advisable.
Brain imaging and sleep disorders
Advance in brain imaging
Brain imaging technologies gained impressive progress in the latest years, permitting some attractive breakthroughs in both structural and functional central nervous system analysis.
The availability of advanced computer-aided tolls to support qualitative evaluation including voxel and surfaced-based morphometry, DTI white matter reconstruction, shape analysis and others, lead to outstanding growth of knowledges about brain tissue characteristics, dynamics and functioning. Modern post-processing methods permit to explore brain anatomy beyond the resolution of naked-eye image observation, allowing to analyze MRI frames with ultra fine resolution, exploring neural connectivity profile and structural-functional coupling (305, 306).
The apparent paradox of using “structural” data to analyze connectivity profiles had already been tackled in multiple research fields, including above all epilepsy and psychiatry (307, 308). Structural networks could be inferred from diffusion MRI tractography or inter-regional covariance patterns of structural measures such as cortical thickness or gyration, extending the significance of morphometric studies to functional rationale. In these frameworks the identification of high morphological correlation between distinct regions (“covariance”) can be interpreted as indirect sign of networks union (309). Besides automated and semi-automated advanced structural MRI processing, variable functional methods including static and dynamic fMRI (functional-MRI), task-related or task-free investigations are also widely used.
Brain imaging in sleep disorders
So far, the utilization of advanced structural or functional brain imaging for sleep disorders is still limited to research purposes, with no application in everyday practice. Indeed, most of described post-processing techniques are strongly demanding and time-consuming, requiring dedicated training formation. Besides sleep disorders diagnosis rarely even require the execution of brain imaging of any sorts, with some minor exceptions including among others sleep-related epilepsies, RBD or narcolepsy. And, even in these cases, brain MRI results are not taken into account in the disease's diagnostic criteria, being useful instead to look for or to rule out the existence of underlying structural lesions and current sleep disorders classification is based only on clinical and/or polysomnographic criteria. We argued if imaging informations could support a more tailored approach toward sleep pathology and sub-classification.
Nowadays one of the major drawbacks of imaging studies in the sleep disorders field is the lack of standardized imaging protocol for acquisition or processing of brain images. Important differences in studies' results may thus reflect methodologies and acquisition differences, hampering results' comparisons and depleting conclusive considerations (310). Moreover, the absence of normative data from healthy sleepers and the small samples of most of available studies affect the interpretation of results.
The creation of open-source big dataset/atlas from healthy sleepers with univocal imaging protocol for acquisition is recommended and could support the continuous increase of expertise in this fascinating field (311).
The sleeping brain organization dynamically changes at variable levels across lifespan, as demonstrated both with PSG recording and at clinical level. At the same time brain structure itself dynamically evolves with aging, following non-stochastic trajectories and respecting hierarchical dynamics (312, 313) according to neurodevelopmental growth. Given the relevance of sleep for brain functioning, memory performance and learning, the measurement of its impact on anatomic and functional brain dynamics is not a trivial issue.
Some common sleep disorders could act as facilitators for premature brain aging (e.g., insomnia, or sleep breathing disorders), leading to potentially severe cognitive consequences (314, 315). This is not surprising due to the demonstrated role of sleep in terms of body and mind restoration, toxins elimination (such as Ab and tau) (316, 317) synaptic strengthening and pruning, endocrine balance and autonomic correct functioning.
Sleep duration influences the amount of cortical deposition of Ab in healthy sleepers (318). Thalamus and hippocampus concentration of Ab increases after only one night of acute sleep deprivation (319) and cerebrospinal fluid levels of Ab42 are significantly increased in chronically insomniac patients (319). Morphometric studies showed that chronic insomniac patients present lower brain volumes in several cortical and subcortical brain areas including medial frontal and middle temporal gyrus, middle cingulate cortex, basal ganglia and hippocampus as well as gray matter increase in the anterior cingulate cortex (320), with changes correlated with disease severity. Insomnia may thus exacerbate the neuropathological processes involved into cognitive impairment (321). Given the wide heterogeneity of insomnia subtypes we argued if the utilization of imaging informations could provide some novel insights into disease mechanism, helping in stratifying patients' severity according to the extent of brain damage. Unfortunately, the lack of standardized MRI protocols and the small sample sizes of most studies exploring insomnia morphometric data account for several inconsistencies across studies' results (322).
Imaging insight into sleep disorders
RLS and PLMD are the commonest sleep-related movement disorders, frequently overlapping in the same patient. Whilst RLS pathogenesis is assumed to derive from a dysfunction of the dopaminergic hypothalamo-spinal inhibitory pathways and a frequent relationship with reduced brain iron content (323) and spinal hyperexcitability is a consolidated issue (324), pathogenesis of PLMD is still largely unknown. Imaging studies in RLS patients revealed the presence of decreased iron content in the substantia nigra, but not in other subcortical nuclei (caudate head, putamen, globus pallidus, red nucleus) among late-onset RLS (325). Conflicting results were found in similar studies covering the topic (326, 327), probably once again reflecting inconsistencies in patients' selection (on medication/drug free; age of participants; disease duration), MRI scan strength (1.5 Tesla vs. higher field machines) or methodologies (manual tracing ROI, automated ROI identification and others).
Neuroimaging investigations exploring isolated PLMD neurobiology are lacking. Higher score on PLM index has been associated with increased risk for white matter hyperintensity burden and thus has been proposed as a neuroradiological biomarker for increased risk of cerebrovascular events (67, 328). Data from HRV analysis revealed the association of PLMD with reduced parasympathetic tone, increased sympathetic tone (49), supporting its role as an acquired (and manageable?) risk for cardiovascular events. Secondary forms of PLMD has been described in association with pontine stroke (329, 330), supratentorial stroke (331) and parietal hemorrage (332), leading to the hypothesis that the loss of cortical or subcortical inhibition on brainstem movement generators (e.g., reticular formation) might cause PLMD. Further imaging studies are advisable to enhance our knowledge on both PLMD pathogenesis and its impact on cardiovascular risk.
Furthermore, given the lifespan dynamics of PLM index itself, dedicated investigations should be performed to ease the discrimination of more “disturbing” phenotypes requiring treatment, from those reflecting the para-physiological aging of neuro-spinal networks.
Brain imaging is currently not recommended for SHE diagnosis. However, numerous SHE patients, according to the International League Against Epilepsy (ILAE) diagnostic criteria (333), can be described as affected by focal epilepsy with a structural etiology, as malformations of cortical development (MCDs) and low-grade associated tumors (LEATs) are among the commonest radiological findings. Even if traditionally associated with frontal-lobe onset, due to its strong association with abrupt hyperkinetic automatisms and complex behaviors, it has been widely confirmed that the seizure onset zone in SHE patients may also be extra-frontal (334). The subsequent spreading of epileptiform discharges to the anterior cingulate and frontal areas, irrespectively of its origin, explain the well-known semiological similarities between patients with distinct seizure onset zone (SOZs). Reduced N-Acetyl-aspartate/Creatine (NAA-Cho) ratio had been identified in the anterior-cingulate cortex of SHE patient using proton magnetic resonance spectroscopy (335). Notably the same NAA-Cho reduced ratio had been associated with neuronal loss, abnormal metabolism and gliosis in temporal lobe epilepsy patients (336). At functional level SHE patients present higher functional connectivity between thalamus and sensori-motor cortices, areas directly involved in the K-complex dynamics and arousal pathway. The intrusive role of arousal in the SHE pathogenesis is a well-known issue. Brain imaging results may support a deeper understanding of mechanisms linking sleep-instability, arousals and SHE-related epileptiform networks in a comprehensive framework.
Another challenging scenario for the sleep clinician is the one of hypersomnolence disorders e.g., narcolepsy type 2 or idiopathic hypersomnia (IH); both conditions' diagnosis being often tricky. Even if underlying medical conditions should always be ruled out, no formal recommendations impose brain imaging as part of the diagnostic work up.
New-onset hypersomnia may be the first clinical hint for inflammatory brain lesion (337), vascular events (338) and numerous other conditions. Previous works highlighted the presence of subtle structural abnormalities at MRI visual inspection among around 1/3 of patients suffering from idiopathic hypersomnia and studied with conventional brain MRI, but with significant clinical impact on patient management just in a minority of cases (339).
In the latest years growing attentions has been dedicated to the “task-free” connections within the brain. Accordingly spontaneous low frequency neural oscillations may be traced to recognize functionally linked (but anatomically separated) brain areas, referred as resting state networks (RSN) (340).
RSN-f-MRI studies identified further consequences at both structural and functional levels in most patients with IH including “thicker” cortex in pivotal nodes of one of the most important RSN, the default mode network (DMN), at the level of precuneus and medial prefrontal cortex, together with decreased functional activation of its anterior part (341). A strong covariance tendency/pattern within the entire DMN had also been described in patients with IH. Similar results were confirmed in functional SPECT studies (342), suggesting a network-wide level for the pathology. EDS has also been associated with regional A-beta amyloid deposition in the anterior cingulate, posterior cingulate-precuneus and parietal regions in an elderly cohort with no cognitive deficits (343), confirming the involvement of DMN nodes in hypersomnolence appearance. In acutely sleep deprived subjects sleepiness was associated with distinct anterior-to-posterior gradient of hypo-activation of the DMN (344) and in Narcolepsy type I sleepiness severity was linked to reduced functional connectivity measures in the left anterior cingulate gyrus and in the bilateral posterior central gyrus (345). The antero-medial part of the DMN is strongly connected with the ascending activating system and represents one the most important networks for alertness and self-consciousness (346). Aberrant connections between DMN and other resting state netwroks such as the salience network, has been described among insomniac patients (347). Hopefully advanced investigations on DMN subdivisions and connections could provide a deeper understanding of alertness and sleepiness mechanisms and of sleep pathologies.
Brain organization dynamics during NREM sleep stages had also been recently explored (348), with some interesting novelties regarding networks' interactions evolution with respect to sleep depth. According to this study interdependencies and interactions between networks progressively increase during the “unstable” stage N2 and subsequently break down with the appearance of the more “stable” stage N3.
Multimodal EEG-fMRI investigations may also help in answering to some fascinating open-questions such as reasons explaining inter-individual differences in dream recall frequency and capacity (349). Enhanced connectivity profile within the DMN and regions involved in memory processing seems to be important to promote short term memory recall during sleep-wake transitions.
In this direction advanced neuroimaging studies could empower our understanding of basic neurophysiological mechanisms embedded into sleeping brain functioning.
The forgotten sleeping cerebellum
Besides cortical and subcortical regions, even more mysterious is the involvement of other relevant brain structures in sleep texture organization and sleep disorders, such as, above all, the cerebellum. Regardless to the central role of sleep in consolidating procedural learning and the major significance of the cerebellum in the development of motor performances surprisingly, so far, only few efforts have been dedicated to understand the dynamics of cerebellum during sleep. Changing the firing patterns during NREM and REM sleep in cerebellum cells is well-documented (350, 351). The cerebellum also express various genes involved in the circadian rhythm organization (352). Sleep spindles are associated to BOLD signal in the cerebellum in fMRI studies (353) and bidirectional influences in neural firing of thalamo-cortical output and cerebellar cortex have been documented in animals (354). Variations in cerebellum morphometry and volume have been identified in numerous sleep disorders including insomnia, OSA or hypersomnolence disorders (355). Cerebellum appears implicated also in RBD pathogenesis with controversial morphometric results (356, 357).
Given the technical difficulties in examining cerebellum EEG activity with surface recording (the near-by occipital visual areas lead to several recording artifacts), probably more reliable informations could be collect through advanced brain imaging analysis (358). Further studies are advisable to allow for a deeper understanding of the complex relationship between sleep, sleep disorders and the cerebellum.
Future directions
The proper characterization of sleep diseases, referring only to disease's clinical features may be challenging and, sometimes, incomplete. The creation of big dataset from healthy sleepers of variable ages and the definition of shared images protocol for acquisition are mandatory to guarantee progress in this fascinating field of research.
Information collected from brain imaging may provide support for disease's diagnosis, enhance understanding regarding disease's neurobiology and lead to extremely sensitive follow-up with respect to disease's evolution and consequences.
Dedicated education for advanced brain imaging management should therefore be proposed to sleep clinicians and, equally important, adequate training regarding sleep disorders and their impact in brain morphometry and imaging should involve neuro-radiologists.
Sleep recording methodology
The overwhelming richness of biological information encoded in sleep state data is largely minimized by conventional approaches of assessment. This “simplification”, though having some utility in the realms of pragmatism and convenience, has severely impaired the cognitive landscape of thinking and using sleep data. Advances in visual and computational analyses now offer opportunities to improve disease characterization and endotype/phenotype characterization, applicable to a range of sleep disorders.
Methods of characterizing sleep
The scoring manual of the AASM accessible through the Internet (The AASM Manual for the Scoring of Sleep and Associated Events. http://www.aasmnet.org/scoringmanual/default.aspx) provides standard but simplistic scoring guidelines generating sleep stage, heart rate, breathing, and motor activation metrics. However, besides the standard stages, alternate methods of characterizing sleep include mature technologies such as high resolution spectral analyses, a continuous sleep depth measure called the Odds Ratio Product (ORP) (359, 360) and variants thereof, CAP of NREM sleep (361) and CPC (high, low and very low frequency coupling of autonomic and respiratory drives, modulated by cortical delta power) (362). NREM stage N3 is usually associated with stable breathing. However, such periods frequently occur outside N3, during a stable form of N2, when the EEG usually shows a non-CAP morphology. Arousals are traditionally scored as an all or none event but show a wide range of perturbation and recovery kinetics, and individual differences which may amplify or dampen the progression of sleep state or respiratory events (363–367). A number of computational methods are available to identify high loop gain of the respiratory control system, which is of key therapeutic importance.
High quality EEG spectral analysis
Spectral analysis of the sleep EEG has a long history of use in sleep research but not in clinical practice. Part of the reason is the “fuzziness” of the graphs generated by conventional polysomnographic software, making reading/interpretation difficult. Multitaper is a spectrum estimation method developed to provide an optimal tradeoff between time and frequency resolutions using taper functions, which are called Slepian sequences. Each taper provides a different emphasis of the signal. Each tapered signal undergoes Fourier transform, which converts signal (time domain) into a spectrum (frequency domain). The multitaper spectrum estimation means to average these spectra, which leads to an unbiased and less noisy spectrum. The strength of the multitaper spectrum estimation is due to the fact that the taper functions are orthogonal to all others. Therefore, each tapered signal is focused on specific spectral aspects, and after averaging it combines these different aspects while not canceling each other (368).
CPC maps sleep stability domains
The SleepImage® System (369) performs CPC analysis and can be run on any signal recordings that include an ECG or similar information-content signal including pulse plethysmography, including full or limited polysomnography. The system enables ambulatory assessment of sleep quality, actigraphy, heart rate measures, and sleep apnea (23). The current embodiment of the technology uses Ring Oximeter coupled to a Smartphone Application linked to a Cloud Computing system. There are no disposable elements, and the Ring enables easy repeated testing. The Ring can record for 16 h on a single charge. The raw pulse photoplethysmogram can also be analyzed for arousal events.
CPC analysis may be performed on any signal which has heart rate and respiratory tidal volume information, including the electrocardiogram and the pulse photoplethysmogram (PPG) (370, 371). Two distinct patterns of CPC are observed: high frequency coupling (HFC; 0.1–0.4 Hz) and low frequency coupling (LFC; 0.01 to < 0.1 Hz). HFC is associated with stable breathing during NREM sleep. HFC aligns with critical biological structures of sleep, including delta power, stable breathing, blood pressure dipping, and an unfragmented < 1 Hz slow oscillation (23, 372). A preponderance of a subset of LFC, named elevated-low frequency coupling (e-LFC), detects apneas and hypopneas (373).
By detection and quantifying self-similar sinusoidal oscillations of HRV typically seen in periodic breathing/Cheyne-Stokes narrow spectral band e-LFC (e-LFCNB, putative central sleep apnea, periodic breathing, or complex sleep apnea) is determined (374). A third CPC pattern named very low frequency band (v-LCF; 0 to < 0.01 Hz) has two subsets, wake and REM sleep. When classifying REM vs. wake, spectral dominance in the upper range of the v-LFC band in addition to lack of motion or motion artifact, is indicative of REM sleep. Total sleep time (TST) and sleep efficiency are then determined as follows: (i) Establish actigraphic sleep boundaries. (ii) mark the sleep onset as the first occurrence of HFC or LFC, (iii) mark the sleep conclusion as the last occurrence of HFC or LFC, or the last occurrence of REM, and (iv) classify the windows of v-LFC dominance into either REM or wake.
Assessment of CPC parameters and the biological correlates have been performed in numerous conditions, including sleep apnea pre/post treatment (23, 375), insomnia (376), depression (377), fibromyalgia (378), stroke (379), atrial fibrillation recurrence risk (380), glucose handling (381), and blood pressure regulation (382). The technology is valid in children as well (383, 384). A FDA-approved polysomnogram-equivalent apnea-hypopnea index is now available (368).
Sleep state modifies the expression of sleep apnea
Airflow patterns and arousal thresholds are modulated by sleep macrostructure (REM and NREM sleep, stages of NREM sleep), sleep microstructure (CAP and non-CAP), homeostatic sleep drive, medication, and age. Data suggest that NREM sleep is bimodal rather than graded, with stable and unstable regime, or alternatively conceptualized as effective and ineffective. Thus, rather than thinking in terms of grades of NREM sleep (N1–N3) of progressive depth, the stability domain has only 2 forms of NREM sleep—stable and unstable. N3 is usually stable, N1 is always unstable, but N2 may be stable or unstable. These periods of stability intrinsic to NREM sleep can determine the instantaneous presence or absence of sleep apnea. Cardiopulmonary analysis shows that stable breathing periods result in high frequency coupling of respiration and HRV, simultaneously associated with high EEG delta power. Such periods are not restricted to N3 but make up most of N2 in health. Intermittent periods of stable breathing are well-recognized in patients with even severe obstructive sleep apnea, during both N3 and N2 (385). Flow-limitation can be prominent, and both hypoxia and hypoventilation may occur during these obstructed but stable periods with prolongation of inspiratory time with minimal breath-to-breath variability of tidal volume. These periods do have an impact on the EEG, but visual determination is not possible, and computational methods such as the respiratory cycle-related EEG change are required (386, 387). The persistence of CAP-type features on the EEG may also occur during SWS associated with severe persistent flow-limitation, suggesting the presence of concomitant disruptive influences from hypoxia, hypercapnia, excessive respiratory effort, and upper airway stimulation.
Excessively unstable NREM sleep disproportionate to disruptive pathology on the PSG may be recognized by a sleep fragmentation phenotype. This phenotype can be suggested by prolonged sleep-wake transitional instability (>10 min), low sleep efficiency (< 70%), persistently high N1 stage during positive airway pressure (PAP) titration (>15%), and poor evolution of SWS (< 1 Hz) (363). In contrast, even severe sleep apnea may coexist with relatively well-preserved architecture. Chin EMG tone elevations during NREM sleep are not part of the conventional arousal definition, but the duration and degree of elevation vary markedly between individuals and stays consistent within individuals. The same concept can be applied to the EEG (arousal duration or return to sleep) and heart rate responses to arousal—inter-individual variability is contrasted with intra-individual stability (388).
Sleep is a continuous flow, which can be perturbed and distorted
The continuity of sleep during individual sleep cycles across a typical night requires networking, cohesion, and reciprocal interactions of NREM and REM sleep processes. Intuitively, stable periods of NREM sleep are deeper, but both stable and unstable NREM sleep can have varying degrees of depth. Conventional stages do not effectively capture sleep depth, even if in most instances N3 is deeper and more stable than N2.
One established method of assessing continuous sleep depth is the Odds Ratio Product (389). ORP is calculated every 3 s from the power spectrum of the EEG. It represents the EEG powers in four different frequency bands relative to each other, with higher values representing increased propensity for an arousal. It has an excellent correlation (r2 = 0.98) with arousability, defined as the probability of an arousal or awakening occurring within 30 s. It also changes appropriately in response to sleep deprivation, sleep restriction, and brief acoustic stimuli, and increases across the night, as may be expected from the decrease in homeostatic sleep pressure. A related method using machine learning approaches provides a wider and intuitive range of numerical values, the Ordinal Sleep Depth, with profiles very similar to the ORP.
Sleep depth post-arousal varies substantially among subjects and patients with OSA from being very light to very deep. The ORP fluctuates after an arousal, rising to wake levels and then decreasing as sleep resumes and stabilizes. ORP-9 is the ORP 9 s after the start of the arousal (390). If the ORP remains high several seconds after the arousal, it suggests that the “glue” (network cohesion) of sleep is poor, and this high ORP-9 would indicate that the high arousability is much more likely to be due to abnormal central regulation of sleep depth. In patients with high ORP-9 (highly arousable soon after a previous arousal), arousal is likely to occur soon after the obstruction and interrupt the recruitment of pharyngeal dilators, leading to recurrence of events. Conversely, subjects in whom sleep becomes quite deep immediately after arousal (low ORP-9) can tolerate the obstruction for a longer time allowing the reflex recruitment of dilator muscles, thereby increasing the probability of resolving the obstruction without arousal. ORP-9 was found to be the main determinant of average sleep depth and to be invariably high in patients with very high AHI. Furthermore, ORP-9 is quite reproducible even when re-measured after 5 years, suggesting that it is a trait (Younes M; unpublished observations).
A vertically integrated approach to sleep data analysis
Every method assessing sleep, provides unique information on sleep architecture in health and disease. How does one reconcile these various methods? A new approach to sleep data is proposed. Rather than various methods being competitive or additive at best, it is proposed that by “stacking” and “vertical integration”, profound new insights may be obtained. The examples below show the strength of this “insight at a glance” approach (Figure 6).
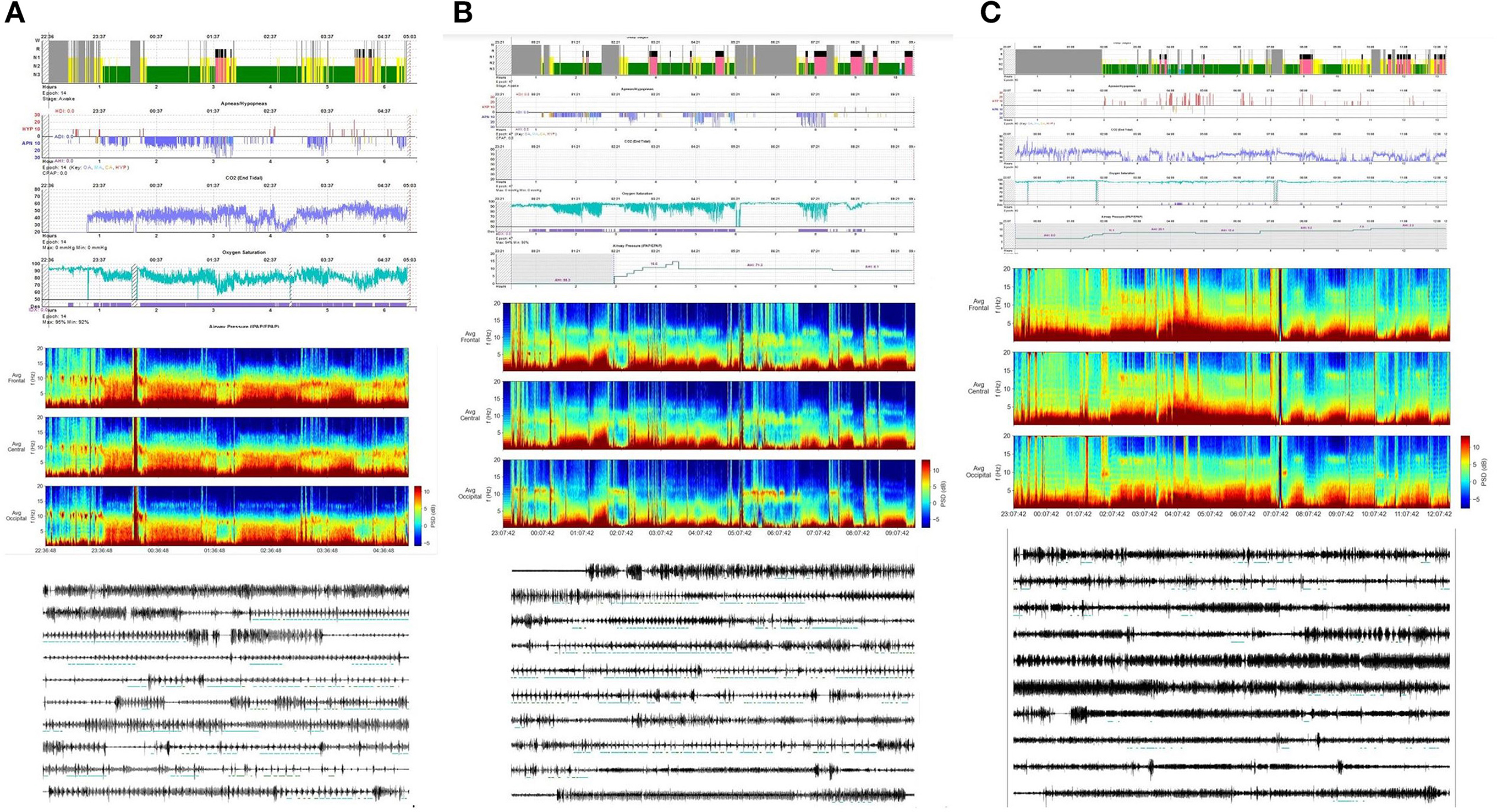
Figure 6. Three examples showing that value of vertical integration of high-quality EEG spectral analysis inclusive of respiration, oximetry, capnometry, and respiratory phenotype in OSA patients. (A) Patient with intrinsically high sleep quality, who develops severe sleep apnea and high loop gain, complicated by hypoventilation. Merely supporting the upper airway with continuous positive airway pressure will improve oxygenation and ventilation, but respiratory instability is likely to persist. Aggressive ventilation could amplify periodic breathing. In this case, a contribution from sleep fragmentation will be minor, given the high delta power despite the highly disruptive pathology. (B) Delta power profile is highly disrupted, and initial sleep apnea treatment is not effective. Once high loop gain is targeted (oxygen and dead space), sleep and sleep apnea markedly improves. (C) Sodium oxybate is administered (3 gm) just before lights out, but the patient remains awake for over 2 h. Once he falls asleep, the large increase in slow-wave power typical of oxybate sleep effects in not seen, but rather, sleep stage and sleep powers remain fragmented. Slow-wave power increases late, suggesting that there is substantial circadian phase delay. The drug does cause an increase in delta power while awake, before sleep onset. This fragmentation is contributed to by difficult to treat sleep apnea, and sleep fragility of uncertain cause. This patient has a diagnosis of hypersomnia in addition, being able to sleep 16+ h in a 24-h period. Even after the second dose of oxybate (3 grams), sleep remains fragmented. Stable breathing is relatively low, but there is no pervasive periodic breathing / high loop gain apnea as in the other two examples.
Author contributions
LP, CM, PH, AS, and RJT conceived of the presented idea and drafted the manuscript. NA, FR, SP, AZ, and FM contributed to the final version of the manuscript. All authors have seen the manuscript and approved it in its current version.
Conflict of interest
The authors declare that the research was conducted in the absence of any commercial or financial relationships that could be construed as a potential conflict of interest.
Publisher's note
All claims expressed in this article are solely those of the authors and do not necessarily represent those of their affiliated organizations, or those of the publisher, the editors and the reviewers. Any product that may be evaluated in this article, or claim that may be made by its manufacturer, is not guaranteed or endorsed by the publisher.
References
1. Shepard JW Jr, Buysse DJ, Chesson AL Jr, Dement WC, Goldberg R, Guilleminault C, et al. History of the development of sleep medicine in the United States. J Clin Sleep Med. (2005) 1:61–82. doi: 10.5664/jcsm.26298
2. Rios P, Cardoso R, Morra D, Nincic V, Goodarzi Z, Farah B, et al. Comparative effectiveness and safety of pharmacological and non-pharmacological interventions for insomnia: an overview of reviews. Syst Rev. (2019) 8:281. doi: 10.1186/s13643-019-1163-9
3. Pillai V, Cheng P, Kalmbach DA, Roehrs T, Roth T, Drake CL. Prevalence and predictors of prescription sleep aid use among individuals with DSM-5 insomnia: the role of hyperarousal. Sleep. (2016) 39:825–32. doi: 10.5665/sleep.5636
4. Winkelman JW. Clinical practice. Insomnia disorder. N Engl J Med. (2015) 373:1437–44. doi: 10.1056/NEJMcp1412740
5. Tricco AC, Zarin W, Lillie E, Pham B, Straus SE. Utility of social media and crowd-sourced data for pharmacovigilance: a scoping review protocol. BMJ Open. (2017) 7:e013474. doi: 10.1136/bmjopen-2016-013474
6. Riemann D, Baglioni C, Bassetti C, Bjorvatn B, Dolenc Groselj L, Ellis JG, et al. European guideline for the diagnosis and treatment of insomnia. J Sleep Res. (2017) 26:675–700. doi: 10.1111/jsr.12594
7. Terzano MG, Parrino L, Cirignotta F, Ferini-Strambi L, Gigli G, Rudelli G, et al. Studio Morfeo: insomnia in primary care, a survey conducted on the Italian population. Sleep Med. (2004) 5:67–75. doi: 10.1016/j.sleep.2003.09.006
8. Bjorvatn B, Meland E, Flo E, Mildestvedt T. High prevalence of insomnia and hypnotic use in patients visiting their general practitioner. Fam Pract. (2017) 34:20–4. doi: 10.1093/fampra/cmw107
9. Wittchen HU, Krause P, Höfler M, Pittrow D, Winter S, Spiegel B, et al. Screening-Studie zur Insomnie-Erkennung in Hausarztpraxen. Fehldiagnose bei jedem zweiten Patienten? [Screening study for detection insomnia in general practice. Diagnostic error in every second patient?]. MMW Fortschr Med. (2001) 143:43–4.
10. Marschall J, Hildebrandt S, Sydow H, Nolting HD. Gesundheitsreport (DAK Report). Heidelberg: Medhochzwei Verlag (2017).
11. Krystal AD, Prather AA, Ashbrook LH. The assessment and management of insomnia: an update. World Psychiatry. (2019) 18:337–52. doi: 10.1002/wps.20674
12. Cellini N, Conte F, De Rosa O, Giganti F, Malloggi S, Reyt M, et al. Changes in sleep timing and subjective sleep quality during the COVID-19 lockdown in Italy and Belgium: age, gender and working status as modulating factors. Sleep Med. (2021) 77:112–9. doi: 10.1016/j.sleep.2020.11.027
13. Kokou-Kpolou CK, Megalakaki O, Laimou D, Kousouri M. Insomnia during COVID-19 pandemic and lockdown: prevalence, severity, and associated risk factors in French population. Psychiatry Res. (2020) 290:113128. doi: 10.1016/j.psychres.2020.113128
14. Li Y, Qin Q, Sun Q, Sanford LD, Vgontzas AN, Tang X. Insomnia and psychological reactions during the COVID-19 outbreak in China. J Clin Sleep Med. (2020) 16:1417–8. doi: 10.5664/jcsm.8524
15. Paul F, Alpers GW, Reinhard I, Schredl M. Nightmares do result in psychophysiological arousal: a multimeasure ambulatory assessment study. Psychophysiology. (2019) 56:e13366. doi: 10.1111/psyp.13366
16. Qaseem A, Kansagara D, Forciea MA, Cooke M, Denberg TD, Clinical Guidelines Committee of the American College of Physicians. Management of chronic insomnia disorder in adults: a clinical practice guideline from the American College of Physicians. Ann Int Med. (2016) 165:125–33. doi: 10.7326/M15-2175
18. Herring WJ, Connor KM, Ivgy-May N, Snyder E, Liu K, Snavely DB, et al. Suvorexant in patients with insomnia: results from two 3-month randomized controlled clinical trials. Biol Psychiatry. (2016) 79:136–48. doi: 10.1016/j.biopsych.2014.10.003
19. Babson KA, Sottile J, Morabito D. Cannabis, cannabinoids, and sleep: a review of the literature. Curr Psychiatry Rep. (2017) 19:23. doi: 10.1007/s11920-017-0775-9
20. Fan M, Sun D, Zhou T, Heianza Y, Lv J, Li L, et al. Sleep patterns, genetic susceptibility, and incident cardiovascular disease: a prospective study of 385 292 UK biobank participants. Eur Heart J. (2020) 41:1182–9. doi: 10.1093/eurheartj/ehz849
21. Delessert A, Espa F, Rossetti A, Lavigne G, Tafti M, Heinzer R. Pulse wave amplitude drops during sleep are reliable surrogate markers of changes in cortical activity. Sleep. (2010) 33:1687–92. doi: 10.1093/sleep/33.12.1687
22. Bosi M, Milioli G, Riccardi S, Melpignano A, Vaudano AE, Cortelli P, et al. Arousal responses to respiratory events during sleep: the role of pulse wave amplitude. J Sleep Res. (2018) 27:259–67. doi: 10.1111/jsr.12593
23. Thomas RJ, Wood C, Bianchi MT. Cardiopulmonary coupling spectrogram as an ambulatory clinical biomarker of sleep stability and quality in health, sleep apnea, and insomnia. Sleep. (2018) 41:zsx196. doi: 10.1093/sleep/zsx196
24. Sateia MJ. International classification of sleep disorders-third edition: highlights and modifications. Chest. (2014) 146:1387–94. doi: 10.1378/chest.14-0970
25. DelRosso LM, Mogavero MP, Ferri R. Restless sleep disorder, restless legs syndrome, and periodic limb movement disorder-Sleep in motion! Pediatr Pulmonol. (2022) 57:1879–86. doi: 10.1002/ppul.25305
26. Picchietti DL, Van Den Eeden SK, Inoue Y, Berger K. Achievements, challenges, and future perspectives of epidemiologic research in restless legs syndrome (RLS). Sleep Med. (2017) 31:3–9. doi: 10.1016/j.sleep.2016.06.007
27. Hornyak M, Feige B, Riemann D, Voderholzer U. Periodic leg movements in sleep and periodic limb movement disorder: prevalence, clinical significance and treatment. Sleep Med Rev. (2006) 10:169–77. doi: 10.1016/j.smrv.2005.12.003
28. Bara-Jimenez W, Aksu M, Graham B, Sato S, Hallett M. Periodic leg movements in sleep: state-dependent excitability of the spinal cord flexor reflex. Neurology. (2000) 54:1609–16. doi: 10.1212/WNL.54.8.1609
29. The AASM Manual for the Scoring of Sleep and Associated Events. Available online at: http://www.aasmnet.org/scoringmanual/default.aspx (accessed May 19, 2022).
30. Ferri R, Fulda S, Allen RP, Zucconi M, Bruni O, Chokroverty S, et al. International and European Restless Legs Syndrome Study Groups (IRLSSG and EURLSSG). World Association of Sleep Medicine (WASM) 2016 standards for recording and scoring leg movements in polysomnograms developed by a joint task force from the International and the European Restless Legs Syndrome Study Groups (IRLSSG and EURLSSG). Sleep Med. (2016) 26:86–95. doi: 10.1016/j.sleep.2016.10.010
31. Schipper MH, Alvarez-Estevez D, Jellema K, Verbraecken J, Fulda S, Rijsman RM. Sleep-related leg movements in obstructive sleep apnea: definitions, determinants, and clinical consequences. Sleep Med. (2020) 75:131–40. doi: 10.1016/j.sleep.2020.06.004
32. Allen RP, Picchietti D, Hening WA, Trenkwalder C, Walters AS, Montplaisi J, et al. Restless legs syndrome: diagnostic criteria, special considerations, and epidemiology. A report from the restless legs syndrome diagnosis and epidemiology workshop at the National Institutes of Health. Sleep Med. (2003) 4:101–19. doi: 10.1016/S1389-9457(03)00010-8
33. Benes H, Walters AS, Allen RP, Hening WA, Kohnen R. Definition of restless legs syndrome, how to diagnose it, and how to differentiate it from RLS mimics. Mov Disord. (2007) 22(Suppl. 18):S401–8. doi: 10.1002/mds.21604
34. Sforza E, Jouny C, Ibanez V. Time course of arousal response during periodic leg movements in patients with periodic leg movements and restless legs syndrome. Clin Neurophysiol. (2003) 114:1116–24. doi: 10.1016/S1388-2457(03)00077-4
35. Hening WA, Walters AS, Wagner M, Rosen R, Chen V, Kim S, et al. Circadian rhythm of motor restlessness and sensory symptoms in the idiopathic restless legs syndrome. Sleep. (1999) 22:901–12. doi: 10.1093/sleep/22.7.901
36. Trenkwalder C, Hening WA, Walters AS, Campbell SS, Rahman K, Chokroverty S. Circadian rhythm of periodic limb movements and sensory symptoms of restless legs syndrome. Mov Disord. (1999) 14:102. doi: 10.1002/1531-8257(199901)14:1<102::AID-MDS1017>3.0.CO;2-E
37. Michaud M, Dumont M, Selmaoui B, Paquet J, Fantini ML, Montplaisir J. Circadian rhythm of restless legs syndrome: relationship with biological markers. Ann Neurol. (2004) 55:372–80. doi: 10.1002/ana.10843
38. Ferri R, Gschliesser V, Frauscher B, Poewe W, Högl B. Periodic leg movements during sleep and periodic limb movement disorder in patients presenting with unexplained insomnia. Clin Neurophysiol. (2009) 120:257–63. doi: 10.1016/j.clinph.2008.11.006
39. Ferri R, DelRosso LM, Silvani A, Cosentino FII, Picchietti DL, Mogavero P, et al. Peculiar lifespan changes of periodic leg movements during sleep in restless legs syndrome. J Sleep Res. (2020) 29:e12896. doi: 10.1111/jsr.12896
40. Pennestri MH, Whittom S, Adam B, Petit D, Carrier J, Montplaisir J. PLMS and PLMW in healthy subjects as a function of age: prevalence and interval distribution. Sleep. (2006) 29:1183–7. doi: 10.1093/sleep/29.9.1183
41. Scholle S, Scholle HC. Leg movements and periodic leg movements during sleep in the development across childhood and adolescence from 1 to 18 years. Sleep Med. (2014) 15:1068–74. doi: 10.1016/j.sleep.2014.04.018
42. Parrino L, Boselli M, Buccino GP, Spaggiari MC, Di Giovanni G, Terzano MG. The cyclic alternating pattern plays a gate-control on periodic limb movements during non-rapid eye movement sleep. J Clin Neurophysiol. (1996) 13:314–23. doi: 10.1097/00004691-199607000-00005
43. Saletu M, Anderer P, Saletu-Zyhlarz G, Prause W, Semler B, Zoghlami A, et al. Restless legs syndrome (RLS) and periodic limb movement disorder (PLMD): acute placebo-controlled sleep laboratory studies with clonazepam. Eur Neuropsychopharmacol. (2001) 11:153–61. doi: 10.1016/S0924-977X(01)00080-3
44. Didato G, Di Giacomo R, Rosa GJ, Dominese A, de Curtis M, Lanteri P. Restless legs syndrome across the lifespan: symptoms, pathophysiology, management and daily life impact of the different patterns of disease presentation. Int J Environ Res Public Health. (2020) 17:3658. doi: 10.3390/ijerph17103658
45. Lubetkin EI, Jia H. Burden of disease due to sleep duration and sleep problems in the elderly. Sleep Heal. (2018) 4:182–7. doi: 10.1016/j.sleh.2017.11.007
46. Ferri R, Rundo F, Zucconi M, Manconi M, Bruni O, Ferini-Strambi L, et al. An evidence-based analysis of the association between periodic leg movements during sleep and arousals in restless legs syndrome. Sleep. (2015) 38:919–24. doi: 10.5665/sleep.4740
47. Gurbani N, Dye TJ, Dougherty K, Jain S, Horn PS, Simakajornboon N. Improvement of parasomnias after treatment of restless leg syndrome/ periodic limb movement disorder in children. J Clin Sleep Med. (2019) 15:743–8. doi: 10.5664/jcsm.7766
48. Newell SA, Drake ME, Jr. Sleep apnea and periodic leg movements in epilepsy. Clin Electroencephalogr. (1994) 25:153–5. doi: 10.1177/155005949402500408
49. Lin CY, Tsai SJ, Peng CK, Yang AC. Sleep state instabilities in patients with periodic limb movements in sleep - Detection and quantification with heart rate variability. Psychiatry Res. (2020) 293:113454. doi: 10.1016/j.psychres.2020.113454
50. Parrino L, Ferri R, Bruni O, Terzano MG. Cyclic alternating pattern (CAP): the marker of sleep instability. Sleep Med Rev. (2012) 16:27–45. doi: 10.1016/j.smrv.2011.02.003
51. Gottlieb DJ, Somers VK, Punjabi NM, Winkelman JW. Restless legs syndrome and cardiovascular disease: a research roadmap. Sleep Med. (2017) 31:10–7. doi: 10.1016/j.sleep.2016.08.008
52. Jin B, Wang A, Earley C, Allen R. Moderate to severe but not mild RLS is associated with greater sleep-related sympathetic autonomic activation than healthy adults without RLS. Sleep Med. (2020) 68:89–95. doi: 10.1016/j.sleep.2019.09.005
53. Montplaisir JY, Whitton S, Rompr, é S, Dang-vu T, Dauvilliers Y, Lavigne G. Prevalence and functional significance of periodic leg movements in narcolepsy. Sleep. (2005) 28(Suppl):A222.
54. Manconi M, Zavalko I, Bassetti CL, Colamartino E, Pons M, Ferri R. Respiratory-related leg movements and their relationship with periodic leg movements during sleep. Sleep. (2014) 37:497–504. doi: 10.5665/sleep.3484
55. Allen RP, Mignot E, Ripley B, Nishino S, Earley CJ. Increased CSF hypocretin-1 (orexin-A) in restless legs syndrome. Neurology. (2002) 59:639–41. doi: 10.1212/WNL.59.4.639
56. Ramanathan S, Hanley JJ, Deniau JM, Bolam JP. Synaptic convergence of motor and somatosensory cortical afferents onto GABAergic interneurons in the rat stri- atum. J Neurosci. (2002) 22:8158–69. doi: 10.1523/JNEUROSCI.22-18-08158.2002
57. Ferré S, García-Borreguero D, Allen RP, Earley CJ. New insights into the neurobiology of restless Legs Syndrome. Neuroscientist. (2019) 25:113–25. doi: 10.1177/1073858418791763
58. Trenkwalder C, Paulus W. Pharmacological treatments of augmentation in restless legs syndrome patients. Adv Pharmacol. (2019) 84:255–65. doi: 10.1016/bs.apha.2019.02.002
59. Lanza G, Ferri R. The neurophysiology of hyperarousal in restless legs syndrome: hints for a role of glutamate/GABA. Adv Pharmacol. (2019) 84:101–19. doi: 10.1016/bs.apha.2018.12.002
60. Ferri R, Zucconi M, Manconi M, Bruni O, Ferini-Strambi L, Vandi S, et al. Different periodicity and time structure of leg movements during sleep in narcolepsy/cataplexy and restless legs syndrome. Sleep. (2006) 29:1587–94. doi: 10.1093/sleep/29.12.1587
61. Droste DW, Krauss JK, Hagedorn G, Kaps M. Periodic leg movements are part of the B-wave rhythm and the cyclic alternating pattern. Acta Neurol Scand. (1996) 94:347–52. doi: 10.1111/j.1600-0404.1996.tb07078.x
62. Terzano MG, Parrino L. Functional relationship between macro and microstructure of sleep. In: Terzano MG, Halasz PL, Declerck AC, editors. Phasic Events and Dynamic Organisation of Sleep. New York, NY: Raven Press (1991). p. 101 ± 19.
63. Mutti C, Ciliento R, Melpignano A, Trippi I, Bernabè G, Zinno L, et al. Can body position and sleep stages influence motor patterns in periodic limb movement disorder?. Sleep Med. (2019) 62:32–3. doi: 10.1016/j.sleep.2019.04.002
64. Karadeniz D, Ondze B, Besset A, Billiard M. EEG arousals and awakenings in relation with periodic leg movements during sleep. J Sleep Res. (2020) 9:273–7. doi: 10.1046/j.1365-2869.2000.00202.x
65. Mirza M, Shen WK, Sofi A, Tran C, Jahangir A, Sultan S, et al. Frequent periodic leg movement during sleep is an unrecognized risk factor for progression of atrial fibrillation. PLoS ONE. (2013) 8:e78359. doi: 10.1371/journal.pone.0078359
66. Kim JS, Azarbarzin A, Wang R, Djonlagic IE, Punjabi NM, Zee PC, et al. Association of novel measures of sleep disturbances with blood pressure: the Multi- Ethnic Study of Atherosclerosis. Thorax. (2020) 75:57–63. doi: 10.1136/thoraxjnl-2019-213533
67. Mirza M, Shen WK, Sofi A, Jahangir A, Mori N, Tajik AJ, et al. (2013). Frequent periodic leg movement during sleep is associated with left ventricular hypertrophy and adverse cardiovascular outcomes. J Am Soc Echocardiogr. (2013) 26:783–90. doi: 10.1016/j.echo.2013.03.018
68. Lombardi C, Parati G, Soranna D, Zambon A, Sliwinski P, Roisman G, et al. Periodic limb movements during sleep and blood pressure changes in sleep apnoea: Data from the European Sleep Apnoea Database. Respirology. (2020) 25:872–9. doi: 10.1111/resp.13760
69. Howard ME, O'Donoghue FJ. Periodic leg movements: a marker of cardiovascular risk? Respirology. (2020) 25:793–4. doi: 10.1111/resp.13795
70. Sforza E, Roche F, Pichot V. Determinants of nocturnal cardiovascular variability and heart rate arousal response in restless legs syndrome (RLS)/periodic limb movements (PLMS). J Clin Med. (2019) 8:1619. doi: 10.3390/jcm8101619
71. Sforza E, Jouny C, Ibanez V. Time-dependent variation in cerebral and autonomic activity during periodic leg movements in sleep: implications for arousal mechanisms. Clin Neurophysiol. (2002) 113:883–91. doi: 10.1016/S1388-2457(02)00066-4
72. Ferri R, Rundo F, Silvani A, Zucconi M, Aricò D, Bruni O, et al. Short-interval leg movements during sleep entail greater cardiac activation than periodic leg movements during sleep in restless legs syndrome patients. J Sleep Res. (2017) 26:602–5. doi: 10.1111/jsr.12529
73. Stein PK, Pu Y. Heart rate variability sleep and sleep disorders. Sleep Med Rev. (2012) 16:47–66. doi: 10.1016/j.smrv.2011.02.005
74. Chiaro G, Manconi M. Restless legs syndrome, periodic limb movements during sleep and cardiovascular risk. Auton Neurosci. (2019) 220:102554. doi: 10.1016/j.autneu.2019.102554
75. Young HA, Benton D. Heart-rate variability: a biomarker to study the influence of nutrition on physiological and psychological health? Behav Pharmacol. (2018) 29:140–51. doi: 10.1097/FBP.0000000000000383
76. Ferri R, Parrino L, Smerieri A, Terzano MG, Elia M, Musumeci SA, et al. Cyclic alternating pattern and spectral analysis of heart rate variability during normal sleep. J Sleep Res. (2000) 9:13–8. doi: 10.1046/j.1365-2869.2000.00190.x
77. Spiegelhalder K, Scholtes C, Riemann D. The association between insomnia and cardiovascular diseases. Nat Sci Sleep. (2010) 4:71–8. doi: 10.2147/NSS.S7471
78. Narkiewicz K, Somers VK. Cardiovascular variability characteristics in obstructive sleep apnea. Auton Neurosci. (2001) 90:89–94. doi: 10.1016/S1566-0702(01)00272-7
79. Ronkainen E, Ansakorpi H, Huikuri HV, Myllylä VV, Isojärvi JI, Korpelainen JT. Suppressed circadian heart rate dynamics in temporal lobe epilepsy. J Neurol Neurosurg Psychiatry. (2005) 76:1382–6. doi: 10.1136/jnnp.2004.053777
80. Mäkikallio TH, Tapanaine JM, Tulppo M, Huikuri HV. Clinical applicability of heart rate variability analysis by methods based on non linear dynamics. Card Electrophysiol Rev. (2002) 6:250–5. doi: 10.1023/A:1016381025759
81. Aurora RN, Kristo DA, Bista SR, Rowley JA, Zak RS, Casey KR, et al. The treatment of restless legs syndrome and periodic limb movement disorder in adults–an update for 2012: Practice parameters with an evidence-based systematic review and meta-analyses: An American Academy of Sleep Medicine Clinical Practice Guideline. Sleep. (2012) 35:1039–62. doi: 10.5665/sleep.1988
82. McNicholas WT, Bonsigore MR, Management Committee of EU COST ACTION B26. Sleep apnoea as an independent risk factor for cardiovascular disease: current evidence, basic mechanisms and research priorities. Eur Respir J. (2007) 29:156–78. doi: 10.1183/09031936.00027406
83. Jelic S, Padeletti M, Kawut SM, Higgins C, Canfield SM, Onat D, et al. Inflammation, oxidative stress, and repair capacity of the vascular endothelium in obstructive sleep apnea. Circulation. (2008) 117:2270–8. doi: 10.1161/CIRCULATIONAHA.107.741512
84. Heinzer R, Vat S, Marques-Vidal P, Marti-Soler H, Andries D, Tobback N, et al. Prevalence of sleep-disordered breathing in the general population: the HypnoLaus study. Lancet Respir Med. (2015) 3:310–8. doi: 10.1016/S2213-2600(15)00043-0
85. Xu J, Wang X, Meng F, Zhao T, Tang T, Wu W, et al. The role of obstructive sleep apnea on the prognosis of pulmonary embolism: A systemic review and meta-analysis. Sleep Breath. (2021) 25:1419–26. doi: 10.1007/s11325-020-02258-z
86. D'Souza H, Kapoor KG. Retinal vascular manifestations of obstructive sleep apnea. Curr Opin Ophthalmol. (2020) 31:508–13. doi: 10.1097/ICU.0000000000000698
87. Shah NM, Malhotra AM, Kaltsakas G. Sleep disorder in patients with chronic liver disease: a narrative review. J Thorac Dis. (2020) 12:S248–60. doi: 10.21037/jtd-cus-2020-012
88. Shapira-Daniels A, Mohanty S, Contreras-Valdes FM, Tieu H, Thomas RJ, Natale A, et al. Prevalence of undiagnosed sleep apnea in patients with atrial fibrillation and its impact on therapy. JACC Clin Electrophysiol. (2020) 6:1499–506. doi: 10.1016/j.jacep.2020.05.030
89. Lui B, Burey L, Ma X, Kjaer K, Abramovitz SE, White RS. Obstructive sleep apnea is associated with adverse maternal outcomes using a United States multistate database cohort, 2007–2014. Int J Obstet Anesth. (2021) 45:74–82. doi: 10.1016/j.ijoa.2020.10.007
90. Chandrakantan A, Adler AC, Tohsun M, Kheradamand F, Ray RS, Roth S. Intermittent hypoxia and effects on early learning/memory: Exploring the hippocampal cellular effects of pediatric obstructive sleep apnea. Anesth Analg. (2021) 133:93–103. doi: 10.1213/ANE.0000000000005273
91. Liguori C, Maestri M, Spanetta M, Placidi F, Bonanni E, Mercuri NB, et al. Sleep-disordered breathing and the risk of Alzheimer's disease. Sleep Med Rev. (2020) 55:101375. doi: 10.1016/j.smrv.2020.101375
92. Shiota S, Takekawa H, Matsumoto SE, Takeda K, Nurwidya F, Yoshioka Y, et al. Chronic intermittent hypoxia/reoxygenation facilitate amyloid-β generation in mice. J Alzheimers Dis. (2013) 37:325–33. doi: 10.3233/JAD-130419
93. Bishir M, Bhat A, Essa MM, Ekpo O, Ihunwo AO, Veeraraghavan VP, et al. (2020). Sleep deprivation and neurological disorders. Biomed Res Int. 2020:5764017. doi: 10.1155/2020/5764017
94. Tkacova R, Niroumand M, Lorenzi-Filho G, Bradley TD. Overnight shift from obstructive to central apneas in patients with heart failure: role of PCO2 and circulatory delay. Circulation. (2001) 103:238–43. doi: 10.1161/01.CIR.103.2.238
95. D'Cruz RF, Murphy PB, Kaltsakas G. Sleep disordered breathing and chronic obstructive pulmonary disease: a narrative review on classification, pathophysiology and clinical outcomes. J Thorac Dis. (2020) 12:S202–16. doi: 10.21037/jtd-cus-2020-006
96. Benjafield AV, Ayas NT, Eastwood PR, Heinzer R, Ip M, Morrell MJ, et al. Estimation of the global prevalence and burden of obstructive sleep apnoea: a literature-based analysis. Lancet Respir Med. (2019) 7:687–98. doi: 10.1016/S2213-2600(19)30198-5
97. Bazzano LA, Hu T, Bertisch SM, Yao L, Harville EW, Gustat J, et al. Childhood obesity patterns and relation to middle-age sleep apnoea risk: the Bogalusa Heart Study. Pediatr Obes. (2016) 11:535–42. doi: 10.1111/ijpo.12103
98. Osman AM, Carter SG, Carberry JC, Eckert DJ. Obstructive sleep apnea: current perspectives. Nat Sci Sleep. (2018) 10:21–34. doi: 10.2147/NSS.S124657
100. Ciscar MA, Juan G, Martínez V, Ramón M, Lloret T, Mínguez J, et al. Magnetic resonance imaging of the pharynx in OSA patients and healthy subjects. Eur Respir J. (2001) 17:79–86. doi: 10.1183/09031936.01.17100790
101. Eckert DJ. Phenotypic approaches to obstructive sleep apnoea–new pathways for targeted therapy. Sleep Med Rev. (2018) 37:45–59. doi: 10.1016/j.smrv.2016.12.003
102. Carberry JC, Amatoury J, Eckert DJ. Personalized management approach for OSA. Chest. (2018) 153:744–55. doi: 10.1016/j.chest.2017.06.011
103. Redolfi S, Yumino D, Ruttanaumpawan P, Yau B, Su MC, Lam J, et al. Relationship between overnight rostral fluid shift and Obstructive Sleep Apnea in nonobese men. Am J Respir Crit Care Med. (2009) 179:241–6. doi: 10.1164/rccm.200807-1076OC
104. Jones RL, Nzekwu MM. The effects of body mass index on lung volumes. Chest. (2006) 130:827–33. doi: 10.1378/chest.130.3.827
105. Orr JE, Malhotra A, Sands SA. Pathogenesis of central and complex sleep apneas. Respirol Carlton Vic. (2017) 22:43–52. doi: 10.1111/resp.12927
106. Pavsic K, Herkenrath S, Treml M, Hagmeyer L, Khayat RN, Hellmich M, et al. Mixed apnea metrics in obstructive sleep apnea predict treatment-emergent central sleep apnea. Am J Respir Crit Care Med. (2021) 203:772–5. doi: 10.1164/rccm.202007-2816LE
107. Zinchuk A, Edwards BA, Jeon S, Koo BB, Concato J, Sands S, et al. Prevalence, associated clinical features, and impact on continuous positive airway pressure use of a low respiratory arousal threshold among male united states veterans with obstructive sleep apnea. J Clin Sleep Med. (2018) 14:809–17. doi: 10.5664/jcsm.7112
108. Messineo L, Taranto-Montemurro L, Azarbarzin A, Oliveira Marques MD, Calianese N, White DP, et al. Breath-holding as a means to estimate the loop gain contribution to obstructive sleep apnoea. J Physiol. (2018) 596:4043–56. doi: 10.1113/JP276206
109. Wellman A, Malhotra A, Jordan AS, Stevenson KE, Gautam S, White DP. Effect of oxygen in obstructive sleep apnea: role of loop gain. Respir Physiol Neurobiol. (2008) 162:144–51. doi: 10.1016/j.resp.2008.05.019
110. Edwards BA, Sands SA, Eckert DJ, White DP, Butler JP, Owens RL, et al. Acetazolamide improves loop gain but not the other physiological traits causing obstructive sleep apnoea. J Physiol. (2012) 590:1199–211. doi: 10.1113/jphysiol.2011.223925
111. Thomas RJ, Terzano MG, Parrino L, Weiss JW. Obstructive sleep-disordered breathing with a dominant cyclic alternating pattern–a recognizable polysomnographic variant with practical clinical implications. Sleep. (2004) 27:229–34. doi: 10.1093/sleep/27.2.229
112. Joosten SA, Landry SA, Wong AM, Mann DL, Terrill PI, Sands SA, et al. (2020). Assessing the physiologic endotypes responsible for REM and NREM Based OSA. Chest.
113. Terzano MG, Parrino L, Boselli M, Spaggiari MC, Di Giovanni G. Polysomnographic analysis of arousal responses in obstructive sleep apnea syndrome by means of the cyclic alternating pattern. J Clin Neurophysiol. (1996) 13:145–55. doi: 10.1097/00004691-199603000-00005
114. Gnoni V, Drakatos P, Higgins S, Duncan I, Wasserman D, Kabiljo R, et al. Cyclic alternating pattern in obstructive sleep apnea: a preliminary study. J Sleep Res. (2021) 30:e13350. doi: 10.1111/jsr.13350
115. Kaur S, Saper CB. Neural circuitry underlying waking up to hypercapnia. Front Neurosci. (2019) 13:401. doi: 10.3389/fnins.2019.00401
116. Remmers JE, deGroot WJ, Sauerland EK, Anch AM. Pathogenesis of upper airway occlusion during sleep. J Appl Physiol Respir Environ Exerc Physiol. (1978) 44:931–8. doi: 10.1152/jappl.1978.44.6.931
117. Sands SA, Terrill PI, Edwards BA, Taranto Montemurro L, Azarbarzin A, Marques M, et al. Quantifying the arousal threshold using polysomnography in obstructive sleep apnea. Sleep. (2018) 41:zsx183. doi: 10.1093/sleep/zsx183
118. Koo BB, Mansour A. Correlates of obstructive apnea duration. Lung. (2014) 192:185–190. doi: 10.1007/s00408-013-9510-4
119. Saraç S, Afsar GC. Effect of mean apnea-hypopnea duration in patients with obstructive sleep apnea on clinical and polysomnography parameter. Sleep Breath. (2020) 24:77–81. doi: 10.1007/s11325-019-01870-y
120. Mutti C, Bernabè G, Barozzi N, Ciliento R, Trippi I, Pedrazzi G, et al. Commonalities and differences in NREM parasomnias and sleep-related epilepsy: Is there a continuum between the two conditions? Front Neurol. (2020) 11:600026. doi: 10.3389/fneur.2020.600026
121. Manni R, Terzaghi M. Comorbidity between epilepsy and sleep disorders. Epilepsy Res. (2010) 90:171–7. doi: 10.1016/j.eplepsyres.2010.05.006
122. Shaheen HA, Abd El-Kader AA, El Gohary AM, El-Fayoumy NM, Afifi LM. Obstructive sleep apnea in epilepsy: a preliminary Egyptian study. Sleep Breath. (2012) 16:765–71. doi: 10.1007/s11325-011-0573-2
123. Malow BA, Levy K, Maturen K, Bowes R. Obstructive sleep apnea is common in medically refractory epilepsy patients. Neurology. (2000) 55:1002–7. doi: 10.1212/WNL.55.7.1002
124. Jaseja H, Goyal M, Mishra P. Drug-resistant epilepsy and obstructive sleep apnea: exploring a link between the two. World Neurosurg. (2021) 146:210–4. doi: 10.1016/j.wneu.2020.11.099
125. Budhiraja R, Javaheri S, Pavlova MK, Epstein LJ, Omobomi O, Quan SF. Prevalence and correlates of periodic limb movements in OSA and the effect of CPAP therapy. Neurology. (2020) 94:e1820–7. doi: 10.1212/WNL.0000000000008844
126. Milagro J, Deviaene M, Gil E, Lázaro J, Buyse B, Testelmans D, et al. Autonomic dysfunction increases cardiovascular risk in the presence of sleep apnea. Front Physiol. (2019) 10:620. doi: 10.3389/fphys.2019.00620
127. Bušek P, Van?ková J, Opavský J, Salinger J, Nevšímalová S. Spectral analysis of heart rate variability in sleep. Physiol Res. (2005) 54:369–76. doi: 10.33549/physiolres.930645
128. Liang J, Zhang X, Luo Y, Wang T, Sun L, Huang S. The impact of respiratory events on the autonomic nervous system during sleep. Int Heart J. (2018) 59:378–86. doi: 10.1536/ihj.16-429
129. Malhotra A, Mesarwi O, Pepin JL, Owens RL. Endotypes and phenotypes in obstructive sleep apnea. Curr Opin Pulm Med. (2020) 26:609–14. doi: 10.1097/MCP.0000000000000724
130. Bailly S, Grote L, Hedner J, Schiza S, McNicholas WT, Basoglu OK, et al. Clusters of sleep apnoea phenotypes: A large pan-European study from the European Sleep Apnoea Database (ESADA). Respirology. (2021) 26:378–87. doi: 10.1111/resp.13969
131. Ye L, Pien GW, Ratcliffe SJ, Björnsdottir E, Arnardottir ES, Pack AI, et al. The different clinical faces of obstructive sleep apnoea: a cluster analysis. Eur Respir J. (2014) 44:1600–7. doi: 10.1183/09031936.00032314
132. Chiang CL, Chen YT, Wang KL, Su VY, Wu LA, Perng DW, et al. Comorbidities and risk of mortality in patients with sleep apnea. Ann Med. (2017) 49:377–83. doi: 10.1080/07853890.2017.1282167
133. Yang X, Xiao Y, Han B, Lin K, Niu X, Chen X. Implication of mixed sleep apnea events in adult patients with obstructive sleep apnea-hypopnea syndrome. Sleep Breath. (2019) 23:559–65. doi: 10.1007/s11325-018-1745-0
134. Zhang J, Wang L, Guo HJ, Wang Y, Cao J, Chen BY. Treatment-emergent central sleep apnea: a unique sleep-disordered breathing. Chin Med J. (2020) 133:2721–30. doi: 10.1097/CM9.0000000000001125
135. Aurora RN, Crainiceanu C, Gottlieb DJ, Kim JS, Punjabi NM. Obstructive sleep apnea during REM sleep and cardiovascular disease. Am J Respir Crit Care Med. (2018) 197:653–60. doi: 10.1164/rccm.201706-1112OC
136. Azarbarzin A, Sands SA, Younes M, Taranto-Montemurro L, Sofer T, Vena D, et al. The sleep apnea-specific pulse-rate response predicts cardiovascular morbidity and mortality. Am J Respir Crit Care Med. (2021) 203:1546–55. doi: 10.1164/rccm.202010-3900OC
137. Azarbarzin A, Sands SA, Taranto-Montemurro L, Vena D, Sofer T, Kim SW, et al. The sleep apnea-specific hypoxic burden predicts incident heart failure. Chest. (2020) 158:739–50. doi: 10.1016/j.chest.2020.03.053
138. Ambati A, Ju YE, Lin L, Olesen AN, Koch H, Hedou JJ, et al. Proteomic biomarkers of sleep apnea. Sleep. (2020) 43:zsaa086. doi: 10.1093/sleep/zsaa086
139. Zhang X, Wang S, Xu H, Yi H, Guan J, Yin S. Metabolomics and microbiome profiling as biomarkers in obstructive sleep apnoea: a comprehensive review. Eur Respir Rev. (2021) 30:200220. doi: 10.1183/16000617.0220-2020
140. Sawyer AM, Gooneratne NS, Marcus CL, Ofer D, Richards KC, Weaver TE. A systematic review of CPAP adherence across age groups: clinical and empiric insights for developing CPAP adherence interventions. Sleep Med Rev. (2011) 343–56. doi: 10.1016/j.smrv.2011.01.003
141. Johns MW. Daytime sleepiness, snoring, and obstructive sleep apnea. The Epworth Sleepiness Scale. Chest. (1993) 103:30–6. doi: 10.1378/chest.103.1.30
142. Punjabi NM, Lim D. Pro. Sleep fragmentation causes hypersomnolence in sleep apnea: the convergence of rationalism and empiricism. Sleep Med Rev. (2021) 55:101397. doi: 10.1016/j.smrv.2020.101397
143. Veasey SC, Davis CW, Fenik P, Zhan G, Hsu YJ, Pratico D, et al. Long-term intermittent hypoxia in mice: protracted hypersomnolence with oxidative injury to sleep-wake brain regions. Sleep. (2004) 27:194–201. doi: 10.1093/sleep/27.2.194
144. Gold AR, Gold MS. Con: sleep fragmentation causes hypersomnolence in OSA. Sleep Med Rev. (2021) 55:101399. doi: 10.1016/j.smrv.2020.101399
145. Kapur VK, Baldwin CM, Resnick HE, Gottlieb DJ, Nieto FJ. Sleepiness in patients with moderate to severe sleep-disordered breathing. Sleep. (2005) 28:472–7. doi: 10.1093/sleep/28.4.472
146. Guilleminault C, Lopes MC, Hagen CC, da Rosa A. The cyclic alternating pattern demonstrates increased sleep instability and correlates with fatigue and sleepiness in adults with upper airway resistance syndrome. Sleep. (2007) 30:641–7. doi: 10.1093/sleep/30.5.641
147. Bao G, Guilleminault C. Upper airway resistance syndrome–one decade later. Curr Opin Pulm Med. (2004) 10:461–7. doi: 10.1097/01.mcp.0000143689.86819.c2
148. Korkmaz S, Bilecenoglu NT, Aksu M, Yoldas TK. Cyclic alternating pattern in obstructive sleep apnea patients with versus without excessive sleepiness. Sleep Disord. (2018) 2018:8713409. doi: 10.1155/2018/8713409
149. Melpignano A, Milioli G, Bertel, è A, Vaudano AE, Ghetti C, et al. Kleine-Levin syndrome: the contribution of PET scan and hydrogen breath test for small intestinal bacterial overgrowth. Sleep Med. (2018) 46:95–7. doi: 10.1016/j.sleep.2018.03.012
150. Arentz M, Yim E, Klaff L, Lokhandwala S, Riedo FX, Chong M, et al. Characteristics and outcomes of 21 critically ill patients with COVID-19 in Washington State. JAMA. (2020) 323:1612–4. doi: 10.1001/jama.2020.4326
151. Pena Orbea C, Wang L, Shah V, Jehi L, Milinovich A, Foldvary-Schaefer N, et al. Association of sleep-related hypoxia with risk of COVID-19 hospitalizations and mortality in a large integrated health system. JAMA Netw Open. (2021) 4:e2134241. doi: 10.1001/jamanetworkopen.2021.34241
152. Mutti C, Azzi N, Soglia M, Pollara I, Alessandrini F, Parrino L. Obstructive sleep apnea, cpap and COVID-19: A brief review. Acta Biomed. (2020) 91:e2020196. doi: 10.23750/abm.v91i4.10941
153. Stallman HM, Kohler M. Prevalence of sleepwalking: a systematic review and meta-analysis. PLoS ONE. (2016) 11:e0164769. doi: 10.1371/journal.pone.0164769
154. Silber MH. Parasomnias occurring in non-rapid eye movement sleep. Continuum. (2020) 26:946–62. doi: 10.1212/CON.0000000000000877
155. Parrino L, Halasz P, Tassinari CA, Terzano MG. CAP, epilepsy and motor events during sleep: the unifying role of arousal. Sleep Med Rev. (2006) 10:267–85. doi: 10.1016/j.smrv.2005.12.004
156. Ohayon MM, Mahowald MWY MD, Krystal AD, Léger D. Prevalence and comorbidity of nocturnal wandering in the US adult general population. Neurology. (2012) 78:1583–9. doi: 10.1212/WNL.0b013e3182563be5
157. Heidbreder A, Frauscher B, Mitterling T, Boentert M, Schirmacher A, Hörtnagl P, et al. Not only sleepwalking but NREM parasomnia irrespective of the type is associated with HLA DQB1*05:01. J Clin Sleep Med. (2016) 12:565–70. doi: 10.5664/jcsm.5692
158. Halász P, Szucs A, Mutti C, Parrino L. Disorders of arousal and sleep-related hypermotor epilepsy - overview and challenges night is a battlefield of sleep and arousal promoting forces. Neurol Sci. (2022) 43:927–37. doi: 10.1007/s10072-021-05857-0
159. Guilleminault C, Moscovitch A, Leger D. Forensic sleep medicine: nocturnal wandering and violence. Sleep 1995. (1985) 18:740–8. doi: 10.1093/sleep/18.9.740
160. Schenck CH, Milner DM, Hurwitz TD, Bundlie SR, Mahowald MW. A polysomnographic and clinical report on sleep-related injury in 100 adult patients. Am J Psychiatry. (1989) 146:1166–73. doi: 10.1176/ajp.146.9.1166
161. Baldini T, Loddo G, Sessagesimi E, Mignani F, Cirignotta F, Mondini S, et al. Clinical features and pathophysiology of disorders of arousal in adults: A window into the sleeping brain. Front Neurol. (2019) 10:526. doi: 10.3389/fneur.2019.00526
162. Bassetti C, Vella S, Donati F, Wielepp P, Weder B. SPECT during sleepwalking. Lancet. (2000) 356:484–5. doi: 10.1016/S0140-6736(00)02561-7
163. Terzaghi M, Sartori I, Tassi L, Rustioni V, Proserpio P, Lorusso G, et al. Dissociated local arousal states underlying essential clinical features of non-rapid eye movement arousal parasomnia: an intracerebral stereo-electroencephalographic study. J Sleep Res. (2012) 21:502–6. doi: 10.1111/j.1365-2869.2012.01003.x
164. Irfan M, Schenck CH, Howell MJ. NonREM disorders of arousal and related parasomnias: an updated review. Neurotherapeutics. (2021) 18:124–39. doi: 10.1007/s13311-021-01011-y
165. Gibbs SA, Proserpio P, Francione S, Mai R, Cardinale F, Sartori I, et al. Clinical features of sleep-related hypermotor epilepsy in relation to the seizure-onset zone: a review of 135 surgically treated cases. Epilepsia. (2019) 60:707–17. doi: 10.1111/epi.14690
166. Scheffer IE, Bhatia KP, Lopes-Cendes I, Fish DR, Marsden CD, Andermann E, et al. Autosomal dominant nocturnal frontal lobe epilepsy. A distinctive clinical disorder. Brain. (1995) 118 (Pt 1):61–73. doi: 10.1093/brain/118.1.61
167. Møller RS, Dahl HA, Helbig I. The contribution of next generation sequencing to epilepsy genetics. Expert Rev Mol Diagn. (2015) 15:1531–8. doi: 10.1586/14737159.2015.1113132
168. Provini F, Plazzi G, Tinuper P, Vandi S, Lugaresi E, Montagna P. Nocturnal frontal lobe epilepsy. a clinical and polygraphic overview of 100 consecutive cases. Brain. (1999) 122 (Pt 6):1017–31. doi: 10.1093/brain/122.6.1017
169. Oldani A, Zucconi M, Asselta R, Modugno M, Bonati MT, Dalprà L, et al. Autosomal dominant nocturnal frontal lobe epilepsy. A video-polysomnographic and genetic appraisal of 40 patients and delineation of the epileptic syndrome. Brain. (1998) 121 (Pt 2):205–23. doi: 10.1093/brain/121.2.205
170. Licchetta L, Bisulli F, Vignatelli L. Sleep-related hypermotor epilepsy: long-term outcome in a large cohort Neurology. (2017) 88:70–7. doi: 10.1212/WNL.0000000000003459
171. Wan H, Wang X, Chen Y, Jiang B, Chen Y, Hu W, et al. Sleep-related hypermotor epilepsy: etiology, electro-clinical features, and therapeutic strategies. Nat Sci Sleep. (2021) 13:2065–84. doi: 10.2147/NSS.S330986
172. Derry CP, Harvey AS, Walker MC, Duncan JS, Berkovic SF. NREM arousal parasomnias and their distinction from nocturnal frontal lobe epilepsy: a video EEG analysis. Sleep. (2009) 32:1637–44. doi: 10.1093/sleep/32.12.1637
173. Loddo G, Baldassarri L, Zenesini C, Licchetta L, Bisulli F, Cirignotta F, et al. Seizures with paroxysmal arousals in sleep-related hypermotor epilepsy (SHE): Dissecting epilepsy from NREM parasomnias. Epilepsia. (2020) 61:2194–202. doi: 10.1111/epi.16659
174. Zucconi M, Oldani A, Smirne S, Ferini-Strambi L. The macrostructure and microstructure of sleep in patients with autosomal dominant nocturnal frontal lobe epilepsy. J Clin Neurophysiol. (2000) 17:77–86. doi: 10.1097/00004691-200001000-00008
175. Halász P, Kelemen A, Szűcs A. Physiopathogenetic interrelationship between nocturnal frontal lobe epilepsy and NREM arousal parasomnias. Epilepsy Res Treat. (2012) 2012:312693. doi: 10.1155/2012/312693
177. Tinuper P, Bisulli F, Cross JH, Hesdorffer D, Kahane P, Nobili L, et al. Definition and diagnostic criteria of sleep-related hypermotor epilepsy. Neurology. (2016) 86:1834–42. doi: 10.1212/WNL.0000000000002666
178. Nobili L, Cardinale F, Magliola U, Cicolin A, Didato G, Bramerio M, et al. Taylor's focal cortical dysplasia increases the risk of sleep-related epilepsy. Epilepsia. (2009) 50:2599–604. doi: 10.1111/j.1528-1167.2009.02169.x
179. Chokroverty S. Sleep-related hypermotor syndrome: an arousal parasomnia or nocturnal frontal lobe epilepsy? Sleep Med. (2018) 41:110–1. doi: 10.1016/j.sleep.2017.09.007
180. Januszko P, Niemcewicz S, Gajda T. Sleepwalking episodes are preceded by arousal-related activation in the cingulate motor area: EEG current density imaging. Clin Neurophysiol. (2016) 127:530–6. doi: 10.1016/j.clinph.2015.01.014
181. Mazars G. Criteria for identifying cingulate epilepsies. Epilepsia. (1970) 11:41–7. doi: 10.1111/j.1528-1157.1970.tb03865.x
182. Bancaud J, Talairach J. Clinical semiology of frontal lobe seizures. Adv Neurol. (1992) 57:3–58.
183. Alkawadri R, So NK, Van Ness PC, Alexopoulos AV. Cingulate epilepsy: report of 3 electroclinical subtypes with surgical outcomes. JAMA Neurol. (2013) 70:995–1002. doi: 10.1001/jamaneurol.2013.2940
184. Chou CC, Lee CC, Lin CF, Chen YH, Peng SJ, Hsiao FJ, et al. Cingulate gyrus epilepsy: semiology, invasive EEG, and surgical approaches. Neurosurg Focus. (2020) 48:E8. doi: 10.3171/2020.1.FOCUS19914
185. Rheims S, Ryvlin P, Scherer C, Minotti L, Hoffmann D, Guenot M, et al. Analysis of clinical patterns and underlying epileptogenic zones of hypermotor seizures. Epilepsia. (2008) 49:2030–40. doi: 10.1111/j.1528-1167.2008.01675.x
186. Tassinari CA, Cantalupo G, Högl B, Cortelli P, Tassi L, Francione S, et al. Neuroethological approach to frontolimbic epileptic seizures and parasomnias: the same central pattern generators for the same behaviours. Rev Neurol. (2009) 165:762–8. doi: 10.1016/j.neurol.2009.08.002
187. Halász P. Are absence epilepsy and nocturnal frontal lobe epilepsy system epilepsies of the sleep/wake system? Behav. Neurolo. (2015) 2015:231676. doi: 10.1155/2015/231676
188. Bonini F, McGonigal A, Trébuchon A, Gavaret M, Bartolomei F, Giusiano B, et al. Frontal lobe seizures: from clinical semiology to localization. Epilepsia. (2014) 55:264–77. doi: 10.1111/epi.12490
189. Castelnovo A, Lopez R, Proserpio P, Nobili L, Dauvilliers Y. NREM sleep parasomnias as disorders of sleep-state dissociation. Nat Rev Neurol. (2018) 14:470–81. doi: 10.1038/s41582-018-0030-y
190. Szucs A, Mutti C, Papp A, Halasz P, Parrino L. REM sleep, REM parasomnias, REM sleep behavior disorder. Ideggyógyászati Szemle. (2022) 75:171–82. doi: 10.18071/isz.75.0171
191. Carskadon MA, Dement WC. Monitoring and staging human sleep. In: Kryger MH, Roth R, Dement WC, editors. Principles and Practice of Sleep Medicine. 5th ed. St. Louis: Elsevier Saunders (2011). p. 16–26.
192. Saper CB, Fuller PM. Wake-sleep circuitry: an overview. Curr Opin Neurobiol. (2017) 44:186–92. doi: 10.1016/j.conb.2017.03.021
193. Fuller PM, Saper CB, Lu J. The pontine REM switch: past and present. J Physiol. (2007) 584(Pt 3):735–41. doi: 10.1113/jphysiol.2007.140160
194. Fraigne JJ, Torontali ZA, Snow MB, Peever JH. REM sleep at its core - circuits, neurotransmitters, and pathophysiology. Front Neurol. (2015) 6:123. doi: 10.3389/fneur.2015.00123
195. Grace KP, Horner RL. A focal inactivation and computational study of ventrolateral periaqueductal gray and deep mesencephalic reticular nucleus involvement in sleep state switching and bistability. eNeuro. (2020) 7:ENEURO.0451-19.2020. doi: 10.1523/ENEURO.0451-19.2020
196. Jego S, Glasgow SD, Herrera CG, Ekstrand M, Reed SJ, Boyce R, et al. Optogenetic identification of a rapid eye movement sleep modulatory circuit in the hypothalamus. Nat Neurosci. (2013) 16:1637–43. doi: 10.1038/nn.3522
197. Boissard R, Fort P, Gervasoni D, Barbagli B, Luppi PH. Localization of the GABAergic and non-GABAergic neurons projecting to the sublaterodorsal nucleus and potentially gating paradoxical sleep onset. Eur J Neurosci. (2003) 18:1627–39. doi: 10.1046/j.1460-9568.2003.02861.x
198. Feng H, Wen SY, Qiao QC, Pang YJ, Wang SY, Li HY, et al. Orexin signaling modulates synchronized excitation in the sublaterodorsal tegmental nucleus to stabilize REM sleep. Nat Commun. (2020) 11:3661. doi: 10.1038/s41467-020-17401-3
199. Boyce R, Williams S, Adamantidis A. REM sleep and memory. Curr Opin Neurobiol. (2017) 44:167–77. doi: 10.1016/j.conb.2017.05.001
200. Puentes-Mestril C, Roach J, Niethard N, Zochowski M, Aton SJ. How rhythms of the sleeping brain tune memory and synaptic plasticity. Sleep. (2019) 42:zsz095. doi: 10.1093/sleep/zsz095
201. Rasch B, Born J. About sleep's role in memory. Physiol Rev. (2013) 93:681–766. doi: 10.1152/physrev.00032.2012
202. Sterpenich V, Albouy G, Boly M, Vandewalle G, Darsaud A, Balteau E, et al. Sleep-related hippocampo-cortical interplay during emotional memory recollection. PLoS Biol. (2007) 5:e282. doi: 10.1371/journal.pbio.0050282
203. Lewis PA, Knoblich G, Poe G. How memory replay in sleep boosts creative problem-solving. Trends Cogn Sci. (2018) 22:491–503. doi: 10.1016/j.tics.2018.03.009
204. Lerner I, Lupkin SM, Tsai A, Khawaja A, Gluck MA. Sleep to remember, sleep to forget: rapid eye movement sleep can have inverse effects on recall and generalization of fear memories. Neurobiol Learn Mem. (2021) 180:107413. doi: 10.1016/j.nlm.2021.107413
205. Hobson JA, Pace-Schott EF, Stickgold R. Dreaming and the brain: toward a cognitive neuroscience of conscious states. Behav Brain Sci. (2001) 23:793–1121. doi: 10.1017/CBO9780511615511.003
206. Parrino L, Vaudano AE. The resilient brain and the guardians of sleep: new perspectives on old assumptions. Sleep Med Rev. (2018) 39:98–107. doi: 10.1016/j.smrv.2017.08.003
207. Luppi PH, Clément O, Sapin E, Gervasoni D, Peyron C, Léger L, et al. The neuronal network responsible for paradoxical sleep and its dysfunctions causing narcolepsy and rapid eye movement (REM) behavior disorder. Sleep Med Rev. (2011) 15:153–63. doi: 10.1016/j.smrv.2010.08.002
208. Arrigoni E, Chen MC, Fuller PM. The anatomical, cellular and synaptic basis of motor atonia during rapid eye movement sleep. J Physiol. (2016) 594.19:5391–414. doi: 10.1113/JP271324
209. Khroud NK, Reddy V, Saadabadi A. Neuroanatomy, locus ceruleus. In: StatPearls. Treasure Island, FL: StatPearls Publishing (2020). Available from: https://www.ncbi.nlm.nih.gov/books/NBK513270/ (accessed May 13, 2022).
210. Kompanje EJ. 'The devil lay upon her and held her down'. Hypnagogic hallucinations and sleep paralysis described by the Dutch physician Isbrand van Diemerbroeck (1609-74) in 1664. J Sleep Res. (2008) 17:464–7. doi: 10.1111/j.1365-2869.2008.00672.x
211. Denis D, French CC, Rowe R, Zavos HM, Nolan PM, Parsons MJ, et al. A twin and molecular genetics study of sleep paralysis and associated factors. J Sleep Res. (2015) 24:438–46. doi: 10.1111/jsr.12282
212. Denis D, Poerio GL. Terror and bliss? Commonalities and distinctions between sleep paralysis, lucid dreaming, and their associations with waking life experiences. J Sleep Res. (2017) 26:38–47. doi: 10.1111/jsr.12441
213. Waters F, Blom JD, Dang-Vu TT, Cheyne AJ, Alderson-Day B, Woodruff P, et al. What is the link between hallucinations. Dreams, and hypnagogic-hypnopompic experiences? Schizophr Bull. (2016) 42:1098–109. doi: 10.1093/schbul/sbw076
214. Ohayon MM, Schenck CH. Violent behavior during sleep: prevalence, comorbidity and consequences. Sleep Med. (2010) 11:941–6. doi: 10.1016/j.sleep.2010.02.016
215. Schenck CH, Bundlie SR, Ettinger MG, Mahowald MW. Chronic behavioral disorders of human REM sleep: a new category of parasomnia. Sleep. (1986) 9:293–308. doi: 10.1093/sleep/9.2.293
216. Högl B, Stefani A. REM sleep behavior disorder (RBD): Update on diagnosis and treatment. Somnologie. (2017) 21(Suppl. 1):1–8. doi: 10.1007/s11818-016-0048-6
217. Melpignano A, Parrino L, Santamaria J, Gaig C, Trippi I, Serradell M, et al. Isolated rapid eye movement sleep behavior disorder and cyclic alternating pattern: is sleep microstructure a predictive parameter of neurodegeneration?. Sleep. (2019) 42:zsz142. doi: 10.1093/sleep/zsz142
218. Zhang F, Niu L, Liu X, Liu Y, Li S, Yu H, et al. Rapid eye movement sleep behavior disorder and neurodegenerative diseases: an update. Aging Dis. (2020) 11:315–26. doi: 10.14336/AD.2019.0324
219. Schenck CH, Boeve BF, Mahowald MW. Delayed emergence of a parkinsonian disorder or dementia in 81% of older men initially diagnosed with idiopathic rapid eye movement sleep behavior disorder: a 16-year update on a previously reported series. Sleep Med. (2013) 14:744–8. doi: 10.1016/j.sleep.2012.10.009
220. Howell MJ, Schenck CH. Rapid eye movement sleep behavior disorder and neurodegenerative disease. JAMA Neurol. (2015) 72:707–12. doi: 10.1001/jamaneurol.2014.4563
221. Postuma RB, Iranzo A, Hu M, Högl B, Boeve BF, Manni R, et al. Risk and predictors of dementia and parkinsonism in idiopathic REM sleep behaviour disorder: a multicentre study. Brain. (2019) 142:744–59. doi: 10.1093/brain/awz030
222. Jung Y, St Louis EK. Treatment of REM sleep behavior disorder. Curr Treat Options Neurol. (2016) 18:50. doi: 10.1007/s11940-016-0433-2
223. Aurora RN, Zak RS, Maganti RK, Auerbach SH, Casey KR, Chowdhuri S, et al. (2010). Best practice guide for the treatment of REM sleep behavior disorder (RBD). J Clin Sleep Med. 6:85–95. doi: 10.5664/jcsm.27717
224. Du Y, Jiang J, Ng CH, Wu L, Zhang P, Xi C, et al. Vortioxetine improves rapid eye movement sleep behavior disorder: a case report. Medicine. (2020) 99:e21003. doi: 10.1097/MD.0000000000021003
225. Unger MM, Belke M, Menzler K, Heverhagen JT, Keil B, Stiasny-Kolster K, et al. Diffusion tensor imaging in idiopathic REM sleep behavior disorder reveals microstructural changes in the brainstem, substantia nigra, olfactory region, and other brain regions. Sleep. (2010) 33:767–73. doi: 10.1093/sleep/33.6.767
226. Campabadal A, Inguanzo A, Segura B, Serradell M, Abos A, Uribe C, et al. Cortical gray matter progression in idiopathic REM sleep behavior disorder and its relation to cognitive decline. NeuroImage Clin. (2020) 28:102421. doi: 10.1016/j.nicl.2020.102421
227. Bae YJ, Kim JM, Kim KJ, Kim E, Park HS, Kang SY, et al. Loss of substantia nigra hyperintensity at 3.0-T MR imaging in idiopathic REM sleep behavior disorder: comparison with 123I-FP-CIT SPECT. Radiology. (2018) 287:285–93. doi: 10.1148/radiol.2017162486
228. Miyamoto M, Miyamoto T. Relationship of substantia nigra hyperechogenicity to risk of Lewy body disease in idiopathic REM sleep behavior disorder patients: a longitudinal study. Sleep Med. (2020) 68:31–4. doi: 10.1016/j.sleep.2019.09.008
229. Pyatigorskaya N, Gaurav R, Arnaldi D, Leu-Semenescu S, Yahia-Cherif L, Valabregue R, et al. Magnetic resonance imaging biomarkers to assess substantia nigra damage in idiopathic rapid eye movement sleep behavior disorder. Sleep. (2017). 40:10.1093/sleep/zsx149. doi: 10.1093/sleep/zsx149
230. Rosenmann H, Grigoriadis N, Karussis D, Boimel M, Touloumi O, Ovadia H, et al. Tauopathy-like abnormalities and neurologic deficits in mice immunized with neuronal tau protein. Arch Neurol. (2006) 63:1459–67. doi: 10.1001/archneur.63.10.1459
231. Iranzo A. Sleep and neurological autoimmune diseases. Neuropsychopharmacology. (2020) 45:129–40. doi: 10.1038/s41386-019-0463-z
232. Cosentino FI, Distefano A, Plazzi G, Schenck CH, Ferri R. A case of REM sleep behavior disorder, narcolepsy-cataplexy, parkinsonism, and rheumatoid arthritis. Behav Neurol. (2014) 2014:572931. doi: 10.1155/2014/572931
233. Schenck CH, Garcia-Rill E, Segall M, Noreen H, Mahowald MW. HLA class II genes associated with REM sleep behavior disorder. Ann Neurol. (1996) 39:261–3. doi: 10.1002/ana.410390216
234. Sabater L, Gaig C, Gelpi E, Bataller L, Lewerenz J, Torres-Vega E, et al. A novel non-rapid-eye movement and rapid-eye-movement parasomnia with sleep breathing disorder associated with antibodies to IgLON5: a case series, characterisation of the antigen, and post-mortem study. Lancet Neurol. (2014) 13:575–86. doi: 10.1016/S1474-4422(14)70051-1
235. Ganguly J, Jog M. Tauopathy and movement disorders-unveiling the chameleons and mimics. Front Neurol. (2020) 11:599384. doi: 10.3389/fneur.2020.599384
236. Landa J, Gaig C, Plagumà J, Saiz A, Antonell A, Sanchez-Valle R, et al. Effects of IgLON5 antibodies on neuronal cytoskeleton: a link between autoimmunity and neurodegeneration. Ann Neurol. (2020) 88:1023–7. doi: 10.1002/ana.25857
237. Heidbreder A, Philipp K. Anti-IgLON 5 disease. Curr Treat Options Neurol. (2018) 20:29. doi: 10.1007/s11940-018-0515-4
238. Hansen N, Hirschel S, Stöcker W, Manig A, Falk HS, Ernst M, et al. Figural memory impairment in conjunction with neuropsychiatric symptoms in IgLON5 antibody-associated autoimmune encephalitis. Front Psychiatry. (2020) 11:576. doi: 10.3389/fpsyt.2020.589466
239. Mulroy E, Jaunmuktane Z, Balint B, Erro R, Latorre A, Bhatia KP. Some new and unexpected tauopathies in movement disorders. Mov Disord Clin Pract. (2020) 7:616–26. doi: 10.1002/mdc3.12995
240. Gaig C, Graus F, Compta Y, Högl B, Bataller L, Brüggemann N, et al. Clinical manifestations of the anti-IgLON5 disease. Neurology. (2017) 88:1736–43. doi: 10.1212/WNL.0000000000003887
241. Gaig C, Iranzo A, Santamaria J, Graus F. The sleep disorder in anti-lgLON5 disease. Curr Neurol Neurosci Rep. (2018) 18:41. doi: 10.1007/s11910-018-0848-0
242. Ross RJ, Ball WA, Dinges DF, Kribbs NB, Morrison AR, Silver SM, et al. Motor dysfunction during sleep in posttraumatic stress disorder. Sleep. (1994) 17:723–32. doi: 10.1093/sleep/17.8.723
243. Elliott JE, Opel RA, Pleshakov D, Rachakonda T, Chau AQ, Weymann KB, et al. Posttraumatic stress disorder increases the odds of REM sleep behavior disorder and other parasomnias in Veterans with and without comorbid traumatic brain injury. Sleep. (2020) 43:zsz237. doi: 10.1093/sleep/zsz237
244. Mysliwiec V, O'Reilly B, Polchinski J, Kwon HP, Germain A, Roth BJ. Trauma associated sleep disorder: a proposed parasomnia encompassing disruptive nocturnal behaviors, nightmares, and REM without atonia in trauma survivors. J Clin Sleep Med. (2014) 10:1143–8. doi: 10.5664/jcsm.4120
245. Feemster JC, Smith KL, McCarter SJ, St Louis EK. Trauma-associated sleep disorder: a posttraumatic stress/REM sleep behavior disorder mash-up? J Clin Sleep Med. (2019) 15:345–9. doi: 10.5664/jcsm.7642
246. Morris LS, Tan A, Smith DA, Grehl M, Han-Huang K, Naidich TP, et al. Sub-millimeter variation in human locus coeruleus is associated with dimensional measures of psychopathology: an in vivo ultra-high field 7-Tesla MRI study. NeuroImage Clin. (2020) 25:102148. doi: 10.1016/j.nicl.2019.102148
247. Borodovitsyna O, Flamini MD, Chandler DJ. Acute stress persistently alters locus coeruleus function and anxiety-like behavior in adolescent rats. Neuroscience. (2018) 373:7–19. doi: 10.1016/j.neuroscience.2018.01.020
248. Lee E, Kim K, So HS, Choi JH, Yoon IY, Choi H. REM sleep behavior disorder among veterans with and without post-traumatic stress disorder. Psychiatry Investig. (2020) 17:987–95. doi: 10.30773/pi.2020.0213
249. Husain AM, Miller PP, Carwile ST. REM sleep behavior disorder: potential relationship to post-traumatic stress disorder. J Clin Neurophysiology. (2001) 18:148–57. doi: 10.1097/00004691-200103000-00005
250. Lavie P, Katz N, Pillar G, Zinger Y. Elevated awaking thresholds during sleep: characteristics of chronic war-related posttraumatic stress disorder patients. Biol Psychiatry. (1998) 44:1060–5. doi: 10.1016/S0006-3223(98)00037-7
251. Barone DA. Dream enactment behavior-a real nightmare: A review of post-traumatic stress disorder, REM sleep behavior disorder, and trauma-associated sleep disorder. J Clin Sleep Med. (2020) 16:1943–48. doi: 10.5664/jcsm.8758
252. Winkelman JW, James L. Serotonergic antidepressants are associated with REM sleep without atonia. Sleep. (2004) 27:317–21. doi: 10.1093/sleep/27.2.317
253. Lee K, Baron K, Soca R, Attarian H. The prevalence and characteristics of REM sleep without atonia (RSWA) in patients taking antidepressants. J Clin Sleep Med. (2016) 12:351–5. doi: 10.5664/jcsm.5582
254. Bušková J, Miletínová E, Kliková M, Bareš M, Novák T, Kosová J, et al. Associated factors of REM sleep without atonia in younger ( ≤ 50 years) hospitalized psychiatric patients. BMC Psychiatry. (2020) 20:482. doi: 10.1186/s12888-020-02879-4
255. Frauscher B, Jennum P, Ju YE, Postuma RB, Arnulf I, Cochen De Cock V, et al. Comorbidity and medication in REM sleep behavior disorder: a multicenter case-control study. Neurology. (2014) 82:1076–9. doi: 10.1212/WNL.0000000000000247
256. McCarter SJ, St Louis EK, Sandness DJ, Arndt K, Erickson M, Tabatabai G, et al. Antidepressants increase REM sleep muscle tone in patients with and without REM sleep behavior disorder. Sleep. (2015) 38:907–17. doi: 10.5665/sleep.4738
257. Dijkstra F, Viaene M, Crosiers D, De Volder I, Cras P. Frequency and characteristic features of REM sleep without atonia. Clin Neurophysiol. (2019) 130:1825–32. doi: 10.1016/j.clinph.2019.07.018
258. McGrane IR, Leung JG, St Louis EK, Boeve BF. Melatonin therapy for REM sleep behavior disorder: a critical review of evidence. Sleep Med. (2015) 16:19–26. doi: 10.1016/j.sleep.2014.09.011
259. Abdessater M, Kanbar A, Zugail AS, Al Hammadi A, Guillonneau B, Beley S. Sleep related painful erection: an algorithm for evaluation and management. Basic Clin Androl. (2019) 29:15. doi: 10.1186/s12610-019-0095-5
260. Karsenty G, Werth E, Knapp PA, Curt A, Schurch B, Bassetti CL. Sleep-related painful erections. Nat Clin Pract Urol. (2005) 2:256–61. doi: 10.1038/ncpuro0184
261. Szücs A, Janszky J, Barsi P, Erdei E, Clemens Z, Migléczi G, et al. Sleep-related painful erection is associated with neurovascular compression of basal forebrain. J Neurol. (2002) 249:486–7. doi: 10.1007/s004150200046
262. Vreugdenhil S, Weidenaar AC, de Jong IJ, van Driel MF. Sleep-related painful erections: a meta-analysis on the pathophysiology and risks and benefits of medical treatments. J Sex Med. (2018) 15:5–19. doi: 10.1016/j.jsxm.2017.11.006
263. Jones SE, Tyrrell J, Wood AR, Beaumont RN, Ruth KS, Tuke MA, et al. Genome-wide association analyses in 128,266 individuals identifies new morningness and sleep duration loci. PLoS Genet. (2016) 12:e1006125. doi: 10.1371/journal.pgen.1006125
264. Merikanto I, Kuula L, Makkonen T, Bódizs R, Halonen R, Heinonen K, et al. Publisher correction: circadian preference towards morningness is associated with lower slow sleep spindle amplitude and intensity in adolescents. Sci Rep. (2018) 8:1662. doi: 10.1038/s41598-018-20335-y
265. Didikoglu A, Maharani A, Canal MM, Pendleton N, Payton A. Interactions between season of birth, chronological age and genetic polymorphisms in determining later-life chronotype. Mech Ageing Dev. (2020) 188:111253. doi: 10.1016/j.mad.2020.111253
266. Duffy JF, Dijk DJ. Getting through to circadian oscillators: why use constant routines? J Biol Rhythms. (2002) 17:4–13. doi: 10.1177/074873002129002294
267. Merikanto I, Partonen T. Increase in eveningness and insufficient sleep among adults in population-based cross-sections from 2007 to 2017. Sleep Med. (2020) 75:368–79. doi: 10.1016/j.sleep.2020.07.046
268. Bin YS, Marshall NS, Glozier N. Secular trends in adult sleep duration: a systematic review. Sleep Med Rev. (2012) 16:223e230. doi: 10.1016/j.smrv.2011.07.003
269. Auger RR, Burgess HJ, Emens JS, Deriy LV, Thomas SM, Sharkey KM. Clinical practice guideline for the treatment of intrinsic circadian rhythm sleep-wake disorders: advanced sleep-wake phase disorder (ASWPD), delayed sleep-wake phase disorder (DSWPD), non-24-hour sleep-wake rhythm disorder (N24SWD), and irregular sleep-wake rhythm disorder (ISWRD). An Update for 2015: An American Academy of Sleep Medicine Clinical Practice Guideline. J Clin Sleep Med. (2015) 11:1199–236. doi: 10.5664/jcsm.5100
270. Duffy JF, Abbott SM, Burgess HJ, Crowley SJ, Emens JS, Epstein LJ, et al. Workshop report. Circadian rhythm sleep-wake disorders: gaps and opportunities. Sleep. (2021) 44:zsaa281. doi: 10.1093/sleep/zsaa281
271. International Labour Organization. Available online at: https://www.ilo.org/global/lang–en/index.htm
272. Kecklund G, Akerstedt T, Lowden A. Morning work: effects of early rising on sleep and alertness. Sleep. (1997) 20:215–23. doi: 10.1093/sleep/20.3.215
273. Pilcher JJ, Lambert BJ, Huffcutt AI. Differential effects of permanent and rotating shifts on self-report sleep length: a meta-analytic review. Sleep. (2000) 23:155–63. doi: 10.1093/sleep/23.2.1b
274. Garbarino S, Nobili L, Beelke M, Balestra V, Carrea P, Ferrillo F. Sonnolenza ed incidenti stradali nei turnisti della polizia stradale Italiana: uno studio sulla rete autostradale nazionale nel quinquennio 1993–1997 [Sleepiness and road accidents among policemen on shif-work on Italian highways: study of the national highway network in the period of 1993–1997]. Med Lav. (2000) 91:486–93. (Italian).
275. Richter K, Peter L, Rodenbeck A, Weess HG, Riedel-Heller SG, Hillemacher T. Shiftwork and alcohol consumption: a systematic review of the literature. Eur Addict Res. (2021) 27:9–15. doi: 10.1159/000507573
276. Wang F, Zhang L, Zhang Y, Zhang B, He Y, Xie S, et al. Meta-analysis on night shift work and risk of metabolic syndrome. Obes Rev. (2014) 15:709–20. doi: 10.1111/obr.12194
277. Proper KI, van de Langenberg D, Rodenburg W, Vermeulen RCH, van der Beek AJ, van Steeg H, et al. The relationship between shift work and metabolic risk factors: a systematic review of longitudinal studies. Am J Prev Med. (2016) 50:e147–57. doi: 10.1016/j.amepre.2015.11.013
278. Vyas MV, Garg AX, Iansavichus AV, Costella J, Donner A, Laugsand LE, et al. Shift work and vascular events: systematic review and meta-analysis. BMJ. (2012) 345:e4800. doi: 10.1136/bmj.e4800
279. Torquati L, Mielke GI, Brown WJ, Kolbe-Alexander T. Shift work and the risk of cardiovascular disease. A systematic review and meta-analysis including dose-response relationship. Scand J Work Environ Health. (2018) 44:229–38. doi: 10.5271/sjweh.3700
280. Manohar S, Thongprayoon C, Cheungpasitporn W, Mao MA, Herrmann SM. Associations of rotational shift work and night shift status with hypertension: a systematic review and meta-analysis. J Hypertens. (2017) 35:1929–37. doi: 10.1097/HJH.0000000000001442
281. Morris CJ, Purvis TE, Hu K, Scheer FA. Circadian misalignment increases cardiovascular disease risk factors in humans. Proc Natl Acad Sci USA. (2016) 113:E1402–11. doi: 10.1073/pnas.1516953113
282. Willis SK, Hatch EE, Wise LA. Sleep and female reproduction. Curr Opin Obstet Gynecol. (2019) 31:222–7. doi: 10.1097/GCO.0000000000000554
283. Mills J, Kuohung W. Impact of circadian rhythms on female reproduction and infertility treatment success. Curr Opin Endocrinol Diabetes Obes. (2019) 26:317–21. doi: 10.1097/MED.0000000000000511
284. Arnardottir ES, Nikonova EV, Shockley KR, Podtelezhnikov AA, Anafi RC, Tanis KQ, et al. Blood-gene expression reveals reduced circadian rhythmicity in individuals resistant to sleep deprivation. Sleep. (2014) 37:1589–600. doi: 10.5665/sleep.4064
285. Warby SC, Mongrain V. Resisting sleep deprivation by breaking the link between sleep and circadian rhythms. Sleep. (2014) 37:1581–2. doi: 10.5665/sleep.4056
286. Ritonja J, Aronson KJ, Matthews RW, Boivin DB, Kantermann T. Working Time Society consensus statements: individual differences in shift work tolerance and recommendations for research and practice. Ind Health. (2019) 57:201–12. doi: 10.2486/indhealth.SW-5
287. Wittmann M, Dinich J, Merrow M, Roenneberg T. Social jetlag: misalignment of biological and social time. Chronobiol Int. (2006) 23:497–509. doi: 10.1080/07420520500545979
288. Nakamura TJ, Nakamura W, Tokuda IT, Ishikawa T, Kudo T, Colwell CS, et al. Age-related changes in the circadian system unmasked by constant conditions. eNeuro. (2015) 2:ENEURO.0064-15.2015. doi: 10.1523/ENEURO.0064-15.2015
289. Van Someren EJ. Circadian and sleep disturbances in the elderly. Exp Gerontol. (2000) 35:1229–37. doi: 10.1016/S0531-5565(00)00191-1
290. Weinert H, Weinert D, Schurov I, Maywood ES, Hastings MH. Impaired expression of the mPer2 circadian clock gene in the suprachiasmatic nuclei of aging mice. Chronobiol Int. (2001) 18:559–65. doi: 10.1081/CBI-100103976
291. Musiek ES, Bhimasani M, Zangrilli MA, Morris JC, Holtzman DM, Ju YS. Circadian rest-activity pattern changes in aging and preclinical Alzheimer disease. JAMA Neurol. (2018) 75:582–90. doi: 10.1001/jamaneurol.2017.4719
292. Witting W, Kwa IH, Eikelenboom P, Mirmiran M, Swaab DF. Alterations in the circadian rest-activity rhythm in aging and Alzheimer's disease. Biol Psychiatry. (1990) 27:563–72. doi: 10.1016/0006-3223(90)90523-5
293. Musiek ES, Holtzman DM. Mechanisms linking circadian clocks, sleep, and neurodegeneration. Science. (2016) 354:1004–8. doi: 10.1126/science.aah4968
294. Gao C, Chapagain NY, Scullin MK. Sleep duration and sleep quality in caregivers of patients with dementia: a systematic review and meta-analysis. JAMA Netw Open. (2019) 2:e199891. doi: 10.1001/jamanetworkopen.2019.9891
295. Torquati L, Mielke GI, Brown WJ, Burton NW, Kolbe-Alexander TL. Shift work and poor mental health: a meta-analysis of longitudinal studies. Am J Public Health. (2019) 109:e13–20. doi: 10.2105/AJPH.2019.305278
296. Park H, Suh BS, Lee K. The difference of suicidal ideation between shift workers and day workers by gender. Arch Suicide Res. (2020) 1–9. doi: 10.1080/13811118.2020.1847708
297. D'Agostino A, Ferrara P, Terzoni S, Ostinelli EG, Carrara C, Prunas C, et al. Efficacy of triple chronotherapy in unipolar and bipolar depression: A systematic review of the available evidence. J Affect Disord. (2020) 276:297–304. doi: 10.1016/j.jad.2020.07.026
298. Quist JS, Sjödin A, Chaput J-P, Hjorth MF. Sleep and cardiometabolic risk in children and adolescents. Sleep Med Rev. (2016) 29:76–100. doi: 10.1016/j.smrv.2015.09.001
299. Cespedes Feliciano EM, Rifas-Shiman SL, Quante M, Redline S, Oken E, Taveras EM. Chronotype, social jet lag, and cardiometabolic risk factors in early adolescence. JAMA Pediatr. (2019) 173:1049–57. doi: 10.1001/jamapediatrics.2019.3089
300. Qian J, Morris CJ, Caputo R, Garaulet M, Scheer F. Ghrelin is impacted by the endogenous circadian system and by circadian misalignment in humans. Int J Obes. (2019) 43:1644–9. doi: 10.1038/s41366-018-0208-9
301. Li HX. The role of circadian clock genes in tumors. Onco Targets Ther. (2019) 12:3645–60. doi: 10.2147/OTT.S203144
302. Davis S, Mirick DK, Stevens RG. Night shift work, light at night, and risk of breast cancer. J Natl Cancer Inst. (2001) 93:1557–62. doi: 10.1093/jnci/93.20.1557
303. Samuelsson LB, Bovbjerg DH, Roecklein KA, Hall MH. Sleep and circadian disruption and incident breast cancer risk: an evidence-based and theoretical review. Neurosci Biobehav Rev. (2018) 84:35–48. doi: 10.1016/j.neubiorev.2017.10.011
304. Pitsillou E, Liang J, Hung A, Karagiannis TC. The circadian machinery links metabolic disorders and depression: a review of pathways, proteins and potential pharmacological interventions. Life Sci. (2021) 265:118809. doi: 10.1016/j.lfs.2020.118809
305. Wykes RC, Khoo HM, Caciagli L, Blumenfeld H, Golshani P, Kapur J, et al. WONOEP appraisal: network concept from an imaging perspective. Epilepsia. (2019) 60:1293–305. doi: 10.1111/epi.16067
306. Larivière S, Vos de Wael R, Paquola C, Hong SJ, Miši, ć B, et al. Microstructure-informed connectomics: enriching large-scale descriptions of healthy and diseased brains. Brain Connect. (2019) 9:113–27. doi: 10.1089/brain.2018.0587
307. Bernhardt BC, Hong S, Bernasconi A, Bernasconi N. Imaging structural and functional brain networks in temporal lobe epilepsy. Front Hum Neurosci. (2013) 7:624. doi: 10.3389/fnhum.2013.00624
308. Zheng W, Zhao Z, Zhang Z, Liu T, Zhang Y, Fan J, et al. Developmental pattern of the cortical topology in high-functioning individuals with autism spectrum disorder. Hum Brain Mapp. (2020). doi: 10.1002/hbm.25251
309. Mechelli A, Friston KJ, Frackowiak RS, Price CJ. Structural covariance in the human cortex. J Neurosci. (2005) 25:8303–10. doi: 10.1523/JNEUROSCI.0357-05.2005
310. Uddin LQ, Yeo BTT, Spreng RN. Towards a universal taxonomy of macro-scale functional human brain networks. Brain Topogr. (2019) 32:926–42. doi: 10.1007/s10548-019-00744-6
311. Bzdok D, Yeo BTT. Inference in the age of big data: future perspectives on neuroscience. Neuroimage. (2017) 155:549–64. doi: 10.1016/j.neuroimage.2017.04.061
312. Yeo BT, Krienen FM, Sepulcre J, Sabuncu MR, Lashkari D, Hollinshead M, et al. The organization of the human cerebral cortex estimated by intrinsic functional connectivity. J Neurophysiol. (2011) 106:1125–65. doi: 10.1152/jn.00338.2011
313. Hill J, Inder T, Neil J, Dierker D, Harwell J, Van Essen D. Similar patterns of cortical expansion during human development and evolution. Proc Natl Acad Sci USA. (2010) 107:13135–40. doi: 10.1073/pnas.1001229107
314. Li M, Yan J, Li S, Wang T, Wen H, Yin Y, et al. Altered gray matter volume in pri- mary insomnia patients: a DARTEL-VBM study. Brain Imag Behav. (2018) 12:1759–67. doi: 10.1007/s11682-018-9844-x
315. Joo EY, Noh HJ, Kim JS, Koo DL, Kim D, Hwang KJ, et al. (2013). Brain gray matter deficits in patients with chronic primary insomnia. Sleep. (2013) 36:999–1007. doi: 10.5665/sleep.2796
316. Rasmussen MK, Mestre H, Nedergaard M. The glymphatic pathway in neurological disorders. Lancet Neurol. (2018) 17:1016–24. doi: 10.1016/S1474-4422(18)30318-1
317. Brown BM, Rainey-Smith SR, Villemagne VL, Weinborn M, Bucks RS, Sohrabi HR. The relationship between sleep quality and brain amyloid burden. Sleep. (2016) 39:1063–8. doi: 10.5665/sleep.5756
318. Shokri-Kojori E, Wang GJ, Wiers CE, Demiral SB, Guo M, Kim SW, et al. β-Amyloid accumulation in the human brain after one night of sleep deprivation. Proc Natl Acad Sci USA. (2018) 115:4483–8. doi: 10.1073/pnas.1721694115
319. Chen DW, Wang J, Zhang LL, Wang YJ, Gao CY. Cerebrospinal fluid amyloid-β levels are increased in patients with insomnia. J Alzheimers Dis. (2018) 61:645–51. doi: 10.3233/JAD-170032
320. Winkelman JW, Plante DT, Schoerning L, Benson K, Buxton OM, O'Connor SP, et al. Increased rostral anterior cingulate cortex volume in chronic primary insomnia. Sleep. (2013) 36:991–8. doi: 10.5665/sleep.2794
321. Sadeghmousavi S, Eskian M, Rahmani F, Rezaei N. The effect of insomnia on development of Alzheimer's disease. J Neuroinflammation. (2020) 17:289. doi: 10.1186/s12974-020-01960-9
322. Tahmasian M, Noori K, Samea F, Zarei M, Spiegelhalder K, Eickhoff SB, et al. A lack of consistent brain alterations in insomnia disorder: an activation likelihood estimation meta-analysis. Sleep Med Rev. (2018) 42:111–8. doi: 10.1016/j.smrv.2018.07.004
323. Clemens S, Rye D, Hochman S. Restless legs syndrome: revisiting the dopamine hypothesis from the spinal cord perspective. Neurology. (2006) 67:125–30. doi: 10.1212/01.wnl.0000223316.53428.c9
324. Connor JR, Boyer PJ, Menzies SL, Dellinger B, Allen RP, Ondo WG, et al. Neuropathological examination suggests impaired brain iron acquisition in restless legs syndrome. Neurology. (2003) 61:304–9. doi: 10.1212/01.WNL.0000078887.16593.12
325. Moon HJ, Chang Y, Lee YS, Song HJ, Chang HW, Ku J, et al. T2 relaxometry using 3.0-tesla magnetic resonance imaging of the brain in early- and late-onset restless legs syndrome. J Clin Neurol. (2014) 10:197–202. doi: 10.3988/jcn.2014.10.3.197
326. Allen RP, Barker PB, Wehrl F, Song HK, Earley CJ. MRI measurement of brain iron in patients with restless legs syndrome. Neurology. (2001) 56:263–5. doi: 10.1212/WNL.56.2.263
327. Knake S, Heverhagen JT, Menzler K, Keil B, Oertel WH, Stiasny-Kolster K. Normal regional brain iron concentration in restless legs syndrome measured by MRI. Nat Sci Sleep. (2009) 2:19–22. doi: 10.2147/NSS.S7040
328. Boulos MI, Murray BJ, Muir RT, Gao F, Szilagyi GM, Huroy M, et al. Periodic limb movements and white matter hyperintensities in first-ever minor stroke or high-risk transient ischemic attack. Sleep. (2017) 40:zsw080. doi: 10.1093/sleep/zsw080
329. Kim JS, Lee SB, Park SK, Han SR, Kim YI, Lee KS. Periodic limb movement during sleep developed after pontine lesion. Mov Disord. (2003) 18:1403–5. doi: 10.1002/mds.10517
330. Lee PH, Lee JS, Yong SW, Huh K. Repetitive involuntary leg movements in patients with brainstem lesions involving the pontine tegmentum: Evidence for a pontine inhibitory region in humans. Parkinsonism Relat Disord. (2005) 11:105–10. doi: 10.1016/j.parkreldis.2004.09.005
331. Kang SY, Sohn YH, Lee IK, Kim JS. Unilateral periodic limb movement in sleep after supratentorial cerebral infarction. Parkinsonism Relat Disord. (2004) 10:429–31. doi: 10.1016/j.parkreldis.2004.04.009
332. Rossetti AO, Heinzer R, Espa F, Tafti M. Unilateral periodic leg movements during wakefulness and sleep after a parietal hemorrhage. Sleep Med. (2008) 9:465–6. doi: 10.1016/j.sleep.2007.06.014
333. Scheffer IE, Berkovic S, Capovilla G, Connolly MB, French J, Guilhoto L, et al. ILAE classification of the epilepsies: Position paper of the ILAE Commission for Classification and Terminology. Epilepsia. (2017) 58:512–21. doi: 10.1111/epi.13709
334. Gibbs SA, Proserpio P, Terzaghi M, Pigorini A, Sarasso S, Lo Russo G, et al. Sleep-related epileptic behaviors and non-REM-related parasomnias: insights from stereo-EEG. Sleep Med Rev. (2016) 25:4–20. doi: 10.1016/j.smrv.2015.05.002
335. Naldi I, Bisulli F, Testa C, Rizzo G, Ferri L, Gramegna LL, et al. Proton MR spectroscopy in patients with sleep-related hypermotor epilepsy (SHE): evidence of altered cingulate cortex metabolism. Sleep. (2017) 40. doi: 10.1093/sleep/zsx115
336. Petroff OA, Errante LD, Kim JH, Spencer DD. N-acetyl-aspartate, total creatine, and myo-inositol in the epileptogenic human hippocampus. Neurology. (2003) 60:1646–51. doi: 10.1212/01.WNL.0000068020.85450.8B
337. Daida K, Ogaki K, Hayashida A, Ando M, Yokoyama K, Noda K, et al. Somnolence preceded the development of a subthalamic lesion in neuromyelitis optica spectrum disorder. Intern Med. (2020) 59:577–9. doi: 10.2169/internalmedicine.2947-19
338. Matsubara T, Suzuki K, Kawasaki A, Miyamoto M, Okamura M, Kanbayashi T, et al. Sudden onset of sleep caused by hypothalamic infarction: a case report. BMC Neurol. (2019) 19:182. doi: 10.1186/s12883-019-1414-3
339. Trotti LM, Bliwise DL. Brain MRI findings in patients with idiopathic hypersomnia. Clin Neurol Neurosurg. (2017) 157:19–21. doi: 10.1016/j.clineuro.2017.03.010
340. van den Heuvel MP, Hulshoff Pol HE. Exploring the brain network: a review on resting-state fMRI functional connectivity. Eur Neuropsychopharmacol. (2010) 20:519–34. doi: 10.1016/j.euroneuro.2010.03.008
341. Pomares FB, Boucetta S, Lachapelle F, Steffener J, Montplaisir J, Cha J, et al. Beyond sleepy: structural and functional changes of the default-mode network in idiopathic hypersomnia. Sleep. (2019) 42:zsz156. doi: 10.1093/sleep/zsz156
342. Boucetta S, Montplaisir J, Zadra A, Lachapelle F, Soucy JP, Gravel P, Dang-Vu TT. Altered regional cerebral blood flow in idiopathic hypersomnia. Sleep. (2017) 40. doi: 10.1093/sleep/zsx140
343. Carvalho DZ, St Louis EK, Knopman DS, Boeve BF, Lowe VJ, Roberts RO, et al. Association of excessive daytime sleepiness with longitudinal β-amyloid accumulation in elderly persons without dementia. JAMA Neurol. (2018) 75:672–80. doi: 10.1001/jamaneurol.2018.0049
344. Ward AM, McLaren DG, Schultz AP, Chhatwal J, Boot BP, Hedden T, et al. Daytime sleepiness is associated with decreased default mode network connectivity in both young and cognitively intact elderly subjects. Sleep. (2013) 36:1609–15. doi: 10.5665/sleep.3108
345. Xiao F, Lu C, Zhao D, Zou Q, Xu L, Li J, et al. Independent component analysis and graph theoretical analysis in patients with narcolepsy. Neurosci Bull. (2019) 35:743–55. doi: 10.1007/s12264-018-0307-6
346. Gusnard DA, Raichle ME, Raichle ME. Searching for a baseline: functional imaging and the resting human brain. Nat Rev Neurosci. (2001) 2:685–94. doi: 10.1038/35094500
347. Schiel JE, Holub F, Petri R, Leerssen J, Tamm S, Tahmasian M, et al. Affect and Arousal in Insomnia: through a Lens of Neuroimaging Studies. Curr Psychiatry Rep. (2020) 22:44. doi: 10.1007/s11920-020-01173-0
348. Tarun A, Wainstein-Andriano D, Sterpenich V, Bayer L, Perogamvros L, Solms M, et al. NREM sleep stages specifically alter dynamical integration of large-scale brain networks. iScience. (2020) 24:101923. doi: 10.1101/2020.07.08.193508
349. Vallat R, Nicolas A, Ruby P. Brain functional connectivity upon awakening from sleep predicts interindividual differences in dream recall frequency. Sleep. (2020) 43:zsaa116. doi: 10.1093/sleep/zsaa116
350. Mano N. Changes of simple and complex spike activity of cerebellar purkinje cells with sleep and waking. Science. (1970) 170:1325. doi: 10.1126/science.170.3964.1325
351. Steriade M, Apostol V, Oakson G. Control of unitary activities in cerebellothalamic pathway during wakefulness and synchronized sleep. J Neurophysiol. (1971) 34:389–413. doi: 10.1152/jn.1971.34.3.389
352. Cirelli C, Gutierrez CM, Tononi G. Extensive and divergent effects of sleep and wakefulness on brain gene expression. Neuron. (2004) 41:35–43. doi: 10.1016/S0896-6273(03)00814-6
353. Schabus M, Dang-Vu TT, Albouy G, Balteau E, Boly M, Carrier J, et al. Hemodynamic cerebral correlates of sleep spindles during human non-rapid eye movement sleep. Proc Natl Acad Sci USA. (2007) 104:13164–9. doi: 10.1073/pnas.0703084104
354. Xu W, De Carvalho F, Clarke AK, Jackson A. Communication from the cerebellum to the neocortex during sleep spindles. Prog Neurobiol. (2020) 101940. doi: 10.1016/j.pneurobio.2020.101940
355. DelRosso LM, Hoque R. The cerebellum and sleep. Neurol Clin. (2014) 32:893–900. doi: 10.1016/j.ncl.2014.07.003
356. Hanyu H, Inoue Y, Sakurai H, Kanetaka H, Nakamura M, Miyamoto T, et al. Voxel-based magnetic resonance imaging study of structural brain changes in patients with idiopathic REM sleep behavior disorder. Parkinson Relat Disord. (2012) 18:136–9. doi: 10.1016/j.parkreldis.2011.08.023
357. Chen M, Li Y, Chen J, Gao L, Sun J, Gu Z, et al. Structural and functional brain alterations in patients with idiopathic rapid eye movement sleep behavior disorder. J neuroradiol. (2022) 49:66–72. doi: 10.1016/j.neurad.2020.04.007
358. Muthukumaraswamy SD. High-frequency brain activity and muscle artifacts in MEG/EEG: a review and recommenda- tions. Front Hum Neurosci. (2013) 7:138. doi: 10.3389/fnhum.2013.00138
359. Penner CG, Gerardy B, Ryan R, Williams M. The odds ratio product (an objective sleep depth measure): normal values, repeatability, and change with CPAP in patients with OSA. J Clin Sleep Med. (2019) 15:1155–63. doi: 10.5664/jcsm.7812
360. Younes M, Ostrowski M, Soiferman M, Younes H, Younes M, Raneri J, et al. Odds ratio product of sleep EEG as a continuous measure of sleep state. Sleep. (2015) 38:641–54. doi: 10.5665/sleep.4588
361. Parrino L, Grassi A, Milioli G. Cyclic alternating pattern in polysomnography: what is it and what does it mean? Curr Opin Pulm Med. (2014) 20:533–41. doi: 10.1097/MCP.0000000000000100
362. Thomas RJ. Alternative approaches to treatment of Central Sleep Apnea. Sleep Med Clin. (2014) 9:87–104. doi: 10.1016/j.jsmc.2013.10.008
363. Amatoury J, Azarbarzin A, Younes M, Jordan AS, Wellman A, Eckert DJ. Arousal intensity is a distinct pathophysiological trait in obstructive sleep apnea. Sleep. (2016) 39:2091–100. doi: 10.5665/sleep.6304
364. Azarbarzin A, Ostrowski M, Moussavi Z, Hanly P, Younes M. Contribution of arousal from sleep to postevent tachycardia in patients with obstructive sleep apnea. Sleep. (2013) 36:881–9. doi: 10.5665/sleep.2716
365. Eckert DJ, Younes MK. Arousal from sleep: implications for obstructive sleep apnea pathogenesis and treatment. J Appl Physiol (1985). (2014) 116:302–13. doi: 10.1152/japplphysiol.00649.2013
366. Gilmartin GS, Thomas RJ. Mechanisms of arousal from sleep and their consequences. Curr Opin Pulm Med. (2004) 10:468–74. doi: 10.1097/01.mcp.0000143690.94442.b3
367. Thomas RJ. Arousals in sleep-disordered breathing: patterns and implications. Sleep. (2003) 26:1042–7. doi: 10.1093/sleep/26.8.1042
368. Prerau MJ, Brown RE, Bianchi MT, Ellenbogen JM, Purdon PL. Sleep neurophysiological dynamics through the lens of multitaper spectral analysis. Physiology. (2017) 32:60–92. doi: 10.1152/physiol.00062.2015
369. Sleepimage. Sleep Quality and Sleep Disorders. Available online at: https://sleepimage.com/
370. Al Ashry HS, Hilmisson H, Ni Y, Thomas RJ; APPLES Investigators. Automated apnea-hypopnea index from oximetry and spectral analysis of cardiopulmonary coupling. Ann Am Thorac Soc. (2021) 18:876–83. doi: 10.1513/AnnalsATS.202005-510OC
371. Thomas RJ, Mietus JE, Peng CK, Goldberger AL. An electrocardiogram-based technique to assess cardiopulmonary coupling during sleep. Sleep. (2005) 28:1151–61. doi: 10.1093/sleep/28.9.1151
372. Thomas RJ, Mietus JE, Peng CK, Guo D, Gozal D, Montgomery-Downs H, et al. Relationship between delta power and the electrocardiogram-derived cardiopulmonary spectrogram: Possible implications for assessing the effectiveness of sleep. Sleep Med. (2014) 15:125–31. doi: 10.1016/j.sleep.2013.10.002
373. Wood C, Bianchi MT, Yun CH, Shin C, Thomas RJ. Multicomponent analysis of sleep using electrocortical, respiratory, autonomic and hemodynamic signals reveals distinct features of stable and unstable NREM and REM sleep. Front Physiol. (2020) 11:592978. doi: 10.3389/fphys.2020.592978
374. Thomas RJ, Mietus JE, Peng CK, Gilmartin G, Daly RW, Goldberger AL, et al. Differentiating obstructive from central and complex sleep apnea using an automated electrocardiogram-based method. Sleep. (2007) 30:1756–69. doi: 10.1093/sleep/30.12.1756
375. Choi JH, Thomas RJ, Suh SY, Park IH, Kim TH, Lee SH, et al. Sleep quality change after upper airway surgery in obstructive sleep apnea: Electrocardiogram-based cardiopulmonary coupling analysis. Laryngoscope. (2015) 125:1737–42. doi: 10.1002/lary.25101
376. Hilmisson H, Sveinsdottir E, Lange N, Magnusdottir S. Insomnia symptoms in primary care: A prospective study focusing on prevalence of undiagnosed co-morbid sleep disordered breathing. Eur J Intern Med. (2019) 63:19–26. doi: 10.1016/j.ejim.2019.01.011
377. Yang AC, Yang CH, Hong CJ, Tsai SJ, Kuo CH, Peng CK, et al. Sleep state instabilities in major depressive disorder: detection and quantification with electrocardiogram-based cardiopulmonary coupling analysis. Psychophysiology. (2011) 48:285–91. doi: 10.1111/j.1469-8986.2010.01060.x
378. Thomas RJ, Mietus JE, Peng CK, Goldberger AL, Crofford LJ, Chervin RD. Impaired sleep quality in fibromyalgia: detection and quantification with ECG-based cardiopulmonary coupling spectrograms. Sleep Med. (2010) 11:497–8. doi: 10.1016/j.sleep.2009.09.003
379. Thomas RJ, Weiss MD, Mietus JE, Peng CK, Goldberger AL, Gottlieb DJ. Prevalent hypertension and stroke in the Sleep Heart Health Study: association with an ECG-derived spectrographic marker of cardiopulmonary coupling. Sleep. (2009) 32:897–904.
380. Kim W, Na JO, Thomas RJ, Jang WY, Kang DO, Park Y, et al. Impact of catheter ablation on sleep quality and relationship between sleep stability and recurrence of paroxysmal atrial fibrillation after successful ablation: 24-hour holter-based cardiopulmonary coupling analysis. J Am Heart Assoc. (2020) 9:e017016. doi: 10.1161/JAHA.120.017016
381. Pogach MS, Punjabi NM, Thomas N, Thomas RJ. Electrocardiogram-based sleep spectrogram measures of sleep stability and glucose disposal in sleep disordered breathing. Sleep. (2012) 35:139–48. doi: 10.5665/sleep.1604
382. Magnusdottir S, Hilmisson H, Thomas RJ. Cardiopulmonary coupling-derived sleep quality is associated with improvements in blood pressure in patients with obstructive sleep apnea at high-cardiovascular risk. J Hypertens. (2020) 38:2287–94. doi: 10.1097/HJH.0000000000002553
383. Hilmisson H, Berman S, Magnusdottir S. Sleep apnea diagnosis in children using software-generated apnea-hypopnea index (AHI) derived from data recorded with a single photoplethysmogram sensor (PPG) : Results from the Childhood Adenotonsillectomy Study (CHAT) based on cardiopulmonary coupling analysis. Sleep Breath. (2020) 24:1739–49. doi: 10.1007/s11325-020-02049-6
384. Hilmisson H, Lange N, Magnusdottir S. Objective sleep quality and metabolic risk in healthy weight children results from the randomized Childhood Adenotonsillectomy Trial (CHAT). Sleep Breath. (2019) 23:1197–208. doi: 10.1007/s11325-019-01802-w
385. Jordan AS, White DP, Lo YL, Wellman A, Eckert DJ, Yim-Yeh S, et al. Airway dilator muscle activity and lung volume during stable breathing in obstructive sleep apnea. Sleep. (2009) 32:361–8. doi: 10.1093/sleep/32.3.361
386. Chervin RD, Burns JW, Subotic NS, Roussi C, Thelen B, Ruzicka DL. Method for detection of respiratory cycle-related EEG changes in sleep-disordered breathing. Sleep. (2004) 27:110–5. doi: 10.1093/sleep/27.1.110
387. Chervin RD, Shelgikar AV, Burns JW. Respiratory cycle-related EEG changes: response to CPAP. Sleep. (2012) 35:203–9. doi: 10.5665/sleep.1622
388. Azarbarzin A, Ostrowski M, Younes M, Keenan BT, Pack AI, Staley B, et al. Arousal responses during overnight polysomnography and their reproducibility in healthy young adults. Sleep. (2015) 38:1313–21. doi: 10.5665/sleep.4916
389. Younes M, Giannouli E. Mechanism of excessive wake time when associated with obstructive sleep apnea or periodic limb movements. J Clin Sleep Med. (2020) 16:389–99. doi: 10.5664/jcsm.8214
390. Younes M, Hanly PJ. Immediate postarousal sleep dynamics: an important determinant of sleep stability in obstructive sleep apnea. J Appl Physiol (1985). (2016) 120:801–8. doi: 10.1152/japplphysiol.00880.2015
Glossary
(N)REM sleep, (non) rapid eye movements sleep; AASM, American Academy of Sleep Medicine; Ab, beta-amyloid; AD, Alzheimer's disease; AHI, apnea–hypopnea index; ALS, amyotrophic lateral sclerosis; ASWPD, sleep-wake phase disorder; BMI, Body max Index; BOLD, blood-oxygen-level-dependent; CAP, Cyclic Alternating Pattern; CBT, Cognitive behavioral therapy for insomnia; CBT-min, core body temperature minimum; CNS, central nervous system; COMISA, Comorbid Insomnia and Sleep Apnea; COPD, chronic obstructive pulmonary disease; CoSA, Comorbidities of Sleep Apnea; CPC, cardiopulmonary coupling; CRSWD, Circadian sleep-wake disorders; CSF, cerebro-spinal fluid; DLB, diffuse Lewy body disease; DLMO, light melatonin onset; DMN, default mode network; DOA, Disorders of arousal; DSWPD, delayed sleep-wake phase disorder; DTI, diffusion tensor imaging; EDS, excessive daytime sleepiness; EEG, electroencephalogram; ESADA, Sleep Apnea Network/European Sleep Apnea Database; fMRI, functional MRI; GABA gamma-aminobutyric acid; GP, general practitionerice; HD, Huntington's disease; HLA, human leucocyte antigen; HRV, Heart rate variability; IARC, The International Agency for Research on Cancer; IEDs, interictal epileptiform discharges; IFL, inspiratory flow limitation; IH, idiopathic hypersomnia; ILAE, International League Against Epilepsy; ILO, International Labor Organization; IMI, intermovement interval; ISI, insomnia severity index; ISWRD, irregular sleep-wake rhythm disorder; LMs, Leg Movements; MCDs, malformations of cortical development; MRI, magnetic resonance imaging; MSA, multisystemic atrophy; N24SWD, non-24-hour sleep-wake rhythm disorder; nAchR, nicotinic acetylcholine receptor; ORP, Odds Ratio Product; OSA, obstructive sleep apnea; PAP, Positive Airway Pressure; PC, pre-coeruleus region; Pcrit, Pharyngeal critical closing pressure; PD, Parkinson's disease; PER2, Period Circadian Regulator 2; PI, Periodicity Index; PLMD, periodic leg movement disorder; PPG, pulse photoplethysmogram; PSP, progressive supranuclear palsy; PTSD, post-traumatic stress disorder; PWA, pulse wave amplitude; RBD, REM sleep Behavior Disorder; REM_rapid eye movement; RLS, restless leg syndrome; RSD, restless sleep disorder; RSN, resting state networks; RSWA, REM sleep without atonia; SHE, Sleep related hypermotor epilepsy; SLD, sublatero-dorsal nucleus; SNRI, selective norepinephrine reuptake inhibitors; SSRI, selective serotonin reuptake inhibitors; SWS, slow wave sleep; TASD, trauma-associated sleep disorder; TDP, TAR DNA binding protein; UARS, upper airway resistance syndrome; WASM, Word Association of Sleep Medicine.
Keywords: sleep, sleep disorders, sleep medicine, sleep diseases, sleep medicine evolution
Citation: Parrino L, Halasz P, Szucs A, Thomas RJ, Azzi N, Rausa F, Pizzarotti S, Zilioli A, Misirocchi F and Mutti C (2022) Sleep medicine: Practice, challenges and new frontiers. Front. Neurol. 13:966659. doi: 10.3389/fneur.2022.966659
Received: 11 June 2022; Accepted: 29 August 2022;
Published: 14 October 2022.
Edited by:
Smaranda Leu-Semenescu, Pitie-Salpetriere Hospital, FranceReviewed by:
Gilles Lavigne, Université de Montréal, CanadaFederica Provini, University of Bologna, Italy
Copyright © 2022 Parrino, Halasz, Szucs, Thomas, Azzi, Rausa, Pizzarotti, Zilioli, Misirocchi and Mutti. This is an open-access article distributed under the terms of the Creative Commons Attribution License (CC BY). The use, distribution or reproduction in other forums is permitted, provided the original author(s) and the copyright owner(s) are credited and that the original publication in this journal is cited, in accordance with accepted academic practice. No use, distribution or reproduction is permitted which does not comply with these terms.
*Correspondence: Liborio Parrino, liborio.parrino@unipr.it