- 1Centre for Interdisciplinary Research in Basic Sciences, Jamia Millia Islamia, New Delhi, India
- 2Department of Biosciences, Jamia Millia Islamia, New Delhi, India
- 3Department of Clinical Laboratory Science, College of Applied Sciences-Qurayyat, Jouf University, Sakakah, Saudi Arabia
- 4Department of Biology, College of Science, University of Hail, Hail, Saudi Arabia
- 5Department of Biotechnology, Jamia Millia Islamia, New Delhi, India
- 6Department of Biomedical Sciences and Therapeutics, Faculty of Medicine & Health Sciences, University Malaysia Sabah, Kota Kinabalu, Malaysia
- 7Department of Biochemistry, Faculty of Medicine and Health Sciences, Abdurrab University, Pekanbaru, Indonesia
- 8Centre for International Collaboration and Research, Reva University, Rukmini Knowledge Park, Kattigenahalli, Bangalore, India
Caffeic acid (CA) is found abundantly in fruits, vegetables, tea, coffee, oils, and more. CA and its derivatives have been used for many centuries due to their natural healing and medicinal properties. CA possesses various biological and pharmacological activities, including antioxidant, anti-inflammatory, anticancer, and neuroprotective effects. The potential therapeutic effects of CA are mediated via repression and inhibition of transcription and growth factors. CA possesses potential anticancer and neuroprotective effects in human cell cultures and animal models. However, the biomolecular interactions and pathways of CA have been described highlighting the target binding proteins and signaling molecules. The current review focuses on CA’s chemical, physical, and pharmacological properties, including antioxidant, anti-inflammatory, anticancer, and neuroprotective effects. We further described CA’s characteristics and therapeutic potential and its future directions.
Introduction
Caffeic acid (CA), one of the most common phenolic acids (PhA), frequently occurs in fruits (1), grains (2), and dietary supplements (3) for human consumption as simple esters with quinic acid or saccharides. It is found in fruits, tea, coffee, oil, spices, and vegetables. CA is isolated and purified from green and roasted coffee sources. Food coming directly from plants is a rich source of phytochemicals. They are considered biologically active compounds, though with negligible nutritional values. Studies reveal their major role in chronic disease prevention. These plant compounds are antioxidants, pharmacological agents, detoxifying/cleansing agents, and dietary fiber (4, 5). CA (3,4-dihydroxycinnamic acid) is a phytochemical that prevents several human diseases, and it is manufactured through the hydrolysis of chlorogenic acid (6, 7).
CA illustrated a wide range of chemical and pharmacological properties (4). It is orally bioavailable due to its anti-inflammatory, antioxidant, and anticancer bioactivities (8–10) and immunomodulatory and neuroprotective activities (11–13). CA has antitumor effects against several human cancers (14, 15). It is an excellent antioxidant and anti-inflammatory agent. CA exhibits the medicinal properties of reducing oxidative stress and significantly inhibits damage to DNA by free radicals (16, 17). CA is known for preventing cancer cell growth by inhibiting the HDM histone demethylase oncoprotein gene during cancer progression (18), and explains neuroprotective results against amyloid-β–mediated neurotoxicity via blocking calcium influx and tau phosphorylation. It may contribute to preventing neurodegeneration and brain injury (19, 20).
CA has established its importance as an effective 5-lipooxygenase inhibitor and has revealed its capacity for downregulating IL-6, IL-1β, and NF-κB in the inflammatory response (21, 22). CA drastically blocks STAT3 action and this, in turn, down-triggers HIF-1α action. It has the promising inhibitors of STAT3 and represses cancer angiogenesis via blocking the action of STAT3 and the expression of VEGF and HIF-1α (14). CA blocked ERKs phosphorylation, NF-κB, and AP-1 transactivation. However, CA targets MEK1 and TOPK for repressing tumor metastasis and neoplastic cell transformation (15). It frankly interrelated with ERK1/2 and blocked ERK1/2 actions in vitro (23).
This review focuses on the effects of CA, such as antioxidant, anti-inflammatory, anticancer, and neuroprotective effects. The potential of CA in cancer and neurological diseases with emphasis on clinical trials has been summarized. This review also describes CA’s features and therapeutic potential and its implications in cancer and neurological disease treatment and management.
Biosynthesis of Phenolic Acids
Higher plants accumulate and synthesize a broad diversity of phenolic compounds that confer defense against the assaults of free radicals created through the process of photosynthesis, as well as against tissue damages (24). Phenolic compounds offer defense against diseases by regulating cellular mechanisms at different levels, such as enzyme inhibition, protein phosphorylation, and alteration of gene expression (25). Phenolics are essential ingredients in plants, commonly found in fruits, cereals, legumes, and vegetables. In plants, they have a role in a broad range of processes, including growth, pigmentation, reproduction, as well as resistance to pathogens. They are categorized into five classes: flavonoids, coumarins, tannins, phenolic acids, and stilbenes (26, 27). However, phenolics are secondary metabolites primarily created in plants from shikimic acid via phenylpropanoid signaling (26). They are created as an effect of the break of lignin and cell wall polymers in vascular plants and the like via the production of monolignol signaling (26, 27). PhA are the furthermore classified into cinnamic acids and benzoic acids. They are present in free appearances or conjugated with ethers, esters, as well as other molecules (26–29). The structural motifs needed for the antitumor action of phenolic compounds comprise the aromatic ring, unsaturated, substituted chains, and the position and number of free hydroxyl groups (26). Phenolics are admired for their effectiveness as medicinal compounds in the treatment and management of numerous diseases, including cancer and neurodegenerative diseases. Their antidiabetic function is induced by the modulation of glucose metabolism (27, 30).
Chemical and Physical Properties of Caffeic Acid
The defensive effect of CA on the human body is described because of its antioxidant functions, which are endorsed to its chemical structure. However, the antioxidant functions of CA are linked with the existence of two hydroxyl groups at its aromatic ring. The structure of CA has a phenol ring with OH at positions 3 and 4 of the ring and a hydrocarbon chain at position 1 with an acid group. The chemical structure characteristics of CA make it an efficient metal-reducing agent. It is prone to auto-oxidation and oxidation by biological agents. The catechol group with an unsaturated carboxylic acid chain interacts with the oxidizing radicals. Chemically, it is cinnamic acid. Its chemical structure comprises a phenyl ring with hydroxyl groups at the 3rd and 4th positions. More commonly in transform, the cis form also exists (31). Thus, ultimately this structural uniqueness imparts anticancer properties (32, 33).
The CA derivative CAPE has a similar structure but with an additional phenylmethyl ester group. However, the chemical and physical properties of the two vary (34, 35). CA is a phenolic compound consequential from the secondary metabolism of plant results (36, 37). For example, olives, fruits, potatoes, coffee beans, carrots, and propolis are the major hydroxycinnamic acid utilized in human diets (38–40). CA is present in yellow crystals that turn alkaline solution from yellow to orange. It is sparingly soluble in cold water and highly soluble in hot water and cold alcohol. It has a small weighted compound with zero formal charges (41, 42).
Bioavailability and Pharmacokinetics of Caffeic Acid
CA is absorbed in the GI tract by MA transporters (43, 44) and by the transepithelial flux to a slighter extent (45). Gut microbiota is linked to the metabolism of CA. Actually, under an anaerobic situation, CA undertakes decarboxylation that is passed out through the bacteria with the analogous product [3-(3- hydroxyphenyl)-propionic acid] exhibiting better antioxidant action in contrast to CA (46). Once absorbed, CA undertakes widespread metabolic transformations in the kidneys and liver (47, 48). The small intestine is the probable location of the cleavage of feruoylquinic acids liberating CA and ferulic acid, the metabolism of CA to its 3- and 4-O-sulfates, and the methylation of CA for forming isoferulic acid and its corresponding 3-O-sulfation and glucuronidation, since the colon is possibly the location of the metabolism of CA to dihydrocaffeic acid that is promoted to be metabolized for dihydro-isoferulic acid. CA is emitted mainly by the urine, which calculates urinary secretion between 5.9% and 27% (32, 49, 50). Accordingly, the pharmacokinetic procedure starts with the ingestion of CA, inward in the stomach, following a little part that is absorbed. Hence, in the colon, the microbial esterases slice the ester piece of CA; in its free appearance, it is absorbed through the intestinal mucosa (51). The transmembrane run of CA into intestinal cells takes place via active transport mediated through the MCT (51).
The highest plasma concentration of the compound has been detected merely 1 h after ingestion of foods, including coffee. Subsequently, plasma concentration is quickly reduced, requiring reiterated doses every 2 h to sustain high concentrations (52–54). Chemical flexibility and modifiability of CA affected its phenylpropanoid scaffold for becoming a usually utilized pattern for improving novel derivatives with increased pharmacokinetic functions, enhanced bioactivity, and superior safety summary (55). However, studies have shown that hepatic metabolism of CA and DHCA occurs when O-methylation, dehydrogenation, hydrogenation, and GSH conjugation take place. Detoxification for CA and DHCA comes from the O-methylation pathway, whereas the toxification occurs via P450 catalyzation to form o-quinones (56, 57). Humans have CA metabolites, mainly glucuronides of meta-coumaric acid and meta-hydroxyhippuric acid. The derivative of CA, CAPE, synthesized by combining CA and phenethyl alcohol in the ratio of 1:5 at room temperature along with the condensing agent DCC, has been proven to have therapeutic potential (35, 58). CAPE arrests the growth of HL-60 cells in leukemia. Different IC50 values of 1.0, 0.5, and 1.5 µM inhibit the DNA, RNA, and protein expression, respectively (59).
Biological Mechanism of Action of Caffeic Acid
Oxidative Stress
Reactive oxygen species (ROS) are linked with numerous cellular functions, including cell differentiation, proliferation, angiogenesis, and apoptosis. ROS are the main signaling molecule that participates in an essential function in developing inflammatory diseases such as cancer and neurodegenerative diseases. Oxidative stress and chronic inflammation are the most crucial factors engaged in the progression of about 15–20% of cancers worldwide (60). The constant inflammatory cell employment, frequent production of ROS, and pro-inflammatory mediators, which persisted the proliferation of genomically unbalanced cells, help the neoplastic transformation and eventually result in cancer metastasis and invasion (61). The inequity between the production and clearance of ROS/RNS aids the progression of the tumor chiefly via stimulating genomic instability. CA blocks lipoxygenase action and represses LPO (62, 63). CA entirely inhibits ROS production and the xanthine oxidase system (62). CA might enhance the cytotoxic activities of M1 macrophages and block cancer growth; inhibitory action on TAMs can be induced by its antioxidative action. However, the anticancer action of CA has been the effect of the synergistic actions of diverse mechanisms through which CA performs on proliferation, survival, angiogenesis, and immunomodulation (64–66). CA competently inhibited the incidence of TAMs and noticeably repressed tumorigenesis in mouse tumor models. Hence, targeting TAMs via antioxidants might be a potentially effective method for tumor treatment (64).
The amount of such ROS-mediated injuries was revealed to be more widespread in the areas of the brain where Aβ is rich (67, 68). The antioxidant defense in the AD brain was found to repeatedly reduce as the disease progressed (69–71). Mitochondrial dysfunction is the main cause of ROS-induced pathologies far too frequent in AD (72). Thus, drugs targeting several mechanisms of ROS/oxidative stress-induced cellular injuries could have therapeutic potential for AD. Hence, a study (73) has examined the anti-AD potential of CA in an in vitro acrolein-mediated toxicity model utilizing HT22 mouse hippocampal cells. However, the study has revealed that pretreatment of cells with CA drastically reduced the acrolein-mediated neurotoxicity, ROS gathering, and GSH reduction. The acrolein-mediated MAPKs and GSK3β/Akt signalings have been altered via CA, recommending their probable mechanisms by such results. The improvement of memory via CA (10–50 mg/kg) in the global cerebral ischemia-reperfusion damage in the rat is partly because of antioxidant results, as a reduced MDA and considerable SOD activities have been detected to be reduced in the hippocampus’s subsequent treatment with CA (22). The defensive result of CA against spatial learning and memory role failure via chronic aluminum excess has been revealed to be accompanied by the reverse of the reduced SOD actions and enhanced MDA contents (21). However, the association between cognitive decline and oxidative stress is fine as demonstrated in most of the work by researchers (74) who utilized streptozotocin-mediated investigational dementia in rats like an AD model.
Antioxidant Properties of Caffeic Acid
Antioxidants perform by blocking or decreasing the results regulated via free radicals and oxidizing compounds (75). The phenolic acids are distinguished via the existence of a benzene ring, a carboxylic acid group, and one/more hydroxyl/methoxy groups of the molecule that confers antioxidant functions (76). CA has been revealed for producing defensive results on α-tocopherol in low-density lipoprotein (77, 78). CA is an antioxidant, which may decrease oxidative stress made in the body because of free radicals. Hence, oxidative stress is described as an inequity between the making of ROS and antioxidant protection (79, 80).
In consequence of this inequity, oxidative stress is frequently responded with the progression of several human diseases including cancer (81, 82). Antioxidants perform by inhibiting the effects regulated via free radicals and oxidizing compounds (75). CA was found to be an α-tocopherol defensive in LDL (77). Additionally, its grouping with other products, including chlorogenic and caftaric acids, explained high potent antioxidant action in various systems (83, 84). CA is among the hydroxycinnamates used to increase dietary products’ constancy (85, 86). The antioxidant promise of CA is influenced by factors including the nature of oil, food processing, constituents, and the ratio of antioxidants and lipid constituents (86, 87). However, novel dihydro-CA amides were proven to have brilliant free radical scavenging capabilities accessed via the DPPH technique; an outstanding potential was identified for protecting polyunsaturated oils (88). The addition of CA was documented to increase the solidity of different oils via the blockage of lipid oxidation (85, 87, 89).
UV radiation is the reason for biological alterations, including photoaging, and injures normal lymphocytes, consequential in their death. CA achieves notable consideration as a potential photoprotective agent (90) and also is present in skincare products due to its antioxidant action. Depending on the exposure time, dose, wavelength, and area exposed, the UV radiation may cause premature skin aging, skin burns, skin cell DNA injury, and skin tumor (91, 92). Though lymphocytes were pretreated with CA for 30 minutes while being irradiated with UVB, a photoprotective result of CA was identified in terms of reduced LPO and decreased DNA injury illustrating the viability of lymphocytes (93). Hence, the photoprotective result of CA might be because of GSH metabolism, which displays free radical scavenging. CA was detected for protecting phospholipidic membranes (94, 95) and opposed vitamins C and E depletion by the membrane (96). CA was found to have photoprotective action against UVB both in vitro and in vivo (97, 98). CA pretreatment causes a significant reduction in g radiation-motivated DNA injury in cultured lymphocytes (97). However, ischemia/reperfusion (I/R) damage may be caused by ROS (99). Reported models might be used to study defensive results of antioxidants administered before the induction of I/R damage (100, 101). CA was proven to have defensive capabilities against I/R injury in rats’ small intestine (99, 102, 103).
Anti-Inflammatory Properties of Caffeic Acid
In response to a stimulus including tissue injury, inflammation expands. It is a physiologic process that might contribute to cancer progression via several intermediates (104). Many modulators involve inflammation to tumor progression, including NF-kB, COX-2, TNF-a, IL-6, Nrf2, iNOS, NFAT, and HIF-1α (104–106). CA illustrated its inhibitory action on NO-making that also sturdily blocked the creation of COX -2 and iNOS (107). Therapeutic agents that target NF-κB and COX-2 can help repress cancer angiogenesis (60, 64, 108, 109). CA powerfully hinders ceramide-mediated NF-κB action (110) and UVB-mediated COX-2 expression (109, 111). Hence, numerous studies have recognized CA to be an inducer of cell death in tumor cells and to be able to perform cancer growth blockage and failure in animals (112–114). The role of CA in inflammation, cancer progression, and other pathways is illustrated in Figure 1.
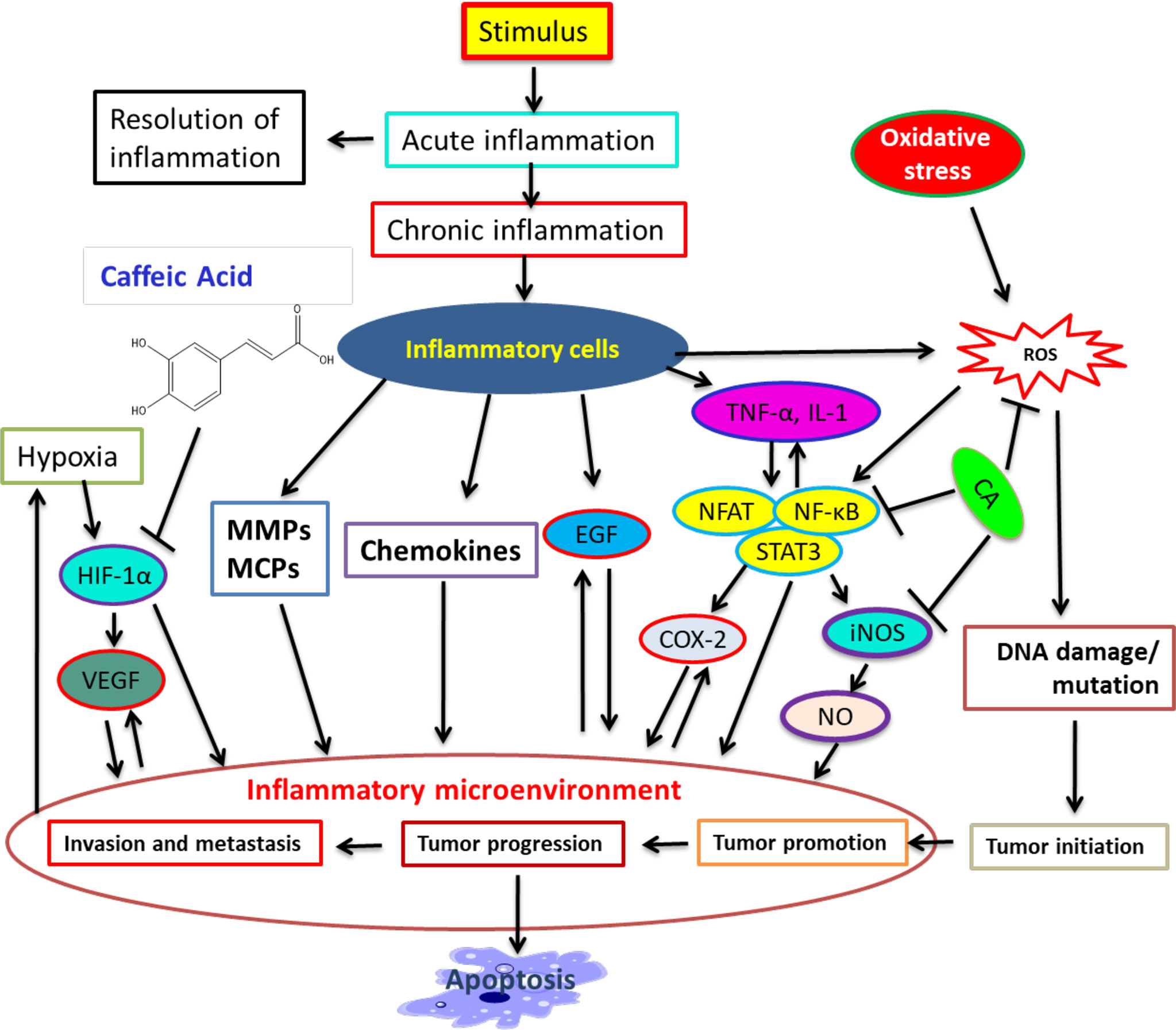
Figure 1 A summary of connection among inflammation, cancer progression, and inhibitory action of CA. Many modulators involve inflammation in tumor progression. CA binds with modulators and represses cancer progression and stimulates apoptosis. [Adapted from Murtaza et al. (115) and Alam et al. (116)].
However, the activation of cells under pathological conditions was revealed for contributing to the progression of numerous neurodegenerative diseases. The discharge of pro-inflammatory mediators in the brain stimulated through various stimulants, including Aβ, was exhibited to account for the inflammatory constituent of neuronal loss in AD (117–119). CA and its derivatives’ recognized anti-inflammatory result are of prospective benefit in combating AD through these agent candidates.
In addition, activation of Nrf2 has been explained for inhibiting inflammatory gene expression (120, 121) by signaling crosstalk linking the HO-1 (122). Kim et al. (123) have illustrated that Nrf2-induced HO-1 introduction of CAPE is correlated with its anti-inflammatory and antioxidant mechanisms. Hence, there is a relationship between CA derivatives’ antioxidant and anti-inflammatory effect and their therapeutic promise to AD through their multifunctional results. A research (22) has confirmed that the enhanced NF-κB, p65, and 5-LOX correlated with the global cerebral ischemia-reperfusion neuronal injury and memory failure in rats is inverted through CA (10–50 mg/kg) treatment. However, CA (50 mg/kg) also improved neuronal loss and infarct volume 24 h after ischemia (124). The anti-inflammatory result of other CA esters in microglial cells was connected to the induction of HO-1 (105, 125).
Anticancer Effect of Caffeic Acid
CA has potential anticancer roles in several human cancers (126–128). Literature analysis led to attractive findings on CA and its therapeutic potentials. Several studies explain an inhibitory effect of CA on cell migration and invasion, with potential activity in decreasing metastases in tumor cells (129). Below are the effects of CA on numerous cancers.
Breast Cancer
One of the deadliest malignancies among women is breast cancer. The migration rate inhibition of breast tumor is treated via CA (130). CA decreased MDA-MB-231 and MCF-7 cell growth, repressing breast tumors’ proliferation (131). The experimental and clinical findings reveal a variety of antitumor functions of CA against ER+ and ER¯ breast tumors, which can sensitize cancer cells to tamoxifen and decrease breast tumor growth (131, 132). This study illustrates that CA mimics the activities of antiestrogens and modifies main growth regulatory signaling ER/cyclin D1 and IGF-IR/pAkt, resulting in damaged cell-cycle development and decreased cellular proliferation (131). CA exhibits a potent antiradical and antioxidant activity in breast tumors, acting by diverse mechanisms. It is a potential and practical clinical move toward presenting less toxicity for healthy cells and more action toward their malignant counterparts (133). CA decreases IL-12-making and NF-κB activation. However, it hinders with the TLR4 pathway via disrupting the TLR4/MD2 complex. Decreased regulation of TRIF, TLR4, and IRAK4 expression provokes apoptosis in breast cancers (10).
Prostate Cancer
Prostate cancer (PCa) is the most frequent cancer in developed countries (116, 134), resistant to cell death. Due to this resistance, it is necessary to develop a novel therapeutic approach; phenolic acids have revealed a relationship with a reduced risk of PCa (135, 136). CA has been linked with a lesser risk of advanced PCa. However, more intake of CA can be connected with decreased risk of PCa (137). CA treatment reduced Skp2 and Akt1 expression in LNCaP cancers as compared with control (138). NF-κB is constitutively stimulated in PCa cells and inhibition of NF-κB action that links with the repression of invasion, angiogenesis, and metastasis. IκBα inactivates the NF-κB transcription via fronting the nuclear signals of NF-κB proteins and keeps them inactive in the cytoplasm (137). CAPE is an NF-κB inhibitor as well as a 5α reductase inhibitor. It is potent for the treatment of PCa. CAPE may block NF-κB activation in PC-3, and it inhibits NF-κB activation (139).
Lung Cancer
Lung cancer is the leading cause of malignancy-related death worldwide (140). NSCLC is reported to comprise more than 85% of all lung cancers, and approximately 60% of NSCLC presents with advanced stage at the time of diagnosis (140, 141). However, chemotherapy to NSCLC is inadequate because of adverse effects of drug resistance and it contributes small to survival (142). CA may prevent oxidative stress-induced exposure to UVB and DNA injury in lymphocytes (93). A study has illustrated the defensive effect of CA on PTX-mediated apoptosis in A549 cells by the NF-κB pathway (143). Another study has found that a dose of CA (600 μM) drastically stimulates apoptosis in H1299 and A549 cells and has a potential function in modulating and increasing PTX-mediated cell death of NSCLC in in vivo and in vitro MAPK signaling. However, the combined treatment of CA with PTX reduced the proliferation of H1299 cells except for not normal Beas-2b cells. CA blocked H1299 cell growth via stimulating apoptosis, and PTX and CA combined created a synergistic antitumor result in H1299 cells (144). The effects of chemopreventive agents on TGF-β-mediated invasive phenotype utilizing A549 cells as a model scheme have been examined using CAPE as a therapeutic drug. CAPE efficiently repressed TGF-β-increased cell motility and TGF-β-mediated Akt activation and a specific PI3K/Akt signaling inhibitor, LY294002 (145, 146).
Melanoma
Because of the strong resistance of melanoma to conventional chemotherapy, numerous studies focused on novel treatment approaches and coadjuvants were investigated. Studies representing an inhibitory effect of CA on cell migration and invasion with potential activity in decreasing metastases in tumor cells are attracting more attention and are increasingly frequent in the scientific data (129). Additionally, research explains the potential of CA in decreasing proliferation, growth, and cell viability in numerous cancers, and a capability to induce via apoptosis (129, 147). Results illustrated a reduction in cell viability, inhibition of colony formation, cell cycle modulation, and modifications in the gene expression of caspases following CA treatment (148). However, these results recommend an anticancer effect of CA on SK-Mel-28 cells. CA might play a key function in inhibiting cancer progression in melanoma cells (148) and efficiently blocked melanin-making in B16 melanoma cells (149). However, CAPE is recommended for suppressing ROS-mediated DNA damage in A2058 cells compared to other potential defensive agents (150).
Oral Cancer
Oral cancer is the most general kind of head and neck cancer. Hence, more than 90% of oral cancers are OSCC (151–153). Pro- and anti-apoptotic Bcl-2 proteins are involved in oral cancer (154–156). The migration rate of OSCC may be drastically blocked via the biological action of CA (157). Treatment with CAPE prevents the expression and activity of COX-2 in OSCC (158). CAPE treatment can inhibit the activity and plenty of COX-2 and EGFR. Administration of CAPE may inhibit and hinder the progression of oral tumors (159). Hence, CAPE is sturdily efficient against oral cancer cells. It prevents the COX-2 and EGFR activities and proliferation, growth, survival, and metastasis, and it hampers with the PI3K-Akt pathway and Skp2 in oral tumor (159).
Liver Cancer
HCC is a leading form of liver cancer, distinguished by being a malignant main solid tumor that differs from hepatocytes (160, 161). CA performs in HCC via its strong antioxidant capability, which inhibits ROS-making, decreases oxidative stress, and is very general in liver cancer (162, 163). CA performs as a primary as well as secondary antioxidant. It may act in the angiogenesis of HCC cells by decreasing the phosphorylation of JNK-1 and reducing the activation of HIF-1α. Animal studies treated with CA and CAPE proved the endorsed repression of cancer growth in HepG2 and the reduction of cancer invasion on a metastatic position in the liver. However, CA and CAPE prevent and obstruct the MMP-9 action via blocking NF-κB function (11, 164). In vitro and in vivo investigations have revealed that CA exerted its antihepatocarcinoma result, dependent on a variety of mechanisms including cell death via induction of TRAIL signaling and the activation of caspase 9, liberation of cyt-c, and creation of the apoptosome (32). Hence, CA is an anticancer agent for inhibiting HCC (165). CA was established to be a stimulant of HO-1, GCLC, and GCLM expression by the Nrf2/ERK pathway, and it can be an efficient chemoprotective agent to defend liver injury against oxidative harm (166).
Cervical Cancer
CA has been known for its antioxidant function in normal cells and pro-oxidant function in tumor cells. Hence, this pro-oxidant-induced oxidative DNA break and its downstream pathway stimulate cancer cell death (167, 168). CA treatment has altered ROS and modified MMP in ME-180 and HeLa cells (167, 169). The enhanced apoptotic morphological alterations in CA-treated cells were examined in ME-180 and HeLa cells. CA possesses an antitumor effect via its pro-oxidant function. It considerably decreases the proliferation of HeLa cells in a concentration-dependent manner. The morphological proof of cell death such as nuclei fragmentation has been noticeably detected after exposure to CA by flow cytometry. CA reduces the levels of uncleaved caspase-3 and Bcl-2 and provokes cleaved caspase-3 and p53 (170).
CA stimulates apoptosis via blocking Bcl-2 action, leading to the liberation of cyt-c and following caspase-3 activation, representing the fact that CA induces cell death by the mitochondrial apoptotic pathway. CA has a potent anticancer effect and can be a promising chemotherapeutic agent (170). It interrelates synergistically with 5−FU, leading to a decline of apoptosis in HeLa cells with the lowest quantity of hemolytic activity (171). CDDP and CA have been selected, and their CxCa antitumor action has been calculated in combination. In CaSki and HeLa cells, a combination index <1 to CDDP and CFC showed the synergistic growth/survival inhibition that drastically enhanced caspase−3, −7, and −9 expression. CFC can be an aspirant antitumor agent and, when exploited in combination, might enhance the therapeutic efficiency of CDDP (172). Synergistic results of CA-cisplatin stimulate apoptosis in cervical cancer cells by the mitochondrial pathway (172).
Colorectal Cancer
Colorectal cancer (CRC) is the third leading cause of malignancy-related death in the United States. Huge common CRC cases may be attributed to environmental reasons, as they account for more than 70% of all occurrences (173). Some features of western dietary outlines can also persuade the risk of CRC. However, adiposity and high insulin levels in obese subjects are associated with an enhanced risk of CRC (174). CA has been revealed to have potential antitumor effects in cell cultures and animal models and might play a defensive role against CRC (175). CA prevents the growth of colon cancer and is a potent antitumor agent that enhances AMPK activation and induces apoptosis in CRC cells. However, the structure of CA may be utilized for the rational plan of novel inhibitors, which target human CRC (176). A meta-analysis documented a decreased occurrence of colon cancer in more versus no- or less-coffee-taking subjects in case-control investigations, but not in cohort studies to CRC (177). CA consumption decreases the risk of colon cancer. It sturdily repressed MEK1 and TOPK actions and bound to either MEK1 or TOPK. CA blocked ERKs phosphorylation, AP-1, and NF-κB and consequently prevented TPA-, EGF-, and H-Ras-mediated neoplastic transformation of JB6 P+ cells. CA targets MEK1 and TOPK for suppressing colon cancer metastasis as well as neoplastic cell transformation (15). It endorses apoptosis in HCT-116 and SW-480 in a dose-dependent way (176). Treatment with CA inhibited PDK1, Akt, and mTOR phosphorylation.
CA Prevents Photoaging and Photodamage of the Skin
CA-blocks UVB-irradiation mediated more expression of MMP-1 and -9 in human skin fibroblasts. CA-affected abdominal skin repressed UVA-irradiation-mediated ROS; CA was found in the skin after oral ingestion (178). CA pretreatment decreased the cytotoxicity of HaCaT after UVA irradiation and repressed UVA-irradiation-mediated MMP-1 action and mRNA oxidant creation. CA upregulated the GSH content and mRNA of γglutamate-cysteine ligase, and mRNA catalase and glutathione peroxidase expression in UVA-irradiated cells. CA generated defensive effects on UVA-irradiation-induced MMP-1 induction in HaCaT, probably as it restored the antioxidant protection system on cellular and molecular levels (178, 179). However, CA showed weak collagenolytic action; topically concerned CA defended the skin from UVB-irradiation-mediated erythema. In vitro and in vivo research revealed that CA can be effectively utilized as a topical defensive agent against UV-irradiation-mediated skin injury (178).
CA attenuates the local immune repression provoked via UVB irradiation and blocks the UVB-irradiation-mediated IL-10 promoter, IL-10 mRNA expression, and protein-making in mouse skin (178, 180). CA can present IL-10-making by interfering with prostaglandin E2 production connected to stimulating the UVB-irradiation-mediated immune repressive cytokine system. CA considerably prevented the UVB-irradiation-mediated activation of MAPK pathway AP-1 and NF-κB. It may have a therapeutic potential as a topical defensive agent against the adverse effects of UVB radiation (181, 182). CA blocked the TPA-dependent motivation of DNA synthesis and enhanced the number of cancers. CA efficiently suppressed UVB-irradiation-mediated COX-2 and prostaglandin E2 in JB6 cells and repressed UVB-irradiation-mediated skin carcinogenesis via preventing the Fyn kinase action (178, 183).
Caffeic Acid Combined With Chemotherapeutics or Nanoparticles
The combination therapy is the prevention and treatment strategy with two or more drugs with the target of accomplishing the comparable effectiveness levels with negligible toxicities at doses lesser than normal, which induces better synergistic/additive effects (184, 185). Hence, successful combinations of promising therapeutic drugs/nanoparticles with products can achieve the needed conclusion but with a slighter toxicity outline (186, 187). CA and cisplatin illustrated potent antitumor action in the tumor. Furthermore, cisplatin-sensitive cells, while exposed to combination therapy of CA and cisplatin, which elucidated speedily, improve in the activity of apoptotic cascade by increased caspase action in contrast to only administrating 5 μM cisplatin. However, combining 5:50 μM (cisplatin/CA) enhances the caspase activity via 4:3 folds through 60% cell viability in A2780cisR cells (188). However, cisplatin combined with CA improved its therapeutic consequence that led to the inhibition of cell survival of HeLa and CaSki cells, which could be revealed by the synergistic effect. Therefore, this combination was correlated with increasing caspase-3, -7, and -9 (172).
Metformin (Met) and CA were recognized to have synergistic results, while combined with anticancer therapies, chiefly to HTB-34 cells (126). CA combined with Met and cytotoxicity mechanism moved toward apoptosis without distressing healthy human fibroblasts. Studies continue to examine the mechanism of the anticancer role of the combination of Met and CA (189). The cytotoxicity of CA-coated iron oxide nanoparticles (CAMNPs) enhanced twofold in tumor cells. The proficient features of CAMNPs may be integrated into appliances, therefore enhancing the efficiency of clinical tumor treatment (190). Antitumor action of nanoparticles has been evaluated with DOX-resistant CT26 cells. Hence, DOX-loaded nanoparticles of ChitoCFA/CMD are a potent vehicle for antitumor drug targeting (191). The CA-SLN formulation might be potent as an alternative nanosize-based drug delivery system for tumor treatment (192).
Neuroprotective Effect of Caffeic Acid
CA generates antidepressive-like action in forced-swim mice (19, 193). It demonstrates anxiolytic-like results in rats running a maze, which defends against H2O2-mediated oxidative break of rat brain tissue (19, 194). CA attenuates the less regulation of the brain-originated neurotrophic factor that plays significant functions in depression pathophysiology and treatment (195, 196). It decreases pathological states after focal cerebral ischemia in rats, which attenuates neuronal injury, astrogliosis, and glial scar-making in cryoinjured mouse brains (19). CA decreases oxidative stress in the rat hippocampus following pilocarpine-mediated seizures (197) and defends mouse brains from aluminum-mediated injury (198). It decreases inflammatory damage in the striatum of MPTP-treated mice (199, 200). CA defends cerebellar granule neurons from cell death mediated via the neurotoxin MPP+ (201). It illustrates neuroprotective results against amyloid-β–mediated neurotoxicity via blocking calcium influx as well as tau phosphorylation (202). CA can contribute to inhibiting neurodegeneration and brain damage, and articulates anxiolytic-like functions. Systemic administration of LPS mediated profound immobility, enhanced the TNF-α and IL-6 levels, and changed the host antioxidant protection in animals (193). Several in vitro studies revealed that CA exhibits a broad-spectrum neuroprotective summary. CA is defensive against the H2O2-mediated oxidative stress that attenuated the H2O2-mediated cell damage in cultured cerebellar granule neurons (203). CA decreased the acrolein-mediated neurotoxicity via the Akt/GSK3 pathway stimulation in HT22 cells (73). In vitro studies demonstrate that CA defends neurons from a broad range of apoptosis-mediating agents. A study (204) proved that repetitive administration of CA defended the mouse brain from aluminum-mediated injury. CA can have particular selectivity related to the AChE from diverse brain regions (205). Hence, statistics from animal studies show that CA might inhibit neuronal injury/death caused via diverse stressors saying that CA is a potent neuroprotective agent for the treatment and prevention of neurodegenerative diseases (Table 1).
Neurodegenerative disorders include a heterogeneous cluster of diseases linked to progressive deterioration of the structure and performance of the peripheral or CNS. The categorization of neurodegenerative diseases depends on clinical symptoms, damaged brain areas, influenced cell types, changed proteins, and etiology. However, affected brain areas have symbols of atrophy and defective metabolic action (214). CA affects Parkinson’s (215, 216) and Alzheimer’s diseases and other neurodegenerative diseases (216, 217)
Alzheimer’s Disease
Alzheimer’s disease (AD) is a progressive, neurodegenerative disorder distinguished via deterioration of cognition and memory, progressively interfering with daily living actions attended through neuropsychiatric symptoms and behavioral disorders (121, 218). However, AD is the most general form of dementia (219). The disease is distinguished by general behavioral symptoms such as apraxia, agnosia, aphasia, erratic emotion, sleep disorders, and interpersonal or social worsening (220, 221). One of the well-identified pathological characteristics of AD is the deposition of amyloid-beta (Aβ), which contributes to neuronal cell death by several mechanisms, such as the disorder of cellular oxidant–antioxidant equilibrium. However, the agent can modify the Aβ creation that increases the antioxidant in neuronal cells, which increases cell viability and might offer therapeutic opportunities for AD (222). CA administration affected a considerable reduction in AChE action and nitrite production in rats with AD compared with the AD model (223). CA repressed inflammation, oxidative stress, NF−κB−p65 protein, and caspase−3 action and regulated the p53 protein expression and p-p38-MAPK expression in rats with AD. However, the useful effects of CA on learning deficits in the AD model were because of the repression of inflammation and oxidative stress by the p38-MAPK pathway (223). CA inhibited AlCl3-mediated dementia in rats (206). It blocked memory deficits mediated through the focal cerebral ischemia (224). The expression of p-tau protein reduced in the rat’s hippocampus administered with CA in contrast to the HF group (225). The antidementia action of CA against AlCl3-mediated dementia in rats (206). Hence, the conclusion of this study suggests a wider extent for screening CA against neurodegeneration-linked disorders.
The combined result of CA and ChA against AD has been performed to find its synergistic/additive result in the biological scheme. The approach targeting the inhibition of AChE and BChE has an excellent effect against AD (226). The combination therapy of ChA and CA demonstrated a synergistic action. However, the mechanism by which the combination therapy exerts its neuroprotective functions is to prevent AChE and BChE actions and inhibit oxidative stress-mediated neurodegeneration (227). The detected synergistic result of combination for these enzymes may improve efficiency and protect these ingredients against neurological states, including AD and PD (Figure 2). CA and CAF combination treatment on a specific ratio might be an enhanced option for further assessment against AD. However, the food ingredients are found in combination and hence promising for exerting a superior neuroprotective effect (229).
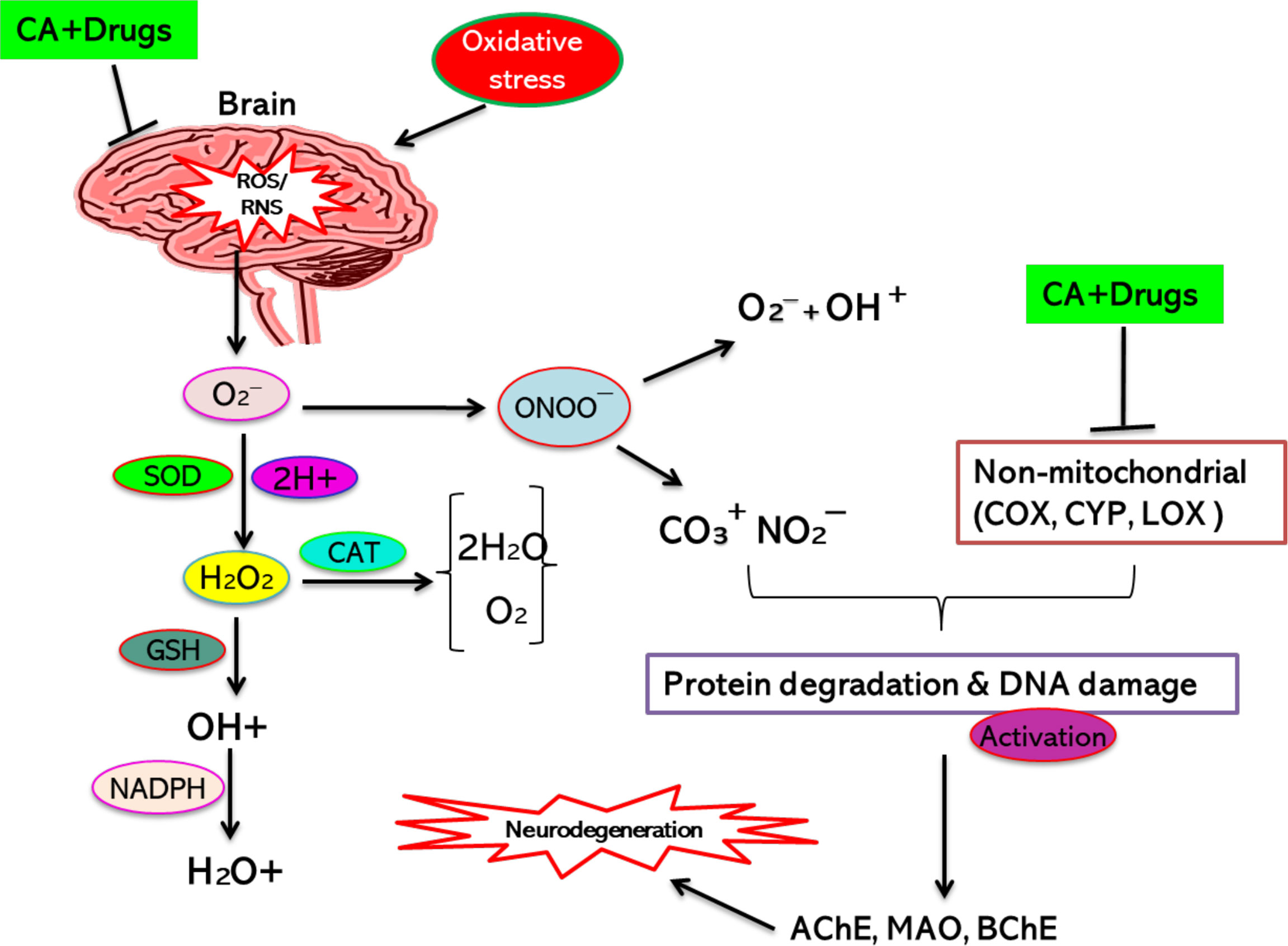
Figure 2 CA’s synergistic action with other agents/drugs (neuroprotective) for inhibiting neurodegeneration. [Adapted from Maity et al. (228)]. This figure was drown by ChemBioDraw.
Parkinson’s Disease
Parkinson’s disease (PD) is a progressive and degenerative disease that affects more than 6 million public (230). Motor, as well as nonmotor, symptoms manifest it. Hence, the motor symptoms include resting tremor, bradykinesia, rigidity, and postural instability. Spontaneous movement is drastically reduced, with the failure of facial expression, decreased blink rate, and damaged spontaneous swallowing, which affects sialorrhea. However, patients with PD can experience retropulsion and festination (231, 232). The nonmotor symptoms include anxiety, dysautonomia, cognitive decline, anosmia, depression, urinary complaints, disturbances, sleep, and orthostatic hypotension (233, 234). CA’s inhibitory action against α-synuclein fibrillation could lead us to plan novel therapeutic agents for PD (235). CA pre-intake has sustained TH action, protein-making, and dopamine synthesis. It is a promising neuroprotective drug for the development of PD (236). CA possesses neurotrophic results exerted by the modulation of Akt and ERK1/2 pathways, hence representing potential agents for finding neurotrophic compounds for the treatment of neurodegenerative disorders (237). However, the anti-inflammatory action of CA emphasized its neuroprotective action against rotenone-mediated neurodegeneration in mice (238).
Biomolecular Interactions
CA interacts with several protein molecules, genes, and chemicals. Around 9 enzymes are identified with similarity >0.85 that CA targets. Table 2 represents the list of target proteins generated from The Binding Database (http://www.bindingdb.org).
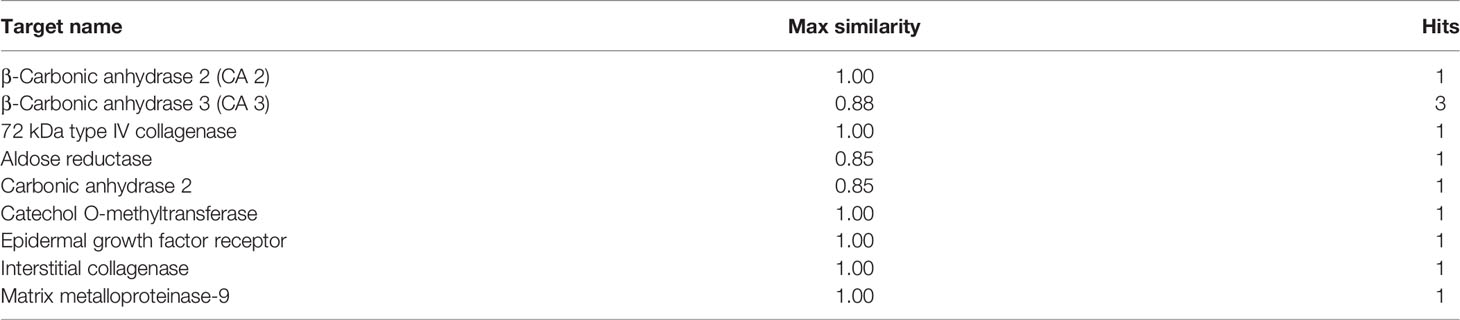
Table 2 List of enzyme inhibition constant data from Binding DB for compound caffeic acid similarity at least >0.85.
β-Carbonic anhydrase 3 (CA 3) from Mycobacterium tuberculosis is the best target enzyme with a similarity of 0.88 to the ligand. It has the highest negative ΔG° at -8.51 kcal/mol among all the targets. It is followed by β-carbonic anhydrase 2 (CA 2) from Mycobacterium tuberculosis with ΔG° at -8.49 kcal/mol and similarity of 1.00. The PDB includes 12 PDB complex structures with CA. Each is defined in Table 3 with inferences on inhibitory and activation capabilities of CA.
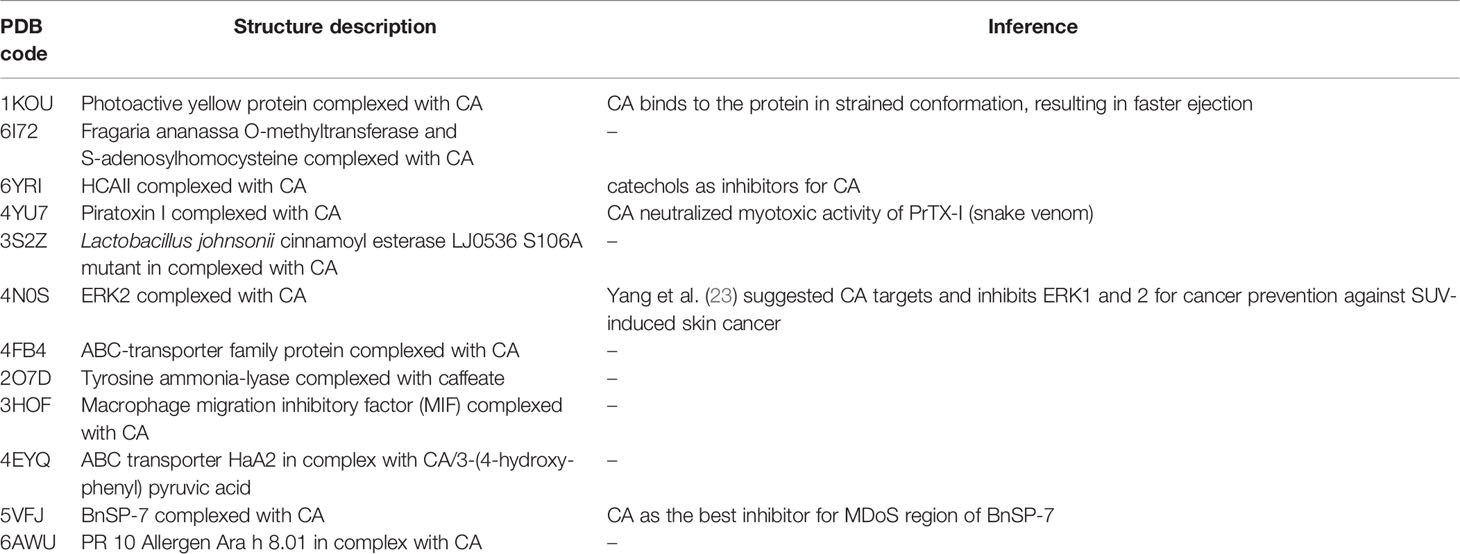
Table 3 List of protein-bound 3D structures complexed with caffeic acid and inferences on binding affinity.
The PDB ID 4N0S, ERK2 complexed with CA has potential medical significance with validation. In a study on ERKs, CA binds strongly with ATP-binding cleft through hydrogen bond formation to specific amino acids (Figure 3). The residues on the hinge loop, Q105, D106, and M108, bind to the 3’ hydroxyl and 4’ hydroxyl ends, respectively (23), thus proving the molecular level impact of CA on enzymes. The experiments were performed in vivo in mice. CA suppresses the SUV-induced ERK phosphorylation with further downstream signaling in HaCaT cells. CA reduces SUV-induced skin cancer before or after exposure to SUV (23, 239). Studies also revealed that the knockdown of ERK2 reduced CA sensitivity in skin cancer cells. Reduction in ERK2 levels decreases CA’s ability to prevent skin cancer. CA suppresses skin cancer when exposed to SUV in mice. It is an inhibitor of ERK1/2 and blocks the activities of downstream substrates such as Elk1, c-Myc, and RSK2. However, CA may be a good anti-skin cancer agent and effective against SUV-mediated skin tumors (23, 239, 240).
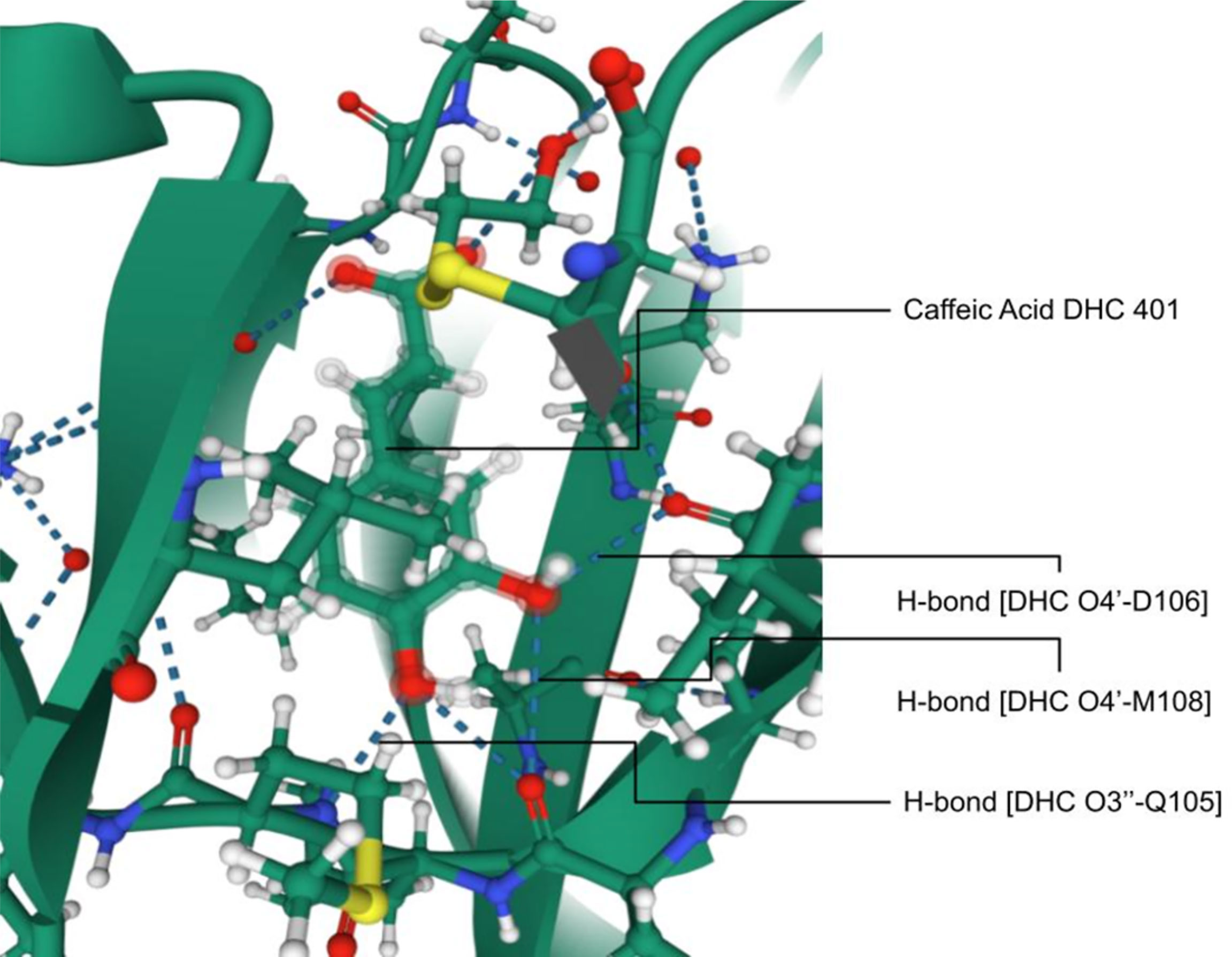
Figure 3 Interaction of ERK2 with caffeic acid; hydrogen bonding between DHC O4’-D106, M108, and CA O3’-Q105 imparting molecular level inhibition of the protein [Adapted from Yang et al. (23)].
Molecular Targets of Caffeic Acid in Therapeutics
CA attaches directly with Fyn noncompetitively by ATP and represses UVB-mediated COX-2 and PGE2-making via the inhibition of Fyn kinase action in vivo (241, 242). The inhibition affected the attenuation of UVB-mediated COX-2 promoter action and AP-1 and NF-κB in JB6 P+ cells. UVB-mediated phosphorylation of JNKs, p38, and ERKs is found in JB6 P+ cells (243, 244). CA is more efficient than chlorogenic acid at preventing Fyn kinase and in repressing the activation of MAPK pathways. However, B-Raf mutations happen commonly in colon polyps and are an early starting incident in melanoma and colon cancer (245). MEK1/2 has unique features among the components of MAPK pathways (246, 247).
Constitutive MEK1 activation affects transformation and cancer, whereas an inhibitor of MEKs represses transformation and cancer growth in mouse models and cell culture (248, 249). Lymphokine-activated killer TOPK is a kinase; an activator of ERKs and TOPK action emerges to be engaged in TOPK’s oncogenic role in tumors (245, 250). CA prevented CT-26 cell-mediated mouse lung metastasis in mice and neoplastic cell transformation through repressing ERKs phosphorylation (15, 245). Decaffeinated coffee or CA prevented CT-26 cell-mediated COX-2, MMP-2, and -9 action, and ERKs phosphorylation (251, 252). Computational modeling study results together with laboratory experiments exhibited that CA interacted particularly with mitogen-activated MEK1 and TOPK with ATP and repressed their respective kinase action (245). The inhibition of TOPK and MEK1 has been linked with TPA-mediated ERKs and p90 RSK2 and attenuation of AP-1 and NF-κB transactivation (245, 253, 254). CA/coffee consumption has been linked with reduced ERKs phosphorylation in colon cancer (242, 245, 255). However, the molecular targets and CA effects are summarized (Figure 4). CA was confirmed to be a stimulant of HO-1, GCLC, and GCLM by ERK/Nrf2 signaling, and it could be an efficient chemoprotective drug for defending liver injury against oxidative damage (166). CA exerts chemopreventive action against solar UV-mediated skin carcinogenesis via targeting ERK1/2 (23).
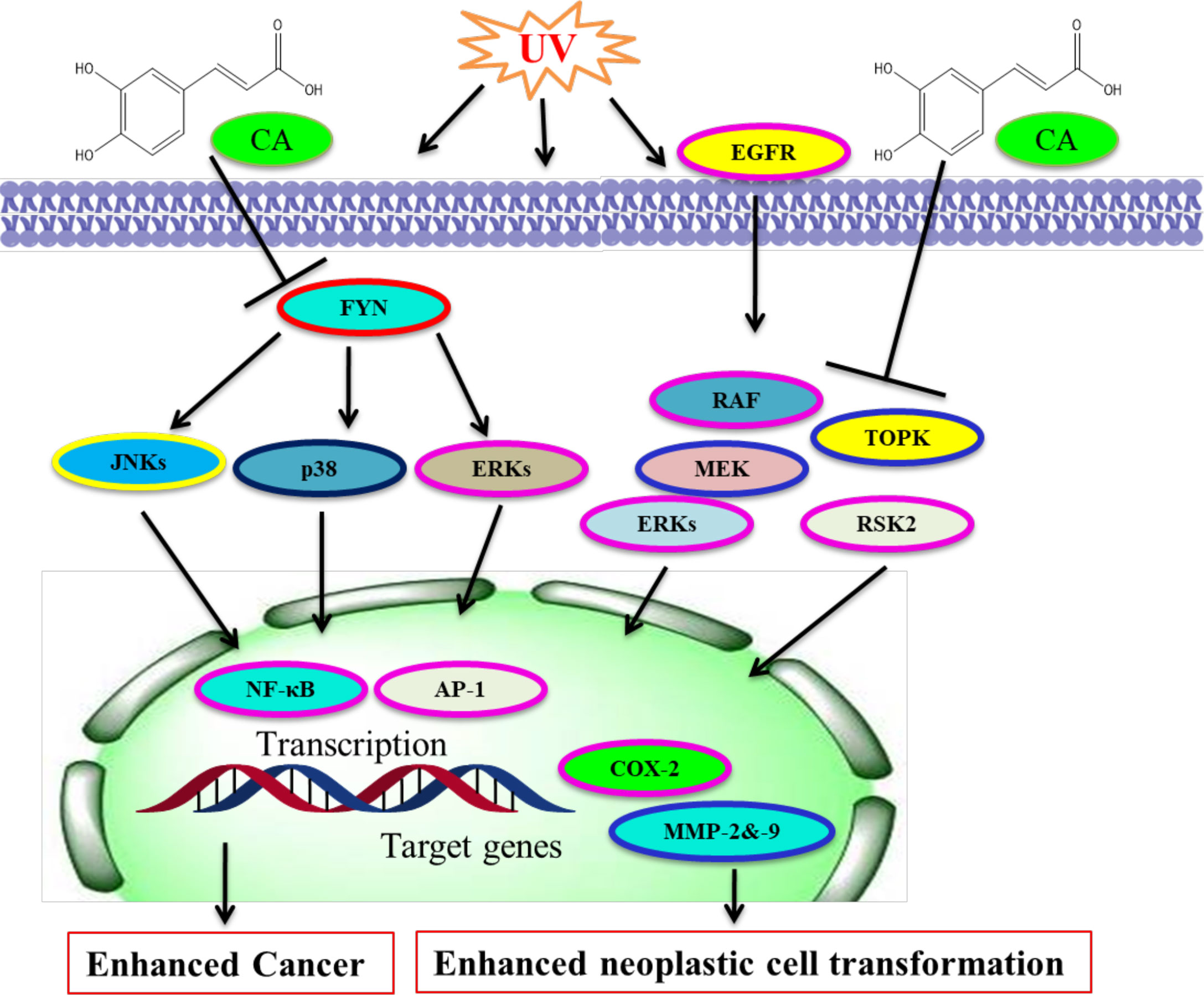
Figure 4 Molecular targets of caffeic acid. CA binds with Fyn for blocking its kinase activity and UV-mediated skin cancer. CA binds with MEK and TOPK to prevent their kinase actions and neoplastic cell transformation. [Adapted from Bode et al. (245)]. This figure was drown by ChemBioDraw.
Some pharmacological effects of CA on CNS, with/without the initiation of neurotoxic results, have been elicited by a molecular target. The treatment with CA has detected a defensive effect against H2O2-mediated oxidative injury on brain tissue. Hence, CA stimulated DNA breaks in brain tissue (256). CA’s neuroprotective results against Aβ-mediated neurotoxicity in vitro prevent peroxynitrite-mediated neuronal injury (257, 258). CA performs as a selective 5-LOX inhibitor and defended mice from aluminum-mediated neuronal injury by the downregulation of APP and Aβ protein (204). The antioxidant effect of CA has been explained to be neuron-defensive in vivo and ameliorates brain damages after focal cerebral ischemia in pathological states (124). CA and its interface with intracellular molecules tell that CGA exerts its neuroprotection through its caffeoyl acid set, potentially being a therapeutic drug in neurodegenerative diseases linked with glutamate excitotoxicity (259). A natural and potent CA might be considered promising therapeutic in diseases that link the cholinergic system (205).
Conclusion and Future Directions
CA, a phenolic compound, is plentiful in medicinal plants. It has numerous biological effects, including antioxidation, anti-inflammation, anticancer, and neuroprotective. CA is involved in several biochemical pathways and suitable targets are also identified. The biological activities of CA come from its functional groups. It is possible to enhance the anticancer capabilities of CA by altering these functional sites. The potential therapeutic effects of CA are mediated via repression of MMP-2 and -9 and inhibited NF-κB, AP-1, ERKs, STAT3, and VEGF. The activity of CA is displayed in several molecular mechanisms that regulate cell survival and stimulate apoptosis.
Broad in vitro and in vivo examinations have revealed that CA exerts anticancer and neuroprotective effects indicating its preventive and therapeutic potential for diverse cancers and neurodegenerative disorders. Hence, the multifunctional properties of CA have been illustrated for displaying the anti-AD result in vitro and in vivo. The diversity of biochemical mechanisms of CA was exhibited as a promising therapeutic potential for neurological diseases. The effects of CA in cancer and neurodegenerative disorders could be affected by the synergistic activity of various active agents. The therapeutic effects of CA may be synergistically increased by other drugs/agents. However, the clinical study must be persisted to entirely evaluate the relationship between CA and other agents/drugs and the risk of cancer and neurodegenerative diseases.
Further studies, the basic research, clinical study, and epidemiological level, carried out with a standardized strategy are required for better understanding the assessment of a broad range of CA in the clinical management and treatment of cancer and neurological diseases. Hence, the inclusive study of CA based on clinical trials could be extremely beneficial in the approach and plan of novel therapies for cancer and neurological diseases.
Author Contributions
MaA: Conceptualization, writing—original draft preparation, data curation, investigation, and methodology. SaA: Data analysis, validation, and visualization. AME: Formal analysis, supervision, investigation, and validation. MoA: Data curation, validation, and writing—review and editing. ShA: Methodology and writing—review and editing. MIH: Conceptualization, writing—original draft preparation, and investigation. ViP: Conceptualization, data analysis, validation, project administration, writing—review, and editing. All authors contributed to the article and approved the submitted version.
Funding
This work is supported and funded through the Indian Council of Medical Research (Grant No. 45/6/2020-DDI/BMS).
Conflict of Interest
The authors declare that the research was conducted in the absence of any commercial or financial relationships that could be construed as a potential conflict of interest.
Publisher’s Note
All claims expressed in this article are solely those of the authors and do not necessarily represent those of their affiliated organizations, or those of the publisher, the editors and the reviewers. Any product that may be evaluated in this article, or claim that may be made by its manufacturer, is not guaranteed or endorsed by the publisher.
Acknowledgments
AME extends his appreciation to the Deanship of Scientific Research at Jouf University for funding his work through Research Grant Number (DSR-2021-01-0368). MA expresses thanks to the Indian Council of Medical Research for financial support (Grant No. 45/6/2020-DDI/BMS). MH expresses gratitude to the Department of Science and Technology, Government of India, for the FIST support (FIST program No. SR/FST/LSII/2020/782).
Abbreviations
CA, Caffeic acid; CAPE, Caffeic acid phenethyl ester; PhA, Phenolic acids; MA, Monocarboxylic acid; OSCC, Oral squamous cell carcinoma; HCC, Hepatocellular carcinoma; COX-2, Cyclooxygenase 2; HCs, Hydroxycinnamic acids; CGA, Chlorogenic acid; AAA, Aromatic amino acids; PLP, Pyridoxal 5-phosphate; NAD, Nicotinamide adenine dinucleotide; TAL, Tyrosine ammonium lyase; NF-κB, Nuclear factor kappa B; VEGF, Vascular endothelial growth factor; PKC, Kinase C protein; CGA, Chlorogenic acid; JNK, C-Jun N-terminal kinase; MCT, Monocarboxylic acid transporters; CLL, Chronic lymphocytic leukemia; ROS, Reactive oxygen species; H2O2, Hydrogen peroxide; TNF, Tumor necrosis factor; AChE, Acetylcholinesterase; BChE, Butyrylcholinesterase; STAT3, Transcription factor and signal translation 3; EGFR, Epidermal growth factor receptor; Nrf2, Nuclear factor-E2 p45-related factor; TOPK, T-cell-originated protein kinase; MMP, Matrix metalloproteinases; VEGF, Vascular endothelial growth factor; ECM, Extracellular matrix; ERK, Extracellular signal-regulated kinase
References
1. Jiang R-W, Lau K-M, Hon P-M, Mak TC, Woo K-S, Fung K-P. Chemistry and Biological Activities of Caffeic Acid Derivatives From Salvia Miltiorrhiza. Curr Med Chem (2005) 12(2):237–46. doi: 10.2174/0929867053363397
2. Bryngelsson S, Dimberg LH, Kamal-Eldin A. Effects of Commercial Processing on Levels of Antioxidants in Oats (Avena Sativa L.). J Agric Food Chem (2002) 50(7):1890–6. doi: 10.1021/jf011222z
3. Castellari M, Sartini E, Fabiani A, Arfelli G, Amati A. Analysis of Wine Phenolics by High-Performance Liquid Chromatography Using a Monolithic Type Column. J Chromatogr A (2002) 973(1-2):221–7. doi: 10.1016/S0021-9673(02)01195-0
4. Rao BN. Bioactive Phytochemicals in Indian Foods and Their Potential in Health Promotion and Disease Prevention. Asia Pac J Clin Nutr (2003) 12(1):9–22.
5. Liu RH. Health-Promoting Components of Fruits and Vegetables in the Diet. Adv Nutr (2013) 4(3):384S–92S. doi: 10.3945/an.112.003517
7. Bel-Rhlid R, Thapa D, Kraehenbuehl K, Hansen CE, Fischer L. Biotransformation of Caffeoyl Quinic Acids From Green Coffee Extracts by Lactobacillus Johnsonii NCC 533. AMB Express (2013) 3(1):1–7. doi: 10.1186/2191-0855-3-28
8. Rahmani AH, Aly SM, Ali H, Babiker AY, Srikar S. Therapeutic Effects of Date Fruits (Phoenix Dactylifera) in the Prevention of Diseases via Modulation of Anti-Inflammatory, Antioxidant and Anti-Tumour Activity. Int J Clin Exp Med (2014) 7(3):483.
9. Mine Y. Egg Proteins and Peptides in Human Health-Chemistry, Bioactivity and Production. Curr Pharm Des (2007) 13(9):875–84. doi: 10.2174/138161207780414278
10. Chen C-Y, Kao C-L, Liu C-M. The Cancer Prevention, Anti-Inflammatory and Anti-Oxidation of Bioactive Phytochemicals Targeting the TLR4 Signaling Pathway. Int J Mol Sci (2018) 19(9):2729. doi: 10.3390/ijms19092729
11. Chung TW, Moon SK, Chang YC, Ko JH, Lee YC, Cho G, et al. Novel and Therapeutic Effect of Caffeic Acid and Caffeic Acid Phenyl Ester on Hepatocarcinoma Cells: Complete Regression of Hepatoma Growth and Metastasis by Dual Mechanism. FASEB J (2004) 18(14):1670–81. doi: 10.1096/fj.04-2126com
12. Tanaka T, Kojima T, Kawamori T, Wang A, Suzui M, Okamoto K, et al. Inhibition of 4-Nitroquinoline-1-Oxide-Induced Rat Tongue Carcinogenesis by the Naturally Occurring Plant Phenolics Caffeic, Ellagic, Chlorogenic and Ferulic Acids. Carcinogenesis (1993) 14(7):1321–5. doi: 10.1093/carcin/14.7.1321
13. Sul D, Kim H-S, Lee D, Joo SS, Hwang KW, Park S-Y. Protective Effect of Caffeic Acid Against Beta-Amyloid-Induced Neurotoxicity by the Inhibition of Calcium Influx and Tau Phosphorylation. Life Sci (2009) 84(9-10):257–62. doi: 10.1016/j.lfs.2008.12.001
14. Jung JE, Kim HS, Lee CS, Park D-H, Kim Y-N, Lee M-J, et al. Caffeic Acid and its Synthetic Derivative CADPE Suppress Tumor Angiogenesis by Blocking STAT3-Mediated VEGF Expression in Human Renal Carcinoma Cells. Carcinogenesis (2007) 28(8):1780–7. doi: 10.1093/carcin/bgm130
15. Kang NJ, Lee KW, Kim BH, Bode AM, Lee H-J, Heo Y-S, et al. Coffee Phenolic Phytochemicals Suppress Colon Cancer Metastasis by Targeting MEK and TOPK. Carcinogenesis (2011) 32(6):921–8. doi: 10.1093/carcin/bgr022
16. Korkina L. Phenylpropanoids as Naturally Occurring Antioxidants: From Plant Defense to Human Health. Cell Mol Biol (2007) 53(1):15–25.
17. Zduńska K, Dana A, Kolodziejczak A, Rotsztejn H. Antioxidant Properties of Ferulic Acid and its Possible Application. Skin Pharmacol Physiol (2018) 31(6):332–6. doi: 10.1159/000491755
18. Gerhauser C. Cancer Chemoprevention and Nutri-Epigenetics: State of the Art and Future Challenges. In: Natural Products in Cancer Prevention and Therapy (2012). p. 73–132.
19. Kim J, Lee KW. Coffee and its Active Compounds Are Neuroprotective. In: Coffee in Health and Disease Prevention. Elsevier (2015). p. 423–7.
20. Angelopoulou E, Paudel YN, Julian T, Shaikh MF, Piperi C. Pivotal Role of Fyn Kinase in Parkinson’s Disease and Levodopa-Induced Dyskinesia: A Novel Therapeutic Target? Mol Neurobiol (2021) 58(4):1372–91. doi: 10.1007/s12035-020-02201-z
21. Kinra M, Arora D, Mudgal J, Pai K, Rao CM, Nampoothiri M. Effect of Caffeic Acid on Ischemia-Reperfusion-Induced Acute Renal Failure in Rats. Pharmacology (2019) 103(5-6):315–9. doi: 10.1159/000497474
22. Liang G, Shi B, Luo W, Yang J. The Protective Effect of Caffeic Acid on Global Cerebral Ischemia-Reperfusion Injury in Rats. Behav Brain Funct (2015) 11(1):18. doi: 10.1186/s12993-015-0064-x
23. Yang G, Fu Y, Malakhova M, Kurinov I, Zhu F, Yao K, et al. Caffeic Acid Directly Targets ERK1/2 to Attenuate Solar UV-Induced Skin Carcinogenesis. Cancer Prev Res (2014) 7(10):1056–66. doi: 10.1158/1940-6207.CAPR-14-0141
25. Kasprzak-Drozd K, Oniszczuk T, Stasiak M, Oniszczuk A. Beneficial Effects of Phenolic Compounds on Gut Microbiota and Metabolic Syndrome. Int J Mol Sci (2021) 22(7):3715. doi: 10.3390/ijms22073715
26. Anantharaju PG, Gowda PC, Vimalambike MG, Madhunapantula SV. An Overview on the Role of Dietary Phenolics for the Treatment of Cancers. Nutr J (2016) 15(1):99. doi: 10.1186/s12937-016-0217-2
27. Bonta RK. Dietary Phenolic Acids and Flavonoids as Potential Anti-Cancer Agents: Current State of the Art and Future Perspectives. Anticancer Agents Med Chem (2020) 20(1):29–48. doi: 10.2174/1871520619666191019112712
28. Srinivasulu C, Ramgopal M, Ramanjaneyulu G, Anuradha CM, Suresh Kumar C. Syringic Acid (SA) A Review of Its Occurrence, Biosynthesis, Pharmacological and Industrial Importance. BioMed Pharmacother (2018) 108:547–57. doi: 10.1016/j.biopha.2018.09.069
29. Rahman MJ, Costa de Camargo A, Shahidi F. Phenolic Profiles and Antioxidant Activity of Defatted Camelina and Sophia Seeds. Food Chem (2018) 240:917–25. doi: 10.1016/j.foodchem.2017.07.098
30. Koyuncu I. Evaluation of Anticancer, Antioxidant Activity and Phenolic Compounds of Artemisia Absinthium L. Extract. Cell Mol Biol (Noisy-le-grand) (2018) 64(3):25–34. doi: 10.14715/cmb/2018.64.3.5
31. Asif M. Phytochemical Study of Polyphenols in Perilla Frutescens as an Antioxidant. Avicenna J Phytomed (2012) 2(4):169.
32. Espíndola KMM, Ferreira RG, Narvaez LEM, Silva Rosario ACR, da Silva AHM, Silva AGB, et al. Chemical and Pharmacological Aspects of Caffeic Acid and its Activity in Hepatocarcinoma. Front Oncol (2019) 9:541. doi: 10.3389/fonc.2019.00541
33. Abdelwahab TS, Abdelhamed RE, Ali EN, Mansour NA, Abdalla MS. Evaluation of Silver Nanoparticles Caffeic Acid Complex Compound as New Potential Therapeutic Agent Against Cancer Incidence in Mice. Asian Pac J Cancer Prev (2021) 22(10):3189–201. doi: 10.31557/APJCP.2021.22.10.3189
34. Yokoyama T, Kosaka Y, Mizuguchi M. Inhibitory Activities of Propolis and Its Promising Component, Caffeic Acid Phenethyl Ester, Against Amyloidogenesis of Human Transthyretin. J Med Chem (2014) 57(21):8928–35. doi: 10.1021/jm500997m
35. Zhang P, Tang Y, Li N-G, Zhu Y, Duan J-A. Bioactivity and Chemical Synthesis of Caffeic Acid Phenethyl Ester and Its Derivatives. Molecules (2014) 19(10):16458–76. doi: 10.3390/molecules191016458
36. Yang D, Huang Z, Jin W, Xia P, Jia Q, Yang Z, et al. DNA Methylation: A New Regulator of Phenolic Acids Biosynthesis in Salvia Miltiorrhiza. Ind Crops Prod (2018) 124:402–11. doi: 10.1016/j.indcrop.2018.07.046
37. Genaro-Mattos TC, Maurício ÂQ, Rettori D, Alonso A, Hermes-Lima M. Antioxidant Activity of Caffeic Acid Against Iron-Induced Free Radical Generation—A Chemical Approach. PloS One (2015) 10(6):e0129963. doi: 10.1371/journal.pone.0129963
38. Kim J, Soh SY, Bae H, Nam S-Y. Antioxidant and Phenolic Contents in Potatoes (Solanum Tuberosum L.) and Micropropagated Potatoes. Appl Biol Chem (2019) 62(1):1–9. doi: 10.1186/s13765-019-0422-8
39. Scarano A, Gerardi C, D’Amico L, Accogli R, Santino A. Phytochemical Analysis and Antioxidant Properties in Colored Tiggiano Carrots. Agriculture (2018) 8(7):102. doi: 10.3390/agriculture8070102
40. Celińska-Janowicz K, Zaręba I, Lazarek U, Teul J, Tomczyk M, Pałka J, et al. Constituents of Propolis: Chrysin, Caffeic Acid, P-Coumaric Acid, and Ferulic Acid Induce PRODH/POX-Dependent Apoptosis in Human Tongue Squamous Cell Carcinoma Cell (CAL-27). Front Pharmacol (2018) 9:336. doi: 10.3389/fphar.2018.00336
42. Karim N, Rubinsin N, Burukan M, Kamarudin S. Sustainable Route of Synthesis Platinum Nanoparticles Using Orange Peel Extract. Int J Green Energy (2019) 16(15):1518–26. doi: 10.1080/15435075.2019.1671422
43. Konishi Y, Kobayashi S. Transepithelial Transport of Chlorogenic Acid, Caffeic Acid, and Their Colonic Metabolites in Intestinal Caco-2 Cell Monolayers. J Agric Food Chem (2004) 52(9):2518–26. doi: 10.1021/jf035407c
44. Konishi Y, Hitomi Y, Yoshioka E. Intestinal Absorption of P-Coumaric and Gallic Acids in Rats After Oral Administration. J Agric Food Chem (2004) 52(9):2527–32. doi: 10.1021/jf035366k
45. Konishi Y, Kobayashi S, Shimizu M. Transepithelial Transport of P-Coumaric Acid and Gallic Acid in Caco-2 Cell Monolayers. Biosci Biotechnol Biochem (2003) 67(11):2317–24. doi: 10.1271/bbb.67.2317
46. Shen H, Tong X, Yang J, Yu L, Zhou H, Wang Y, et al. Biotransformation of Natural Hydroxycinnamic Acids by Gut Microbiota From Normal and Cerebral Ischemia-Reperfusion Injured Rats: A Comparative Study. Food Funct (2020) 11(6):5389–95. doi: 10.1039/D0FO00775G
47. Ito H, Gonthier M-P, Manach C, Morand C, Mennen L, Rémésy C, et al. Polyphenol Levels in Human Urine After Intake of Six Different Polyphenol-Rich Beverages. Br J Nutr (2005) 94(4):500–9. doi: 10.1079/BJN20051522
48. Lafay S, Morand C, Manach C, Besson C, Scalbert A. Absorption and Metabolism of Caffeic Acid and Chlorogenic Acid in the Small Intestine of Rats. Br J Nutr (2006) 96(1):39–46. doi: 10.1079/BJN20061714
49. Manach C, Scalbert A, Morand C, Rémésy C, Jiménez L. Polyphenols: Food Sources and Bioavailability. Am J Clin Nutr (2004) 79(5):727–47. doi: 10.1093/ajcn/79.5.727
50. Olthof MR, Hollman PC, Katan MB. Chlorogenic Acid and Caffeic Acid are Absorbed in Humans. J Nutr (2001) 131(1):66–71. doi: 10.1093/jn/131.1.66
51. Oliveira D, Bastos DHM. Biodisponibilidade De Ácidos Fenólicos. Quím Nova (2011) 34:1051–6. doi: 10.1590/S0100-40422011000600023
52. Lee Y, Howard L, Villalon B. Flavonoids and Antioxidant Activity of Fresh Pepper (Capsicum Annuum) Cultivars. J Food Sci (1995) 60(3):473–6. doi: 10.1111/j.1365-2621.1995.tb09806.x
53. Scalbert A, Williamson G. Chocolate: Modern Science Investigates an Ancient Medicine. J Med Food (2000) 3(2):121–5. doi: 10.1089/109662000416311
54. Kolodziejczyk-Czepas J, Szejk M, Pawlak A, Zbikowska H. Właściwości Przeciwutleniające Kwasu Kawowego I Jego Pochodnych. Żywn Nauka Technol Jakość (2015) 22(3).
55. Silva T, Oliveira C, Borges F. Caffeic Acid Derivatives, Analogs and Applications: A Patent Review (2009-2013). Expert Opin Ther Pat (2014) 24(11):1257–70. doi: 10.1517/13543776.2014.959492
56. Moridani MY, Scobie H, O'Brien PJ. Metabolism of Caffeic Acid by Isolated Rat Hepatocytes and Subcellular Fractions. Toxicol Lett (2002) 133(2-3):141–51. doi: 10.1016/S0378-4274(02)00105-4
58. Anjaly K, Tiku AB. Radio-Modulatory Potential of Caffeic Acid Phenethyl Ester: A Therapeutic Perspective. Anticancer Agents Med Chem (Formerly Curr Med Chem-Anti-Cancer Agents) (2018) 18(4):468–75. doi: 10.2174/1871520617666171113143945
59. Salim EI, Abd El-Magid AD, Farara KM, Maria DS. Antitumoral and Antioxidant Potential of Egyptian Propolis Against the PC3 Prostate Cancer Cell Line. Asian Pac J Cancer Prev (2015) 16(17):7641–51. doi: 10.7314/APJCP.2015.16.17.7641
60. Bak SP, Alonso A, Turk MJ, Berwin B. Murine Ovarian Cancer Vascular Leukocytes Require Arginase-1 Activity for T Cell Suppression. Mol Immunol (2008) 46(2):258–68. doi: 10.1016/j.molimm.2008.08.266
61. Corthay A, Skovseth DK, Lundin KU, Røsjø E, Omholt H, Hofgaard PO, et al. Primary Antitumor Immune Response Mediated by CD4+ T Cells. Immunity (2005) 22(3):371–83. doi: 10.1016/j.immuni.2005.02.003
62. Oršolić N, Knežević AH, Šver L, Terzić S, Bašić I. Immunomodulatory and Antimetastatic Action of Propolis and Related Polyphenolic Compounds. J Ethnopharmacol (2004) 94(2-3):307–15. doi: 10.1016/j.jep.2004.06.006
63. Wang Y, Kaur G, Kumar M, Kushwah AS, Kabra A, Kainth R. Caffeic Acid Prevents Vascular Oxidative Stress and Atherosclerosis Against Atherosclerogenic Diet in Rats. Evid Based Complement Alternat Med (2022) 2022. doi: 10.1155/2022/8913926
64. Oršolić N, Kunštić M, Kukolj M, Gračan R, Nemrava J. Oxidative Stress, Polarization of Macrophages and Tumour Angiogenesis: Efficacy of Caffeic Acid. Chem Biol Interact (2016) 256:111–24. doi: 10.1016/j.cbi.2016.06.027
65. Adem Ş, Eyupoglu V, Sarfraz I, Rasul A, Zahoor AF, Ali M, et al. Caffeic Acid Derivatives (CAFDs) as Inhibitors of SARS-CoV-2: CAFDs-Based Functional Foods as a Potential Alternative Approach to Combat COVID-19. Phytomedicine (2021) 85:153310. doi: 10.1016/j.phymed.2020.153310
66. Ali S, Alam M, Khatoon F, Fatima U, Elasbali AM, Adnan M, et al. Natural Products can be Used in Therapeutic Management of COVID-19: Probable Mechanistic Insights. Biomed Pharmacother (2022) 147:112658. doi: 10.1016/j.biopha.2022.112658
67. Butterfield DA, Drake J, Pocernich C, Castegna A. Evidence of Oxidative Damage in Alzheimer's Disease Brain: Central Role for Amyloid Beta-Peptide. Trends Mol Med (2001) 7(12):548–54. doi: 10.1016/S1471-4914(01)02173-6
68. Pereira C, Agostinho P, Moreira PI, Cardoso SM, Oliveira CR. Alzheimer's Disease-Associated Neurotoxic Mechanisms and Neuroprotective Strategies. Curr Drug Targets CNS Neurol Disord (2005) 4(4):383–403. doi: 10.2174/1568007054546117
69. Asha Devi S. Aging Brain: Prevention of Oxidative Stress by Vitamin E and Exercise. ScientificWorldJournal (2009) 9:366–72. doi: 10.1100/tsw.2009.46
70. Murakami K, Murata N, Noda Y, Tahara S, Kaneko T, Kinoshita N, et al. SOD1 (Copper/Zinc Superoxide Dismutase) Deficiency Drives Amyloid Beta Protein Oligomerization and Memory Loss in Mouse Model of Alzheimer Disease. J Biol Chem (2011) 286(52):44557–68. doi: 10.1074/jbc.M111.279208
71. Liu H, Wang H, Shenvi S, Hagen TM, Liu RM. Glutathione Metabolism During Aging and in Alzheimer Disease. Ann N Y Acad Sci (2004) 1019:346–9. doi: 10.1196/annals.1297.059
72. Moreira PI, Carvalho C, Zhu X, Smith MA, Perry G. Mitochondrial Dysfunction Is a Trigger of Alzheimer's Disease Pathophysiology. Biochim Biophys Acta (2010) 1802(1):2–10. doi: 10.1016/j.bbadis.2009.10.006
73. Huang Y, Jin M, Pi R, Zhang J, Chen M, Ouyang Y, et al. Protective Effects of Caffeic Acid and Caffeic Acid Phenethyl Ester Against Acrolein-Induced Neurotoxicity in HT22 Mouse Hippocampal Cells. Neurosci Lett (2013) 535:146–51. doi: 10.1016/j.neulet.2012.12.051
74. Deshmukh R, Kaundal M, Bansal V. Samardeep, Caffeic Acid Attenuates Oxidative Stress, Learning and Memory Deficit in Intra-Cerebroventricular Streptozotocin Induced Experimental Dementia in Rats. BioMed Pharmacother (2016) 81:56–62. doi: 10.1016/j.biopha.2016.03.017
75. Soares DG, Andreazza AC, Salvador M. Avaliação De Compostos Com Atividade Antioxidante Em Células Da Levedura Saccharomyces Cerevisiae. Rev Bras Ciênc Farm (2005) 41:95–100. doi: 10.1590/S1516-93322005000100011
76. Magnani C, Isaac VLB, Correa MA, Salgado HRN. Caffeic Acid: A Review of its Potential Use in Medications and Cosmetics. Anal Methods (2014) 6(10):3203–10. doi: 10.1039/C3AY41807C
77. Laranjinhas J, Vieira O, Almeida L, Modeira V. Inhibition of Metmyoglobin/H2O2-Dependent Low Density Lipoprotein Lipid Peroxidation by Naturally Occurring Phenolic Acids. Biochem Pharmacol (1996) 51(4):395–402. doi: 10.1016/0006-2952(95)02171-X
78. Lopes R, Costa M, Ferreira M, Gameiro P, Fernandes S, Catarino C, et al. Caffeic Acid Phenolipids in the Protection of Cell Membranes From Oxidative Injuries. Interaction With the Membrane Phospholipid Bilayer. Biochim Biophys Acta (BBA)-Biomembr (2021) 1863(12):183727. doi: 10.1016/j.bbamem.2021.183727
79. Birková A, Hubková B, Bolerázska B, Mareková M, Čižmárová B. Caffeic Acid: A Brief Overview of its Presence, Metabolism, and Bioactivity. Bioact Compd Health Dis (2020) 3(4):74–81. doi: 10.31989/bchd.v3i4.692
80. Khan F, Bamunuarachchi NI, Tabassum N, Kim Y-M. Caffeic Acid and Its Derivatives: Antimicrobial Drugs Toward Microbial Pathogens. J Agric Food Chem (2021) 69(10):2979–3004. doi: 10.1021/acs.jafc.0c07579
81. Bjørklund G, Chirumbolo S. Role of Oxidative Stress and Antioxidants in Daily Nutrition and Human Health. Nutrition (2017) 33:311–21. doi: 10.1016/j.nut.2016.07.018
82. Ahmed AA, Fedail JS, Musa HH, Kamboh AA, Sifaldin AZ, Musa TH. Gum Arabic Extracts Protect Against Hepatic Oxidative Stress in Alloxan Induced Diabetes in Rats. Pathophysiology (2015) 22(4):189–94. doi: 10.1016/j.pathophys.2015.08.002
83. Meyer AS, Donovan JL, Pearson DA, Waterhouse AL, Frankel EN. Fruit Hydroxycinnamic Acids Inhibit Human Low-Density Lipoprotein Oxidation In Vitro. J Agric Food Chem (1998) 46(5):1783–7. doi: 10.1021/jf9708960
84. Fukumoto L, Mazza G. Assessing Antioxidant and Prooxidant Activities of Phenolic Compounds. J Agric Food Chem (2000) 48(8):3597–604. doi: 10.1021/jf000220w
85. Sun-Waterhouse D, Thakorlal J, Zhou J. Effects of Added Phenolics on the Storage Stability of Avocado and Coconut Oils. Int J Food Sci Technol (2011) 46(8):1575–85. doi: 10.1111/j.1365-2621.2011.02655.x
86. Damasceno SS, Santos NA, Santos IM, Souza AL, Souza AG, Queiroz N. Caffeic and Ferulic Acids: An Investigation of the Effect of Antioxidants on the Stability of Soybean Biodiesel During Storage. Fuel (2013) 107:641–6. doi: 10.1016/j.fuel.2012.11.045
87. Kowalski R. Changes of Linoleic Acid Concentration During Heating of Some Plant-Origin Oils With Polyphenol Addition. J Food Qual (2010) 33(3):269–82. doi: 10.1111/j.1745-4557.2010.00295.x
88. Aladedunye F, Catel Y, Przybylski R. Novel Caffeic Acid Amide Antioxidants: Synthesis, Radical Scavenging Activity and Performance Under Storage and Frying Conditions. Food Chem (2012) 130(4):945–52. doi: 10.1016/j.foodchem.2011.08.021
89. Luo M, Zhang R-Y, Zheng Z, Wang J-l, Ji J-B. Impact of Some Natural Derivatives on the Oxidative Stability of Soybean Oil Based Biodiesel. J Braz Chem Soc (2012) 23:241–6. doi: 10.1590/S0103-50532012000200008
90. Yamada Y, Yasui H, Sakurai H. Suppressive Effect of Caffeic Acid and its Derivatives on the Generation of UVA-Induced Reactive Oxygen Species in the Skin of Hairless Mice and Pharmacokinetic Analysis on Organ Distribution of Caffeic Acid in ddY Mice. Photochem Photobiol (2006) 82(6):1668–76. doi: 10.1111/j.1751-1097.2006.tb09829.x
91. Scharffetter-Kochanek K, Wlaschek M, Brenneisen P, Schauen M, Blaudschun R, Wenk J. UV-Induced Reactive Oxygen Species in Photocarcinogenesis and Photoaging. Biol Chem (1997) 378(11):1247–58.
92. Matsumura Y, Ananthaswamy HN. Short-Term and Long-Term Cellular and Molecular Events Following UV Irradiation of Skin: Implications for Molecular Medicine. Expert Rev Mol Med (2002) 4(26):1–22. doi: 10.1017/S146239940200532X
93. Prasad NR, Jeyanthimala K, Ramachandran S. Caffeic Acid Modulates Ultraviolet Radiation-B Induced Oxidative Damage in Human Blood Lymphocytes. J Photochem Photobiol B Biol (2009) 95(3):196–203. doi: 10.1016/j.jphotobiol.2009.03.007
94. Saija A, Tomaino A, Trombetta D, Giacchi M, De Pasquale A, Bonina F. Influence of Different Penetration Enhancers on In Vitro Skin Permeation and In Vivo Photoprotective Effect of Flavonoids. Int J Pharm (1998) 175(1):85–94. doi: 10.1016/S0378-5173(98)00259-2
95. Saija A, Tomaino A, Trombetta D, De Pasquale A, Uccella N, Barbuzzi T, et al. In Vitro and In Vivo Evaluation of Caffeic and Ferulic Acids as Topical Photoprotective Agents. Int J Pharm (2000) 199(1):39–47. doi: 10.1016/S0378-5173(00)00358-6
96. Maher P. The Effects of Stress and Aging on Glutathione Metabolism. Ageing Res Rev (2005) 4(2):288–314. doi: 10.1016/j.arr.2005.02.005
97. Devipriya N, Sudheer AR, Menon VP. Caffeic Acid Protects Human Peripheral Blood Lymphocytes Against Gamma Radiation-Induced Cellular Damage. J Biochem Mol Toxicol (2008) 22(3):175–86. doi: 10.1002/jbt.20228
98. Hung C-Y, Yen G-C. Antioxidant Activity of Phenolic Compounds Isolated From Mesona Procumbens Hemsl. J Agric Food Chem (2002) 50(10):2993–7. doi: 10.1021/jf011454y
99. Sato Y, Itagaki S, Kurokawa T, Ogura J, Kobayashi M, Hirano T, et al. In Vitro and In Vivo Antioxidant Properties of Chlorogenic Acid and Caffeic Acid. Int J Pharm (2011) 403(1-2):136–8. doi: 10.1016/j.ijpharm.2010.09.035
100. Guneli E, Cavdar Z, Islekel H, Sarioglu S, Erbayraktar S, Kiray M, et al. Erythropoietin Protects the Intestine Against Ischemia/Reperfusion Injury in Rats. Mol Med (2007) 13(9):509–17. doi: 10.2119/2007-00032.Guneli
101. Guven A, Tunc T, Topal T, Kul M, Korkmaz A, Gundogdu G, et al. α-Lipoic Acid and Ebselen Prevent Ischemia/Reperfusion Injury in the Rat Intestine. Surg Today (2008) 38(11):1029–35. doi: 10.1007/s00595-007-3752-9
102. Feng Y, Lu Y-W, Xu P-H, Long Y, Wu W-M, Li W, et al. Caffeic Acid Phenethyl Ester and its Related Compounds Limit the Functional Alterations of the Isolated Mouse Brain and Liver Mitochondria Submitted to In Vitro Anoxia–Reoxygenation: Relationship to Their Antioxidant Activities. Biochim Biophys Acta (BBA)-Gen Subj (2008) 1780(4):659–72. doi: 10.1016/j.bbagen.2008.01.002
103. Wang X, Stavchansky S, Kerwin SM, Bowman PD. Structure–activity Relationships in the Cytoprotective Effect of Caffeic Acid Phenethyl Ester (CAPE) and Fluorinated Derivatives: Effects on Heme Oxygenase-1 Induction and Antioxidant Activities. Eur J Pharmacol (2010) 635(1-3):16–22. doi: 10.1016/j.ejphar.2010.02.034
104. Lu H, Ouyang W, Huang C. Inflammation, a Key Event in Cancer Development. Mol Cancer Res (2006) 4(4):221–33. doi: 10.1158/1541-7786.MCR-05-0261
105. Alam M, Hasan GM, Hassan MI. A Review on the Role of TANK-Binding Kinase 1 Signaling in Cancer. Int J Biol Macromol (2021) 183:2364–75. doi: 10.1016/j.ijbiomac.2021.06.022
106. Ali S, Alam M, Hasan GM, Hassan MI. Potential Therapeutic Targets of Klebsiella Pneumoniae: A Multi-Omics Review Perspective. Brief Funct Genomics (2021). doi: 10.1093/bfgp/elab038
107. Zhao W-X, Wang L, Yang J-L, Li L-Z, Xu W-M, Li T. Caffeic Acid Phenethyl Ester Attenuates Pro-Inflammatory and Fibrogenic Phenotypes of LPS-Stimulated Hepatic Stellate Cells Through the Inhibition of NF-κb Signaling. Int J Mol Med (2014) 33(3):687–94. doi: 10.3892/ijmm.2013.1613
108. Garcia-Ruiz C, Colell A, Mari M, Morales A, Fernandez-Checa JC. Direct Effect of Ceramide on the Mitochondrial Electron Transport Chain Leads to Generation of Reactive Oxygen Species. Role of Mitochondrial Glutathione. J Biol Chem (1997) 272(17):11369–77. doi: 10.1074/jbc.272.17.11369
109. Hseu YC, Chang WH, Chen CS, Liao JW, Huang CJ, Lu FJ, et al. Antioxidant Activities of Toona Sinensis Leaves Extracts Using Different Antioxidant Models. Food Chem Toxicol (2008) 46(1):105–14. doi: 10.1016/j.fct.2007.07.003
110. Orsolic N, Basic I. Water-Soluble Derivative of Propolis and its Polyphenolic Compounds Enhance Tumoricidal Activity of Macrophages. J Ethnopharmacol (2005) 102(1):37–45. doi: 10.1016/j.jep.2005.05.036
111. Siveen KS, Kuttan G. Role of Macrophages in Tumour Progression. Immunol Lett (2009) 123(2):97–102. doi: 10.1016/j.imlet.2009.02.011
112. Corraliza IM, Campo ML, Soler G, Modolell M. Determination of Arginase Activity in Macrophages: A Micromethod. J Immunol Methods (1994) 174(1-2):231–5. doi: 10.1016/0022-1759(94)90027-2
113. Mantovani A, Sica A. Macrophages, Innate Immunity and Cancer: Balance, Tolerance, and Diversity. Curr Opin Immunol (2010) 22(2):231–7. doi: 10.1016/j.coi.2010.01.009
114. Qian BZ, Pollard JW. Macrophage Diversity Enhances Tumor Progression and Metastasis. Cell (2010) 141(1):39–51. doi: 10.1016/j.cell.2010.03.014
115. Murtaza G, Sajjad A, Mehmood Z, Shah SH, Siddiqi AR. Possible Molecular Targets for Therapeutic Applications of Caffeic Acid Phenethyl Ester in Inflammation and Cancer. J Food Drug Anal (2015) 23(1):11-18.
116. Alam M, Ali S, Ashraf GM, Bilgrami AL, Yadav DK, Hassan MI. Epigallocatechin 3-Gallate: From Green Tea to Cancer Therapeutics. Food Chem (2022) 379:132135. doi: 10.1016/j.foodchem.2022.132135
117. Ito D, Imai Y, Ohsawa K, Nakajima K, Fukuuchi Y, Kohsaka S. Microglia-Specific Localisation of a Novel Calcium Binding Protein, Iba1. Mol Brain Res (1998) 57(1):1–9. doi: 10.1016/S0169-328X(98)00040-0
118. Verri M, Pastoris O, Dossena M, Aquilani R, Guerriero F, Cuzzoni G, et al. Mitochondrial Alterations, Oxidative Stress and Neuroinflammation in Alzheimer's Disease. Int J Immunopathol Pharmacol (2012) 25(2):345–53. doi: 10.1177/039463201202500204
119. Prokop S, Miller KR, Heppner FL. Microglia Actions in Alzheimer’s Disease. Acta Neuropathol (2013) 126(4):461–77. doi: 10.1007/s00401-013-1182-x
120. Chen X-L, Dodd G, Thomas S, Zhang X, Wasserman MA, Rovin BH, et al. Activation of Nrf2/ARE Pathway Protects Endothelial Cells From Oxidant Injury and Inhibits Inflammatory Gene Expression. Am J Physiol Heart Circ Physiol (2006) 290(5):H1862–70. doi: 10.1152/ajpheart.00651.2005
121. Alam M, Ali S, Ahmed S, Elasbali AM, Adnan M, Islam A, et al. Therapeutic Potential of Ursolic Acid in Cancer and Diabetic Neuropathy Diseases. Int J Mol Sci (2021) 22(22):12162. doi: 10.3390/ijms222212162
122. Kapturczak MH, Wasserfall C, Brusko T, Campbell-Thompson M, Ellis TM, Atkinson MA, et al. Heme Oxygenase-1 Modulates Early Inflammatory Responses: Evidence From the Heme Oxygenase-1-Deficient Mouse. Am J Pathol (2004) 165(3):1045–53. doi: 10.1016/S0002-9440(10)63365-2
123. Kim J-K, Jang H-D. Nrf2-Mediated HO-1 Induction Coupled With the ERK Signaling Pathway Contributes to Indirect Antioxidant Capacity of Caffeic Acid Phenethyl Ester in HepG2 Cells. Int J Mol Sci (2014) 15(7):12149–65. doi: 10.3390/ijms150712149
124. Zhou Y, Fang SH, Ye YL, Chu LS, Zhang WP, Wang ML, et al. Caffeic Acid Ameliorates Early and Delayed Brain Injuries After Focal Cerebral Ischemia in Rats 1. Acta Pharmacol Sin (2006) 27(9):1103–10. doi: 10.1111/j.1745-7254.2006.00406.x
125. Lu D-Y, Huang B-R, Yeh W-L, Lin H-Y, Huang S-S, Liu Y-S, et al. Anti-Neuroinflammatory Effect of a Novel Caffeamide Derivative, KS370G, in Microglial Cells. Mol Neurobiol (2013) 48(3):863–74. doi: 10.1007/s12035-013-8474-y
126. Tyszka-Czochara M, Konieczny P, Majka M. Caffeic Acid Expands Anti-Tumor Effect of Metformin in Human Metastatic Cervical Carcinoma HTB-34 Cells: Implications of AMPK Activation and Impairment of Fatty Acids de novo Biosynthesis. Int J Mol Sci (2017) 18(2):462. doi: 10.3390/ijms18020462
127. Bouzaiene NN, Jaziri SK, Kovacic H, Chekir-Ghedira L, Ghedira K, Luis J. The Effects of Caffeic, Coumaric and Ferulic Acids on Proliferation, Superoxide Production, Adhesion and Migration of Human Tumor Cells In Vitro. Eur J Pharmacol (2015) 766:99–105. doi: 10.1016/j.ejphar.2015.09.044
128. Prasad NR, Karthikeyan A, Karthikeyan S, Reddy BV. Inhibitory Effect of Caffeic Acid on Cancer Cell Proliferation by Oxidative Mechanism in Human HT-1080 Fibrosarcoma Cell Line. Mol Cell Biochem (2011) 349(1):11–9. doi: 10.1007/s11010-010-0655-7
129. Weng C-J, Yen G-C. Chemopreventive Effects of Dietary Phytochemicals Against Cancer Invasion and Metastasis: Phenolic Acids, Monophenol, Polyphenol, and Their Derivatives. Cancer Treat Rev (2012) 38(1):76–87. doi: 10.1016/j.ctrv.2011.03.001
130. Kabała-Dzik A, Rzepecka-Stojko A, Kubina R, Jastrzębska-Stojko Ż, Stojko R, Wojtyczka RD, et al. Migration Rate Inhibition of Breast Cancer Cells Treated by Caffeic Acid and Caffeic Acid Phenethyl Ester: An In Vitro Comparison Study. Nutrients (2017) 9(10):1144. doi: 10.3390/nu9101144
131. Rosendahl AH, Perks CM, Zeng L, Markkula A, Simonsson M, Rose C, et al. Caffeine and Caffeic Acid Inhibit Growth and Modify Estrogen Receptor and Insulin-Like Growth Factor I Receptor Levels in Human Breast Cancer. Clin Cancer Res (2015) 21(8):1877–87. doi: 10.1158/1078-0432.CCR-14-1748
132. Rosendahl AH, Perks CM, Markkula A, Simonsson M, Rose CC, Ingvar C, et al. Caffeine and Caffeic Acid Inhibit Growth and Modify Estrogen Receptor (ER)-α and Insulin-Like Growth Factor I Receptor (IGF-IR) Levels in Human Breast Cancer. Clin Cancer Res (2015) 21(8):1877–87. doi: 10.1158/1078-0432.CCR-14-1748
133. Serafim TL, Milhazes N, Borges F, Oliveira PJ. Caffeic and Ferulic Acid Derivatives: Use in Breast Cancer. In: Coffee in Health and Disease Prevention. Elsevier (2015). p. 663–71.
134. Dy GW, Gore JL, Forouzanfar MH, Naghavi M, Fitzmaurice C. Global Burden of Urologic Cancers, 1990–2013. Eur Urol (2017) 71(3):437–46. doi: 10.1016/j.eururo.2016.10.008
135. Fei X, Shen Y, Li X, Guo H. The Association of Tea Consumption and the Risk and Progression of Prostate Cancer: A Meta-Analysis. Int J Clin Exp Med (2014) 7(11):3881.
136. Liu H, Hu G-H, Wang X-C, Huang T-B, Xu L, Lai P, et al. Coffee Consumption and Prostate Cancer Risk: A Meta-Analysis of Cohort Studies. Nutr Cancer (2015) 67(3):392–400. doi: 10.1080/01635581.2015.1004727
137. Russo GI, Campisi D, Di Mauro M, Regis F, Reale G, Marranzano M, et al. Dietary Consumption of Phenolic Acids and Prostate Cancer: A Case-Control Study in Sicily, Southern Italy. Molecules (2017) 22(12):2159. doi: 10.3390/molecules22122159
138. Lin H-P, Lin C-Y, Huo C, Hsiao P-H, Su L-C, Jiang SS, et al. Caffeic Acid Phenethyl Ester Induced Cell Cycle Arrest and Growth Inhibition in Androgen-Independent Prostate Cancer Cells via Regulation of Skp2, p53, p21Cip1 and p27Kip1. Oncotarget (2015) 6(9):6684. doi: 10.18632/oncotarget.3246
139. Natarajan K, Singh S, Burke TR, Grunberger D, Aggarwal BB. Caffeic Acid Phenethyl Ester is a Potent and Specific Inhibitor of Activation of Nuclear Transcription Factor NF-Kappa B. Proc Natl Acad Sci (1996) 93(17):9090–5. doi: 10.1073/pnas.93.17.9090
140. Siegel R, Ma J, Zou Z, Jemal A. Cancer Statistics, 2014. CA Cancer J Clin (2014) 64:9–29. doi: 10.3322/caac.21208
141. Jemal A, Bray F, Center MM, Ferlay J, Ward E, Forman D. Global Cancer Statistics. CA Cancer J Clin (2011) 61(2):69–90. doi: 10.3322/caac.20107
142. Scheff RJ, Schneider BJ. In Non–Small-Cell Lung Cancer: Treatment of Late Stage Disease: Chemotherapeutics and New Frontiers. Semin Intervent Radiol (2013) 30(2):191–8. doi: 10.1055/s-0033-1342961
143. Lin C-L, Chen R-F, Chen JY-F, Chu Y-C, Wang H-M, Chou H-L, et al. Protective Effect of Caffeic Acid on Paclitaxel Induced Anti-Proliferation and Apoptosis of Lung Cancer Cells Involves NF-κB Pathway. Int J Mol Sci (2012) 13(5):6236–45. doi: 10.3390/ijms13056236
144. Min J, Shen H, Xi W, Wang Q, Yin L, Zhang Y, et al. Synergistic Anticancer Activity of Combined Use of Caffeic Acid With Paclitaxel Enhances Apoptosis of Non-Small-Cell Lung Cancer H1299 Cells In Vivo and In Vitro. Cell Physiol Biochem (2018) 48(4):1433–42. doi: 10.1159/000492253
145. Shigeoka Y, Igishi T, Matsumoto S, Nakanishi H, Kodani M, Yasuda K, et al. Sulindac Sulfide and Caffeic Acid Phenethyl Ester Suppress the Motility of Lung Adenocarcinoma Cells Promoted by Transforming Growth Factor-β Through Akt Inhibition. J Cancer Res Clin Oncol (2004) 130(3):146–52. doi: 10.1007/s00432-003-0520-0
146. Alam M, Mishra R. Role of PI3K and EGFR in Oral Cancer Progression and Drug Resistance. Int J Res Appl Sci Biotechnol (2020) 7(6):85–9. doi: 10.31033/ijrasb.7.6.14
147. Jaganathan SK. Growth Inhibition by Caffeic Acid, One of the Phenolic Constituents of Honey, in HCT 15 Colon Cancer Cells. Sci World J (2012) 2012:372345. doi: 10.1100/2012/372345
148. Pelinson LP, Assmann CE, Palma TV, da Cruz IBM, Pillat MM, Mânica A, et al. Antiproliferative and Apoptotic Effects of Caffeic Acid on SK-Mel-28 Human Melanoma Cancer Cells. Mol Biol Rep (2019) 46(2):2085–92. doi: 10.1007/s11033-019-04658-1
149. Maruyama H, Kawakami F, Lwin T-T, Imai M, Shamsa F. Biochemical Characterization of Ferulic Acid and Caffeic Acid Which Effectively Inhibit Melanin Synthesis via Different Mechanisms in B16 Melanoma Cells. Biol Pharm Bull (2018) 41(5):806–10. doi: 10.1248/bpb.b17-00892
150. Chen C-N, Wu C-L, Lin J-K. Apoptosis of Human Melanoma Cells Induced by the Novel Compounds Propolin A and Propolin B From Taiwenese Propolis. Cancer Lett (2007) 245(1-2):218–31. doi: 10.1016/j.canlet.2006.01.016
151. Mascolo M, Siano M, Ilardi G, Russo D, Merolla F, Rosa GD, et al. Epigenetic Disregulation in Oral Cancer. Int J Mol Sci (2012) 13(2):2331–53. doi: 10.3390/ijms13022331
152. Alam M, Kashyap T, Pramanik KK, Singh AK, Nagini S, Mishra R. The Elevated Activation of Nfκb and AP-1 is Correlated With Differential Regulation of Bcl-2 and Associated With Oral Squamous Cell Carcinoma Progression and Resistance. Clin Oral Investig (2017) 21(9):2721–31. doi: 10.1007/s00784-017-2074-6
153. Pramanik KK, Singh AK, Alam M, Kashyap T, Mishra P, Panda AK, et al. Reversion-Inducing Cysteine-Rich Protein With Kazal Motifs and its Regulation by Glycogen Synthase Kinase 3 Signaling in Oral Cancer. Tumor Biol (2016) 37(11):15253–64. doi: 10.1007/s13277-016-5362-x
154. Alam M, Kashyap T, Mishra P, Panda AK, Nagini S, Mishra R. Role and Regulation of Proapoptotic Bax in Oral Squamous Cell Carcinoma and Drug Resistance. Head Neck (2019) 41(1):185–97. doi: 10.1002/hed.25471
155. Alam M, Mishra R. Bcl-xL Expression and Regulation in the Progression, Recurrence, and Cisplatin Resistance of Oral Cancer. Life Sci (2021) 280:119705. doi: 10.1016/j.lfs.2021.119705
156. Alam M, Ali S, Mohammad T, Hasan GM, Yadav DK, Hassan M. B Cell Lymphoma 2: A Potential Therapeutic Target for Cancer Therapy. Int J Mol Sci (2021) 22(19):10442. doi: 10.3390/ijms221910442
157. Dziedzic A, Kubina R, Kabała-Dzik A, Wojtyczka RD, Morawiec T, Bułdak RJ. Caffeic Acid Reduces the Viability and Migration Rate of Oral Carcinoma Cells (SCC-25) Exposed to Low Concentrations of Ethanol. Int J Mol Sci (2014) 15(10):18725–41. doi: 10.3390/ijms151018725
158. Michaluart P, Masferrer JL, Carothers AM, Subbaramaiah K, Zweifel BS, Koboldt C, et al. Inhibitory Effects of Caffeic Acid Phenethyl Ester on the Activity and Expression of Cyclooxygenase-2 in Human Oral Epithelial Cells and in a Rat Model of Inflammation. Cancer Res (1999) 59(10):2347–52.
159. Kuo Y-Y, Jim W-T, Su L-C, Chung C-J, Lin C-Y, Huo C, et al. Caffeic Acid Phenethyl Ester is a Potential Therapeutic Agent for Oral Cancer. Int J Mol Sci (2015) 16(5):10748–66. doi: 10.3390/ijms160510748
160. McGlynn KA, Petrick JL, London WT. Global Epidemiology of Hepatocellular Carcinoma: An Emphasis on Demographic and Regional Variability. Clin Liver Dis (2015) 19(2):223–38. doi: 10.1016/j.cld.2015.01.001
161. França AVC, Elias Júnior J, Lima BLGd, Martinelli AL, Carrilho FJ. Diagnosis, Staging and Treatment of Hepatocellular Carcinoma. Braz J Med Biol Res (2004) 37:1689–705. doi: 10.1590/S0100-879X2004001100015
162. Silva T, Oliveira C, Borges F. Caffeic Acid Derivatives, Analogs and Applications: A Patent Review (2009–2013). Expert Opin Ther Pat (2014) 24(11):1257–70. doi: 10.1517/13543776.2014.959492
163. Sidoryk K, Jaromin A, Filipczak N, Cmoch P, Cybulski M. Synthesis and Antioxidant Activity of Caffeic Acid Derivatives. Molecules (2018) 23(9):2199. doi: 10.3390/molecules23092199
164. Pramanik KK, Nagini S, Singh AK, Mishra P, Kashyap T, Nath N, et al. Glycogen Synthase Kinase-3β Mediated Regulation of Matrix Metalloproteinase-9 and its Involvement in Oral Squamous Cell Carcinoma Progression and Invasion. Cell Oncol (2018) 41(1):47–60. doi: 10.1007/s13402-017-0358-0
165. Brautigan DL, Gielata M, Heo J, Kubicka E, Wilkins LR. Selective Toxicity of Caffeic Acid in Hepatocellular Carcinoma Cells. Biochem Biophys Res Commun (2018) 505(2):612–7. doi: 10.1016/j.bbrc.2018.09.155
166. Yang S-Y, Pyo MC, Nam M-H, Lee K-W. ERK/Nrf2 Pathway Activation by Caffeic Acid in HepG2 Cells Alleviates its Hepatocellular Damage Caused by T-Butylhydroperoxide-Induced Oxidative Stress. BMC Complement Altern Med (2019) 19(1):1–13. doi: 10.1186/s12906-019-2551-3
167. Kanimozhi G, Prasad N. Anticancer Effect of Caffeic Acid on Human Cervical Cancer Cells. In: Coffee in Health and Disease Prevention. Elsevier (2015). p. 655–61.
168. León-González AJ, Auger C, Schini-Kerth VB. Pro-Oxidant Activity of Polyphenols and its Implication on Cancer Chemoprevention and Chemotherapy. Biochem Pharmacol (2015) 98(3):371–80. doi: 10.1016/j.bcp.2015.07.017
169. Lau AT, Wang Y, Chiu JF. Reactive Oxygen Species: Current Knowledge and Applications in Cancer Research and Therapeutic. J Cell Biochem (2008) 104(2):657–67. doi: 10.1002/jcb.21655
170. Chang W-C, Hsieh C-H, Hsiao M-W, Lin W-C, Hung Y-C, Ye J-C. Caffeic Acid Induces Apoptosis in Human Cervical Cancer Cells Through the Mitochondrial Pathway. Taiwan J Obstet Gynecol (2010) 49(4):419–24. doi: 10.1016/S1028-4559(10)60092-7
171. Hemaiswarya S, Doble M. Combination of Phenylpropanoids With 5-Fluorouracil as Anti-Cancer Agents Against Human Cervical Cancer (HeLa) Cell Line. Phytomedicine (2013) 20(2):151–8. doi: 10.1016/j.phymed.2012.10.009
172. Koraneekit A, Limpaiboon T, Sangka A, Boonsiri P, Daduang S, Daduang J. Synergistic Effects of Cisplatin-Caffeic Acid Induces Apoptosis in Human Cervical Cancer Cells via the Mitochondrial Pathways. Oncol Lett (2018) 15(5):7397–402. doi: 10.3892/ol.2018.8256
173. Guffey CR. Linking Obesity to Colorectal Cancer: Recent Insights Into Plausible Biological Mechanisms. Curr Opin Clin Nutr Metab Care (2013) 16(5):595–600. doi: 10.1097/MCO.0b013e328362d10b
174. Fung TT, Hu FB, Schulze M, Pollak M, Wu T, Fuchs CS, et al. A Dietary Pattern That is Associated With C-Peptide and Risk of Colorectal Cancer in Women. Cancer Causes Control (2012) 23(6):959–65. doi: 10.1007/s10552-012-9969-y
175. Dik VK, Bueno-de-Mesquita HB, Van Oijen MG, Siersema PD, Uiterwaal CS, Van Gils CH, et al. Coffee and Tea Consumption, Genotype-Based CYP1A2 and NAT2 Activity and Colorectal Cancer Risk—Results From the EPIC Cohort Study. Int J Cancer (2014) 135(2):401–12. doi: 10.1016/S0016-5085(13)62173-3
176. Chiang E-PI, Tsai S-Y, Kuo Y-H, Pai M-H, Chiu H-L, Rodriguez RL, et al. Caffeic Acid Derivatives Inhibit the Growth of Colon Cancer: Involvement of the PI3-K/Akt and AMPK Signaling Pathways. PloS One (2014) 9(6):e99631. doi: 10.1371/journal.pone.0099631
177. Li G, Ma D, Zhang Y, Zheng W, Wang P. Coffee Consumption and Risk of Colorectal Cancer: A Meta-Analysis of Observational Studies. Public Health Nutr (2013) 16(2):346–57. doi: 10.1017/S1368980012002601
178. Chiang H-M, Chen C-W, Chen C-C, Wang H-W, Jhang J-H, Huang Y-H, et al. Role of Coffea Arabica Extract and Related Compounds in Preventing Photoaging and Photodamage of the Skin. In: Coffee in Health and Disease Prevention. Elsevier (2015). p. 523–30.
179. Pluemsamran T, Tripatara P, Phadungrakwittaya R, Akarasereenont P, Laohapand T, Panich U. Redox Mechanisms of AVS022, an Oriental Polyherbal Formula, and its Component Herbs in Protection Against Induction of Matrix Metalloproteinase-1 in UVA-Irradiated Keratinocyte HaCaT Cells. Evid Based Complement Alternat Med (2013) 2013:739473. doi: 10.1155/2013/739473
180. Ryšavá A, Vostálová J, Rajnochova Svobodova A. Effect of Ultraviolet Radiation on the Nrf2 Signaling Pathway in Skin Cells. Int J Radiat Biol (2021) 97(10):1383–403. doi: 10.1080/09553002.2021.1962566
181. Zhang M, Hwang E, Lin P, Gao W, Ngo HT, Yi T-H, et al. Exerts a Protective Effect Against Extrinsic Aging Through NF-κb, MAPKs, AP-1, and TGF-β/Smad Signaling Pathways in UVB-Aged Normal Human Dermal Fibroblasts. Rejuvenation Res (2018) 21(4):313–22. doi: 10.1089/rej.2017.1971
182. Kuo Y-H, Chen C-W, Chu Y, Lin P, Chiang H-M. In Vitro and In Vivo Studies on Protective Action of N-Phenethyl Caffeamide Against Photodamage of Skin. PloS One (2015) 10(9):e0136777. doi: 10.1371/journal.pone.0136777
183. Balupillai A, Prasad RN, Ramasamy K, Muthusamy G, Shanmugham M, Govindasamy K, et al. Caffeic Acid Inhibits UVB-Induced Inflammation and Photocarcinogenesis Through Activation of Peroxisome Proliferator-Activated Receptor-γ in Mouse Skin. Photochem Photobiol (2015) 91(6):1458–68. doi: 10.1111/php.12522
184. Mokhtari RB, Homayouni TS, Baluch N, Morgatskaya E, Kumar S, Das B, et al. Combination Therapy in Combating Cancer. Oncotarget (2017) 8(23):38022. doi: 10.18632/oncotarget.16723
185. Chen X, Ren B, Chen M, Wang Q, Zhang L, Yan G. NLLSS: Predicting Synergistic Drug Combinations Based on Semi-Supervised Learning. PloS Comput Biol (2016) 12(7):e1004975. doi: 10.1371/journal.pcbi.1004975
186. Bukowska B, Gajek A, Marczak A. Two Drugs are Better Than One. A Short History of Combined Therapy of Ovarian Cancer. Contemp Oncol (2015) 19(5):350. doi: 10.5114/wo.2014.43975
187. Das P, Delost MD, Qureshi MH, Smith DT, Njardarson JT. A Survey of the Structures of US FDA Approved Combination Drugs. J Med Chem (2018) 62(9):4265–311. doi: 10.1021/acs.jmedchem.8b01610
188. Sirota R, Gibson D, Kohen R. The Timing of Caffeic Acid Treatment With Cisplatin Determines Sensitization or Resistance of Ovarian Carcinoma Cell Lines. Redox Biol (2017) 11:170–5. doi: 10.1016/j.redox.2016.12.006
189. Tyszka-Czochara M, Bukowska-Strakova K, Majka M. Metformin and Caffeic Acid Regulate Metabolic Reprogramming in Human Cervical Carcinoma SiHa/HTB-35 Cells and Augment Anticancer Activity of Cisplatin via Cell Cycle Regulation. Food Chem Toxicol (2017) 106:260–72. doi: 10.1016/j.fct.2017.05.065
190. Lee J, Kim KS, Na K. Caffeic Acid-Coated Multifunctional Magnetic Nanoparticles for the Treatment and Bimodal Imaging of Tumours. J Photochem Photobiol B Biol (2016) 160:210–6. doi: 10.1016/j.jphotobiol.2016.03.058
191. Lee SJ, Choi K-C, Kang M-S, Oh J-S, Jeong Y-I, Lee HC. Self-Organized Nanoparticles of Caffeic Acid Conjugated Polysaccharide and its Anticancer Activity. J Nanosci Nanotechnol (2015) 15(2):1130–4. doi: 10.1166/jnn.2015.9312
192. Mehtap Kutlu H, Genc L, Guney G. The Impact of Caffeic Acid Loaded Solid Lipid Nanoparticles on Cancer Treatment. Curr Nanosci (2013) 9(6):698–703. doi: 10.2174/15734137113099990077
193. Mudgal J, Mallik SB, Nampoothiri M, Kinra M, Hall S, Grant GD, et al. Effect of Coffee Constituents, Caffeine and Caffeic Acid on Anxiety and Lipopolysaccharide-Induced Sickness Behavior in Mice. J Funct Foods (2020) 64:103638. doi: 10.1016/j.jff.2019.103638
194. Da Silveira C. C. S. D. M., Fernandes LMP, Silva ML, Luz DA, Gomes ARQ, Monteiro MC, et al. Neurobehavioral and Antioxidant Effects of Ethanolic Extract of Yellow Propolis. Oxid Med Cell Longev (2016) 2016:2906953. doi: 10.1155/2016/2906953
195. Takeda H, Tsuji M, Yamada T, Masuya J, Matsushita K, Tahara M, et al. Caffeic Acid Attenuates the Decrease in Cortical BDNF mRNA Expression Induced by Exposure to Forced Swimming Stress in Mice. Eur J Pharmacol (2006) 534(1-3):115–21. doi: 10.1016/j.ejphar.2006.01.026
196. Wang R, Li Y-B, Li Y-H, Xu Y, Wu H-L, Li X-J. Curcumin Protects Against Glutamate Excitotoxicity in Rat Cerebral Cortical Neurons by Increasing Brain-Derived Neurotrophic Factor Level and Activating TrkB. Brain Res (2008) 1210:84–91. doi: 10.1016/j.brainres.2008.01.104
197. dos Santos Sales ÍM, Do Nascimento KG, Feitosa CM, Saldanha GB, Feng D, de Freitas RM. Caffeic Acid Effects on Oxidative Stress in Rat Hippocampus After Pilocarpine-Induced Seizures. Neurological Sci (2011) 32(3):375–80. doi: 10.1007/s10072-010-0420-4
198. Socała K, Szopa A, Serefko A, Poleszak E, Wlaź P. Neuroprotective Effects of Coffee Bioactive Compounds: A Review. Int J Mol Sci (2021) 22(1):107. doi: 10.3390/ijms22010107
199. Fontanilla C, Ma Z, Wei X, Klotsche J, Zhao L, Wisniowski P, et al. Caffeic Acid Phenethyl Ester Prevents 1-Methyl-4-Phenyl-1, 2, 3, 6-Tetrahydropyridine-Induced Neurodegeneration. Neuroscience (2011) 188:135–41. doi: 10.1016/j.neuroscience.2011.04.009
200. Tsai S-j, Chao C-y, Yin M-c. Preventive and Therapeutic Effects of Caffeic Acid Against Inflammatory Injury in Striatum of MPTP-Treated Mice. Eur J Pharmacol (2011) 670(2-3):441–7. doi: 10.1016/j.ejphar.2011.09.171
201. Tian X-F, Pu X-P. Caffeic Acid (CA) Protects Cerebellar Granule Neurons (CGNs) From Apoptosis Induced by Neurotoxin 1-Methyl-4-Phenylpyridnium (MPP+). Beijing Da Xue Xue Bao Yi Xue Ban (2004) 36(1):27–30.
202. Gürbüz P, Dokumacı AH, Gündüz MG, Perez C, Göger F, Paksoy MY, et al. In Vitro Biological Activity of Salvia Fruticosa Mill. Infusion Against Amyloid β-Peptide-Induced Toxicity and Inhibition of GSK-3β, CK-1δ, and BACE-1 Enzymes Relevant to Alzheimer's Disease. Saudi Pharm J (2021) 29(3):236–43. doi: 10.1016/j.jsps.2021.01.007
203. Taram F, Winter AN, Linseman DA. Neuroprotection Comparison of Chlorogenic Acid and its Metabolites Against Mechanistically Distinct Cell Death-Inducing Agents in Cultured Cerebellar Granule Neurons. Brain Res (2016) 1648(Pt A):69–80. doi: 10.1016/j.brainres.2016.07.028
204. Yang JQ, Zhou QX, Liu BZ, He BC. Protection of Mouse Brain From Aluminum-Induced Damage by Caffeic Acid. CNS Neurosci Ther (2008) 14(1):10–6. doi: 10.1111/j.1755-5949.2007.00031.x
205. Anwar J, Spanevello RM, Thome G, Stefanello N, Schmatz R, Gutierres J, et al. Effects of Caffeic Acid on Behavioral Parameters and on the Activity of Acetylcholinesterase in Different Tissues From Adult Rats. Pharmacol Biochem Behav (2012) 103(2):386–94. doi: 10.1016/j.pbb.2012.09.006
206. Khan KA, Kumar N, Nayak PG, Nampoothiri M, Shenoy RR, Krishnadas N, et al. Impact of Caffeic Acid on Aluminium Chloride-Induced Dementia in Rats. J Pharm Pharmacol (2013) 65(12):1745–52. doi: 10.1111/jphp.12126
207. Deshmukh R, Kaundal M, Bansal V. Caffeic Acid Attenuates Oxidative Stress, Learning and Memory Deficit in Intra-Cerebroventricular Streptozotocin Induced Experimental Dementia in Rats. Biomed Pharmacother (2016) 81:56–62. doi: 10.1016/j.biopha.2016.03.017
208. Uz T, Pesold C, Longone P, Manev H. Aging-Associated Up-Regulation of Neuronal 5-Lipoxygenase Expression: Putative Role in Neuronal Vulnerability. FASEB J (1998) 12(6):439–49. doi: 10.1096/fasebj.12.6.439
209. Kalonia H, Kumar P, Kumar A, Nehru B. Effects of Caffeic Acid, Rofecoxib, and Their Combination Against Quinolinic Acid-Induced Behavioral Alterations and Disruption in Glutathione Redox Status. Neurosci Bull (2009) 25(6):343–52. doi: 10.1007/s12264-009-0513-3
210. Coelho VR, Vieira CG, de Souza LP, da Silva LL, Pflüger P, Regner GG, et al. Behavioral and Genotoxic Evaluation of Rosmarinic and Caffeic Acid in Acute Seizure Models Induced by Pentylenetetrazole and Pilocarpine in Mice. Naunyn Schmiedebergs Arch Pharmacol (2016) 389(11):1195–203. doi: 10.1007/s00210-016-1281-z
211. Song Y, Bei Y, Xiao Y, Tong H-D, Wu X-Q, Chen M-T. Edaravone, a Free Radical Scavenger, Protects Neuronal Cells’ Mitochondria From Ischemia by Inactivating Another New Critical Factor of the 5-Lipoxygenase Pathway Affecting the Arachidonic Acid Metabolism. Brain Res (2018) 1690:96–104. doi: 10.1016/j.brainres.2018.03.006
212. Miyazaki I, Isooka N, Wada K, Kikuoka R, Kitamura Y, Asanuma M. Effects of Enteric Environmental Modification by Coffee Components on Neurodegeneration in Rotenone-Treated Mice. Cells (2019) 8(3):221. doi: 10.3390/cells8030221
213. Li Z, Choi D-Y, Shin E-J, Hunter RL, Jin CH, Wie M-B, et al. Phenidone Protects the Nigral Dopaminergic Neurons From LPS-Induced Neurotoxicity. Neurosci Lett (2008) 445(1):1–6. doi: 10.1016/j.neulet.2008.08.053
214. Kovacs GG. Molecular Pathology of Neurodegenerative Diseases: Principles and Practice. J Clin Pathol (2019) 72(11):725–35. doi: 10.1136/jclinpath-2019-205952
215. Gokcen BB, Sanlier N. Coffee Consumption and Disease Correlations. Crit Rev Food Sci Nutr (2019) 59(2):336–48. doi: 10.1080/10408398.2017.1369391
216. Trevitt J, Kawa K, Jalali A, Larsen C. Differential Effects of Adenosine Antagonists in Two Models of Parkinsonian Tremor. Pharmacol Biochem Behav (2009) 94(1):24–9. doi: 10.1016/j.pbb.2009.07.001
217. de Mendonca A, Cunha RA. Therapeutic Opportunities for Caffeine in Alzheimer's Disease and Other Neurodegenerative Disorders. J Alzheimers Dis (2010) 20 Suppl 1:S1–2. doi: 10.3233/JAD-2010-01420
218. Olazarán J, Reisberg B, Clare L, Cruz I, Peña-Casanova J, Del Ser T, et al. Nonpharmacological Therapies in Alzheimer’s Disease: A Systematic Review of Efficacy. Dement Geriatr Cogn Disord (2010) 30(2):161–78. doi: 10.1159/000316119
219. Wortmann M. Dementia: A Global Health Priority - Highlights From an ADI and World Health Organization Report. Alzheimers Res Ther (2012) 4(5):40. doi: 10.1186/alzrt143
220. Cummings JL, Back C. The Cholinergic Hypothesis of Neuropsychiatric Symptoms in Alzheimer's Disease. Am J Geriatr Psychiatry (1998) 6(2 Suppl 1):S64–78. doi: 10.1097/00019442-199821001-00009
221. Whitehouse PJ, Price DL, Struble RG, Clark AW, Coyle JT, Delon MR. Alzheimer's Disease and Senile Dementia: Loss of Neurons in the Basal Forebrain. Science (1982) 215(4537):1237–9. doi: 10.1126/science.7058341
222. Habtemariam S. Protective Effects of Caffeic Acid and the Alzheimer's Brain: An Update. Mini Rev Med Chem (2017) 17(8):667–74. doi: 10.2174/1389557516666161130100947
223. Wang Y, Li J, Hua L, Han B, Zhang Y, Yang X, et al. Effects of Caffeic Acid on Learning Deficits in a Model of Alzheimer's Disease. Int J Mol Med (2016) 38(3):869–75. doi: 10.3892/ijmm.2016.2683
224. Pinheiro Fernandes FD, Fontenele Menezes AP, de Sousa Neves JC, Fonteles AA, da Silva AT, de Araujo Rodrigues P, et al. Caffeic Acid Protects Mice From Memory Deficits Induced by Focal Cerebral Ischemia. Behav Pharmacol (2014) 25(7):637–47. doi: 10.1097/FBP.0000000000000076
225. Chang W, Huang D, Lo YM, Tee Q, Kuo P, Wu JS, et al. Protective Effect of Caffeic Acid Against Alzheimer's Disease Pathogenesis via Modulating Cerebral Insulin Signaling, Beta-Amyloid Accumulation, and Synaptic Plasticity in Hyperinsulinemic Rats. J Agric Food Chem (2019) 67(27):7684–93. doi: 10.1021/acs.jafc.9b02078
226. Zhao T, Ding K-M, Zhang L, Cheng X-M, Wang C-H, Wang Z-T. Acetylcholinesterase and Butyrylcholinesterase Inhibitory Activities of β-Carboline and Quinoline Alkaloids Derivatives From the Plants of Genus Peganum. J Chem (2013) 2016:2906953. doi: 10.1155/2013/717232
227. Oboh G, Agunloye OM, Akinyemi AJ, Ademiluyi AO, Adefegha SA. Comparative Study on the Inhibitory Effect of Caffeic and Chlorogenic Acids on Key Enzymes Linked to Alzheimer’s Disease and Some Pro-Oxidant Induced Oxidative Stress in Rats’ Brain-In Vitro. Neurochem Res (2013) 38(2):413–9. doi: 10.1007/s11064-012-0935-6
228. Maity S, Kinra M, Nampoothiri M, Arora D, Pai K, Mudgal J. Caffeic Acid, A Dietary Polyphenol, as a Promising Candidate for Combination Therapy. Chem Pap (2022) 76:1271–83.
229. Akomolafe S, Akinyemi A, Ogunsuyi O, Oyeleye S, Oboh G, Adeoyo O, et al. Effect of Caffeine, Caffeic Acid and Their Various Combinations on Enzymes of Cholinergic, Monoaminergic and Purinergic Systems Critical to Neurodegeneration in Rat Brain—In Vitro. NeuroToxicology (2017) 62:6–13. doi: 10.1016/j.neuro.2017.04.008
230. Dorsey ER, Elbaz A, Nichols E, Abbasi N, Abd-Allah F, Abdelalim A, et al. Global, Regional, and National Burden of Parkinson's Disease, 1990-2016: A Systematic Analysis for the Global Burden of Disease Study 2016. Lancet Neurol (2018) 17(11):939–53. doi: 10.1016/S1474-4422(18)30295-3
231. Bagheri H, Damase-Michel C, Lapeyre-Mestre M, Cismondo S, O'Connell D, Senard JM, et al. A Study of Salivary Secretion in Parkinson's Disease. Clin Neuropharmacol (1999) 22(4):213–5.
232. Park JH, Kang YJ, Horak FB. What Is Wrong With Balance in Parkinson's Disease? J Mov Disord (2015) 8(3):109–14. doi: 10.14802/jmd.15018
233. Brown RG, Landau S, Hindle JV, Playfer J, Samuel M, Wilson KC, et al. Depression and Anxiety Related Subtypes in Parkinson's Disease. J Neurol Neurosurg Psychiatry (2011) 82(7):803–9. doi: 10.1136/jnnp.2010.213652
234. Hardoff R, Sula M, Tamir A, Soil A, Front A, Badarna S, et al. Gastric Emptying Time and Gastric Motility in Patients With Parkinson's Disease. Mov Disord (2001) 16(6):1041–7. doi: 10.1002/mds.1203
235. Fazili NA, Naeem A. Anti-Fibrillation Potency of Caffeic Acid Against an Antidepressant Induced Fibrillogenesis of Human Alpha-Synuclein: Implications for Parkinson's Disease. Biochimie (2015) 108:178–85. doi: 10.1016/j.biochi.2014.11.011
236. Tsai SJ, Chao CY, Yin MC. Corrigendum to: "Preventive and Therapeutic Effects of Caffeic Acid Against Inflammatory Injury in Striatum of MPTP-Treated Mice": [Eur.J.Pharmacol.670(2011)441-447]. Eur J Pharmacol (2018) 819:293. doi: 10.1016/j.ejphar.2017.12.048
237. Moosavi F, Hosseini R, Rajaian H, Silva T, Magalhaes ESD, Saso L, et al. Derivatives of Caffeic Acid, a Natural Antioxidant, as the Basis for the Discovery of Novel Nonpeptidic Neurotrophic Agents. Bioorg Med Chem (2017) 25(12):3235–46. doi: 10.1016/j.bmc.2017.04.026
238. Zaitone SA, Ahmed E, Elsherbiny NM, Mehanna ET, El-Kherbetawy MK, ElSayed MH, et al. Caffeic Acid Improves Locomotor Activity and Lessens Inflammatory Burden in a Mouse Model of Rotenone-Induced Nigral Neurodegeneration: Relevance to Parkinson's Disease Therapy. Pharmacol Rep (2019) 71(1):32–41. doi: 10.1016/j.pharep.2018.08.004
239. Liu K, Yu D, Cho Y-Y, Bode AM, Ma W, Yao K, et al. Sunlight UV-Induced Skin Cancer Relies Upon Activation of the P38α Signaling Pathway. Cancer Res (2013) 73(7):2181–8. doi: 10.1158/0008-5472.CAN-12-3408
240. F'guyer S, Afaq F, Mukhtar H. Photochemoprevention of Skin Cancer by Botanical Agents. Photodermatol Photoimmunol Photomede (2003) 19(2):56–72. doi: 10.1034/j.1600-0781.2003.00019.x
241. Kang NJ, Lee KW, Shin BJ, Jung SK, Hwang MK, Bode AM, et al. Caffeic Acid, a Phenolic Phytochemical in Coffee, Directly Inhibits Fyn Kinase Activity and UVB-Induced COX-2 Expression. Carcinogenesis (2009) 30(2):321–30. doi: 10.1093/carcin/bgn282
242. Kang NJ, Shin SH, Lee HJ, Lee KW. Polyphenols as Small Molecular Inhibitors of Signaling Cascades in Carcinogenesis. Pharmacol Ther (2011) 130(3):310–24. doi: 10.1016/j.pharmthera.2011.02.004
243. Kim J-E, Kwon JY, Seo SK, Son JE, Jung SK, Min SY, et al. Cyanidin Suppresses Ultraviolet B-Induced COX-2 Expression in Epidermal Cells by Targeting MKK4, MEK1, and Raf-1. Biochem Pharmacol (2010) 79(10):1473–82. doi: 10.1016/j.bcp.2010.01.008
244. Kwon JY, Lee KW, Kim J-E, Jung SK, Kang NJ, Hwang MK, et al. Delphinidin Suppresses Ultraviolet B-Induced Cyclooxygenases-2 Expression Through Inhibition of MAPKK4 and PI-3 Kinase. Carcinogenesis (2009) 30(11):1932–40. doi: 10.1093/carcin/bgp216
245. Bode AM, Dong Z. Molecular Targets of Coffee Phytochemicals Caffeic Acid and Chlorogenic Acid in Chemoprevention. In: Coffee in Health and Disease Prevention. Elsevier (2015). p. 673–80.
246. Troppmair J, Bruder J, Munoz H, Lloyd PA, Kyriakis J, Banerjee P, et al. Mitogen-Activated Protein Kinase/Extracellular Signal-Regulated Protein Kinase Activation by Oncogenes, Serum, and 12-O-Tetradecanoylphorbol-13-Acetate Requires Raf and is Necessary for Transformation. J Biol Chem (1994) 269(9):7030–5. doi: 10.1016/S0021-9258(17)37478-1
247. Mirzaei S, Gholami MH, Zabolian A, Saleki H, Farahani MV, Hamzehlou S, et al. Caffeic Acid and its Derivatives as Potential Modulators of Oncogenic Molecular Pathways: New Hope in the Fight Against Cancer. Pharmacol Res (2021) 171:105759. doi: 10.1016/j.phrs.2021.105759
248. Voisin L, Julien C, Duhamel S, Gopalbhai K, Claveau I, Saba-El-Leil MK, et al. Activation of MEK1 or MEK2 Isoform is Sufficient to Fully Transform Intestinal Epithelial Cells and Induce the Formation of Metastatic Tumors. BMC Cancer (2008) 8(1):1–17. doi: 10.1186/1471-2407-8-337
249. Roberts PJ, Der CJ. Targeting the Raf-MEK-ERK Mitogen-Activated Protein Kinase Cascade for the Treatment of Cancer. Oncogene (2007) 26(22):3291–310. doi: 10.1038/sj.onc.1210422
250. Zykova TA, Zhu F, Lu C, Higgins L, Tatsumi Y, Abe Y, et al. Lymphokine-Activated Killer T-Cell-Originated Protein Kinase Phosphorylation of Histone H2AX Prevents Arsenite-Induced Apoptosis in RPMI7951 Melanoma Cells. Clin Cancer Res (2006) 12(23):6884–93. doi: 10.1158/1078-0432.CCR-06-0410
251. Gaascht F, Dicato M, Diederich M. Coffee Provides a Natural Multitarget Pharmacopeia Against the Hallmarks of Cancer. Genes Nutr (2015) 10(6):1–17. doi: 10.1007/s12263-015-0501-3
252. Das PR, Eun J-B. Phenolic Acids in Tea and Coffee and Their Health Benefits. In: Phenolic Acids: Properties, Food Sources and Health Effects. Hauppauge, NY, USA: Nova Science Publisher (2016).
253. Truong V-L, Kong AN, Jeong W-S. Red Ginseng Oil Inhibits TPA-Induced Transformation of Skin Epidermal JB6 Cells. J Med Food (2018) 21(4):380–9. doi: 10.1089/jmf.2017.4082
254. Bode AM, Dong Z. Signal Transduction and Molecular Targets of Selected Flavonoids. Antioxid Redox Signal (2013) 19(2):163–80. doi: 10.1089/ars.2013.5251
255. Tewari D, Nabavi SF, Nabavi SM, Sureda A, Farooqi AA, Atanasov AG, et al. Targeting Activator Protein 1 Signaling Pathway by Bioactive Natural Agents: Possible Therapeutic Strategy for Cancer Prevention and Intervention. Pharmacol Res (2018) 128:366–75. doi: 10.1016/j.phrs.2017.09.014
256. Pereira P, De Oliveira PA, Ardenghi P, Rotta L, Henriques JAP, Picada JN. Neuropharmacological Analysis of Caffeic Acid in Rats. Basic Clin Pharmacol Toxicol (2006) 99(5):374–8. doi: 10.1111/j.1742-7843.2006.pto_533.x
257. Vauzour D, Corona G, Spencer JP. Caffeic Acid, Tyrosol and P-Coumaric Acid are Potent Inhibitors of 5-S-Cysteinyl-Dopamine Induced Neurotoxicity. Arch Biochem Biophys (2010) 501(1):106–11. doi: 10.1016/j.abb.2010.03.016
258. Vauzour D, Vafeiadou K, Spencer JP. Inhibition of the Formation of the Neurotoxin 5-S-Cysteinyl-Dopamine by Polyphenols. Biochem Biophys Res Commun (2007) 362(2):340–6. doi: 10.1016/j.bbrc.2007.07.153
259. Rebai O, Belkhir M, Sanchez-Gomez MV, Matute C, Fattouch S, Amri M. Differential Molecular Targets for Neuroprotective Effect of Chlorogenic Acid and its Related Compounds Against Glutamate Induced Excitotoxicity and Oxidative Stress in Rat Cortical Neurons. Neurochem Res (2017) 42(12):3559–72. doi: 10.1007/s11064-017-2403-9
Keywords: caffeic acid, clinical trials, diabetic neuropathy, inflammatory diseases, inhibitors, targeted therapy
Citation: Alam M, Ahmed S, Elasbali AM, Adnan M, Alam S, Hassan MI and Pasupuleti VR (2022) Therapeutic Implications of Caffeic Acid in Cancer and Neurological Diseases. Front. Oncol. 12:860508. doi: 10.3389/fonc.2022.860508
Received: 23 January 2022; Accepted: 04 February 2022;
Published: 10 March 2022.
Edited by:
Mohd Wasim Nasser, University of Nebraska Medical Center, United StatesReviewed by:
Rajesh Sinha, University of Alabama at Birmingham, United StatesAshutosh Kumar, Tripura University, India
Somasish Dastidar, Manipal Academy of Higher Education, India
Copyright © 2022 Alam, Ahmed, Elasbali, Adnan, Alam, Hassan and Pasupuleti. This is an open-access article distributed under the terms of the Creative Commons Attribution License (CC BY). The use, distribution or reproduction in other forums is permitted, provided the original author(s) and the copyright owner(s) are credited and that the original publication in this journal is cited, in accordance with accepted academic practice. No use, distribution or reproduction is permitted which does not comply with these terms.
*Correspondence: Abdelbaset Mohamed Elasbali , aeelasbali@ju.edu.sa; Visweswara Rao Pasupuleti , pvrao@ums.edu.my