- 1Collaborative Innovation Platform for Modernization and Industrialization of Regional Characteristic Traditional Chinese Medicine, School of Integrated Traditional Chinese and Western Medicine, Binzhou Medical University, Yantai, Shandong, China
- 2Clinical Trial Agency, Yantai Yuhuangding Hospital Affiliated to Qingdao University, Yantai, Shandong, China
Cancer is a major threat to human health, with high mortality and a low cure rate, continuously challenging public health worldwide. Extensive clinical application of traditional Chinese medicine (TCM) for patients with poor outcomes of radiotherapy and chemotherapy provides a new direction in anticancer therapy. Anticancer mechanisms of the active ingredients in TCM have also been extensively studied in the medical field. As a type of TCM against cancer, Rhizoma Paridis (Chinese name: Chonglou) has important antitumor effects in clinical application. The main active ingredients of Rhizoma Paridis (e.g., total saponins, polyphyllin I, polyphyllin II, polyphyllin VI, and polyphyllin VII) have shown strong antitumor activities in various cancers, such as breast cancer, lung cancer, colorectal cancer, hepatocellular carcinoma (HCC), and gastric cancer. Rhizoma Paridis also has low concentrations of certain other active ingredients with antitumor effects, such as saponins polyphyllin E, polyphyllin H, Paris polyphylla-22, gracillin, and formosanin-C. Many researchers have studied the anticancer mechanism of Rhizoma Paridis and its active ingredients. This review article describes research progress regarding the molecular mechanism and antitumor effects of the active ingredients in Rhizoma Paridis, suggesting that various active ingredients in Rhizoma Paridis may be potentially therapeutic against cancer.
Research background
With the growth of aging populations globally, the incidence of cancer is also increasing, which seriously affects the quality of life and life expectancy of cancer patients and has become one of the main causes of death in countries worldwide (Torre et al., 2016). Carcinogenesis is a complex process involving multiple causes including cellular damage, inflammation, proliferation, and genomic instability, leading to alterations in several oncogenic pathways that induce cancer (Liao et al., 2019). To date, many procedures are available to treat cancer that some called “local treatments” such as surgery and radiation therapy which are used for specific tumors or areas. And some are “systemic treatments” that affect the entire body, including chemotherapy, immunotherapy, or targeted therapy. In some case, complementary and alternative therapies are also applied in cancer treatment (Chang et al., 2017). However, these approaches are not very effective because of metastasis and recurrence even after surgery and many side effects in patients after radiotherapy and chemotherapy (Long et al., 2015). Hence, it is crucial to develop new and effective anticancer drugs to inhibit tumor growth and improve the quality of life and survival of cancer patients. More and more traditional Chinese medicines (TCMs), such as Rhizoma Paridis, toad venom, Prunella vulgaris, and Solanum nigrum, have been used in anticancer therapy in TCM, which has gradually become an indispensable tool against cancer (Xiang et al., 2019; Zhang et al., 2020; Li et al., 2021; He et al., 2021).
Rhizoma Paridis is the dried root and rhizome of Paris polyphlla, a perennial herbaceous plant of the Liliaceae (Chinese Pharmacopeia), or Melanthiaceae (The World Flora Online) mainly distributed throughout southwest China. Rhizoma Paridis is listed in the Pharmacopoeia of the People’s Republic of China as the main TCM for heat-clearing and detoxifying (Commission, 2015). According to TCM theory, Rhizoma Paridis has the above functions as well as those of alleviating inflammation (swelling) and relieving pain; it has been extensively used to treat pharyngitis, venomous snake bites, pain, and convulsions (Ding et al., 2021). Rhizoma Paridis is also the main ingredient in many renowned traditional Chinese patent medicines (TCPMs), such as Yunnan Baiyao and Gongxuening capsules (Cunningham et al., 2018). In addition, Rhizoma Paridis has strong antitumor effects and has been widely used in TCM prescriptions for cancer treatments in recent years.
With the development of chemical extraction technology, the active ingredients of Rhizoma Paridis have gradually been identified. The chemical components of Rhizoma Paridis mainly include steroidal saponins, C21 steroids, flavonoids, polysaccharides, and amino acids (Su et al., 2022). As the main active ingredients, steroidal saponins have been shown to have good pharmacological activity (Qin et al., 2016). Specifically, polyphyllin I, polyphyllin II, polyphyllin VI, and polyphyllin VII have been extensively reported as the main active ingredients against cancer (Wu et al., 2012). In addition, the anti-tumor effects of some low-abundance active ingredients in Rhizoma Paridis have been reported in previous studies, such as Paris polyphylla-22 (PP-22, promotes autophagy and apoptosis in nasopharyngeal carcinoma cells) (Tan et al., 2019), polyphyllin E (inhibits proliferation, migration and invasion in ovarian cancer cells) (Liu. et al., 2022), Paris saponin H (induces cell apoptosis, suppresses EMT and invasion in liver cancer cells) (Chen et al., 2019), formosanin C (inhibits pulmonary metastasis on mouse lung adenocarcinoma) (Man et al., 2011b), and gracillin (induces apoptosis and inhibits migration in BGC823 cells) (Liu et al., 2021). In additional, previous studies have demonstrated anticancer activities of polyphyllin in Rhizoma Paridis in a variety of cancers, including lung cancer (Shuli et al., 2011), gastric cancer (Sun et al., 2007), colon cancer (Teng et al., 2015), prostate cancer (Zhang et al., 2018), and melanoma (Man et al., 2011a). Although increasingly more scientists and experts in the medical field have researched the active ingredients in Rhizoma Paridis, due to its complex combination of active ingredients and anticancer mechanisms (Zhang et al., 2010), further research is needed to reveal the mechanism of some active ingredients in Rhizoma Paridis.
In this article, we reviewed the research results regarding active ingredients in Rhizoma Paridis in regulating tumor cells, and we explore and discuss the mechanisms of the main active ingredients in Rhizoma Paridis in regulating different cancers, different signaling pathways, and different molecular targets.
Methods and strategies
We searched the published literature the databases Web of Science, PubMed, China National Knowledge Infrastructure, and Wanfang Data up to July 2022 for original research articles related to the antitumor effects of active ingredients in Rhizoma Paridis against cancer and Rhizoma Paridis combination therapy. Key words: cancer, Chinese medicine, Rhizoma Paridis, Rhizoma Paridis saponins, polyphyllin I, polyphyllin II, polyphyllin VI, polyphyllin VII.
Application of Rhizoma Paridis and research on its active ingredients
Since ancient China, Rhizoma Paridis has generally used in the dosage of 9 g to treat inflammation, infection, sore throat, and bleeding. Pharmacological studies have shown that Rhizoma Paridis has significant antitumor, hemostatic, antiinfection, and antioxidant activities (Zhao et al., 2009; Liu et al., 2012b). Rhizoma Paridis is the main ingredient in well-known TCPMs including Yunnan Baiyao, Gongxuening capsule, and Baibaodan that are mainly used for promoting blood circulation, removing blood stasis, heat-clearing, detoxifying, and regulating menstruation, according to TCM theory (Liu et al., 2009; Yao et al., 2021). Moreover, as a traditional anticancer TCM, the active ingredients of Rhizoma Paridis have been widely studied as potential new anticancer drugs. Studies have shown that the root extract of Rhizoma Paridis has obvious inhibitory effects on lung cancer, gastric cancer, colon cancer, and breast cancer (You et al., 2021). Other studies have also shown that the fruit and aerial parts of Rhizoma Paridis have the effects of inhibiting the migration of cancer cells (Qin et al., 2018; Lin et al., 2021).
Research on Rhizoma Paridis extraction and identification has confirmed that the active ingredients in Rhizoma Paridis include steroidal saponins, cholesterol, C21 steroids, phytosterols, insect allergens, triterpenoids, flavonoids, and other chemical compounds (Wei et al., 2014). Steroidal saponins, also known as Rhizoma Paridis saponins (RPS), are the main biologically active chemical components of Rhizoma Paridis and are divided into four types (Kang et al., 2012): spirostanol; isosprirostanol; furostanol; and pseudospirostand and mainly include polyphyllin I, polyphyllin II, polyphyllin VI, formosanin C, polyphyllin VII, gracillin, and other saponin active ingredients (Figure 1). Numerous studies have shown that saponins have antitumor activities in a variety of tumor cells. Among them, five steroidal saponins including total saponins, polyphyllin I, polyphyllin II, polyphyllin VI, and polyphyllin VII have aroused the most attention and have been studied the most. The antitumor activities of these five steroidal saponins have also been verified in a variety of cancers.
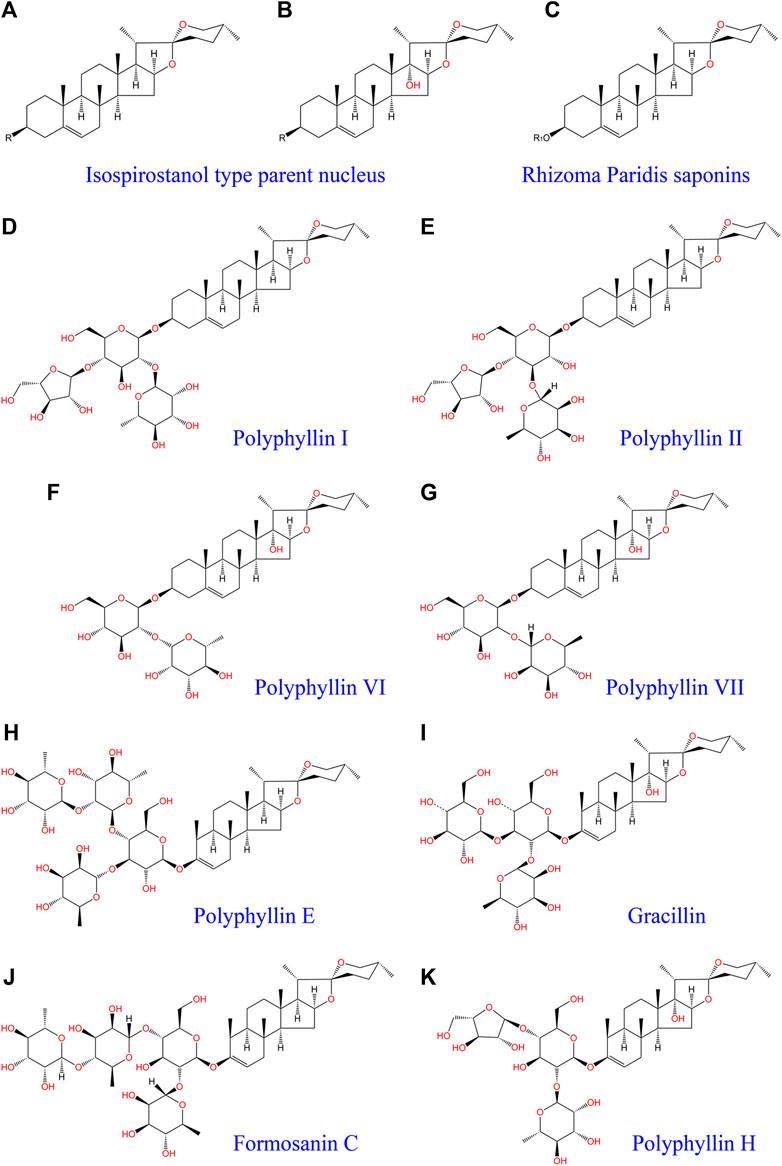
FIGURE 1. Isospirostanol type parent nucleus (A,B), Rhizoma Paridis saponins (C), polyphyllin I (D), polyphyllin II (E), polyphyllin VI (F), polyphyllin VII (G), polyphyllin E (H), Gracillin (I), Formosanin C (J), and polyphyllin H (K). “R1” indicates that different functional groups represent different types of saponins with different molecular structures.
Antitumor effects of RPS
RPS is a general term for steroidal saponins in Rhizoma Paridis, with strong activity against hepatocellular carcinoma (HCC), lung cancer, colon cancer, and glioma (Man et al., 2009; He et al., 2014; Qiu et al., 2016; Yao et al., 2018; Lin et al., 2019). RPS has been widely reported as a potential anticancer drug with the main mechanism of inhibiting cell proliferation, inducing apoptosis, autophagy, and cell cycle arrest, as well as enhancing the sensitivity of chemotherapeutic drugs (figure 2).
Anti-HCC activities of RPS
Protein analysis using matrix-assisted laser desorption/ionization time-of-flight mass spectrometry in RPS-treated hepatoma HepG2 cells revealed that the levels of deoxyuridine triphosphatase, heterogeneous nuclear ribonucleoprotein K, and guanosine monophosphate synthetase were significantly high whereas levels deoxyribonuclease gamma, nucleoside diphosphate kinase A, and centrin-2 were significantly low in the cells. These protein molecules have been confirmed to be closely related to tumorigenesis and tumor progression (Cheng et al., 2008). A recent study showed that RPS treatment in H22 mice bearing HCC not only inhibited but also slowed tumor growth in the mice. RPS also inhibited the metabolism of glycine and serine, reversed aerobic glycolysis, and suppressed adipogenesis, thereby inhibiting the metabolism of tumor cells and effectively suppressing the growth of hepatoma in tumor-bearing mice (Qiu et al., 2016). Another experimental study in H22 tumor-bearing mice also confirmed that RPS significantly reduced levels of glucose, glycine, and alanine and inhibited the fatty acid oxidation pathway and gluconeogenesis pathway that are involved in the body’s energy supply, thereby exerting an anticancer effect (Man et al., 2014b). RPS may also overcome sorafenib resistance in mice hepatocellular carcinoma H22-bearing through the mitochondrial damage pathway and inhibition of phosphatidylinositol-3-kinase (PI3K)/Akt/mammalian target of rapamycin (mTOR) pathway-based lipid synthesis (Yao et al., 2018). In short, RPS is a potential future therapeutic agent against HCC.
Anti-lung cancer activities of RPS
For a long time, RPS has been studied for its therapeutic effect on lung cancer. Overexpression of matrix metalloproteinases (MMPs) occurs in a variety of malignant tumors and promotes tumor development by promoting cancer cell growth and migration as well as tumor invasion, metastasis, and angiogenesis (Hidalgo and Eckhardt, 2001). RPS inhibits the expression and secretion of MMP2 and MMP9 in A549 lung cancer cells to further inhibit the proliferation, migration, and differentiation of A549 cells (He et al., 2014). Another study has shown that RPS significantly downregulates the expression of P53, B-cell lymphoma 2 (BCL2), and cyclin-dependent kinase 2 (CDK2) and significantly upregulates the expression of P21 and BCL2-associated X (Bax) in A549 cells, thereby inducing cell cycle arrest at the G1 phase. P53 is an important tumor suppressor regulating cell growth and apoptosis in the process of carcinogenesis. RPS directly or indirectly affects the expression of target-regulated p53, thereby changing the expression of downregulated genes including P21, Bax, BCL2, and CDK2 and resulting in inhibition of cell proliferation, cell cycle arrest, and apoptosis (Zhang et al., 2015). In addition, RPS reduces the level of inflammatory cytokines, tumor necrosis factor-alpha (TNF-α), interleukin (IL)-8 and IL-10 in the serum of C57BL/6 mice through immunomodulation and induces nuclear changes in A549 cells, such as DNA condensation and chromatin fragmentation, thereby inducing apoptosis in the cells. The inhibitory effect of RPS in lung cancer may be achieved by reducing the inflammatory response and inducing tumor cell apoptosis (Li et al., 2013). RPS also has an inhibitory effect in diethyl nitrosamine (DEN)-induced lung adenoma to significantly reduce energy metabolism and glycine, serine, and threonine metabolism, thereby blocking tumor growth. In addition, RPS significantly reduces the expression of inflammatory cytokines, such as TNF-α, IL-6, cyclooxygenase-2 (COX-2), and prostaglandin E2 in lung cancer tissues, reduces the infiltration of inflammatory cells and liver toxicity caused by DEN (Man et al., 2015; Man et al., 2017). In brief, RPS has potential as a future therapeutic agent that can effectively suppress lung tumors.
Anti-intestinal cancer activities of RPS
Angiogenesis, the development of new blood vessels from pre-existing blood vessels, is an important factor in tumorigenesis and tumor progression (Potente et al., 2011). Tumor progression relies on angiogenesis for nutrient supply and metastasis. RPS has been shown to have selective cytotoxic effects on colon cancer cells (i.e., Lovo cells) and to exhibit significant antiangiogenic effects. RPS inhibits the proliferation, differentiation, and migration of Lovo cells by suppressing angiogenesis; it also induces apoptosis and cell cycle arrest in Lovo cells (Qian et al., 2012). Another study showed that RPS induces death of the colorectal adenocarcinoma cell line DLD-1 by upregulating autophagy markers without triggering apoptosis dependent on p53 and caspase-3, i.e., RPS inhibits cellular DNA production by inducing autophagy. Combined application of RPS doxorubicin to treat colorectal cancer cells showed a stronger anticancer effect than RPS monotherapy (Lin et al., 2019).
Anti-glioma activities of RPS
Studies have shown that RPS also has a good anticancer effect on glioblastoma, one of the most aggressive type of cancers and among the hardest to treat. Its frequent recurrence limits the therapeutic effects of drugs against glioblastoma, mainly related to the drug resistance of glioblastoma cells (Mrugala, 2013). Drug resistance in glioblastoma treatment is also exhibited with temozolomide (TMZ), a clinical chemotherapeutic drug with broad-spectrum antitumor activity, and DNA-repair enzyme O6-methylguanine-DNA methyltransferase (MGMT) plays a key role in TMZ resistance (Lai et al., 2018). A previous study showed that RPS regulates the expression of MGMT by downregulating the expression of PI3K/AKT and its downstream protein nuclear factor κappa-light-chain-enhancer of activated B cells (NF-κB) p65, thereby inhibiting TMZ resistance and inducing mitochondrial apoptosis of U87R cells; results of Cell Counting Kit-8 colorimetric assay and flow cytometry indicated that RPS significantly inhibited the proliferation of glioblastoma cells and TMZ-resistant glioblastoma U87R cells (Zhang et al., 2020).
Other antitumor activities of RPS
Studies have shown that COX-2 dysfunction is related to digestive system cancer, and its expression level is correlated with the aggressiveness of tumor progression (Misra and Sharma, 2014). A previous study confirmed that RPS significantly reduced the expression of COX-2 and cyclin D1 in rat esophageal tissue and esophageal cancer cells, thereby inducing apoptosis of esophageal cancer cells and cell cycle G2/M arrest. RPS also significantly reduced the release of prostaglandin E2, a downstream molecule of COX-2 in a dose-dependent manner. These results suggest that RPS inhibits the development of esophageal cancer by promoting apoptosis and cell cycle arrest and inhibiting the COX-2 pathway (Yan et al., 2015). RPS exerts antitumor effects in osteosarcoma by inhibiting tumor cell proliferation, metastasis, and vasculogenic mimicry (Yao et al., 2022). In addition, in an experimental study involving a mouse model of cancer pain, it was found that RPS can increase 5-HT and β-EP in the brain by inhibiting inflammatory pain caused by oxidative damage, suggesting that RPS has a good therapeutic effect on cancer pain and provides a new tool in the treatment of cancer (Wang G. et al., 2018).
Antitumor effects of polyphyllin I
Polyphyllin I is one of the main active ingredients of steroidal saponins in Rhizoma Paridis and is also known as polyphyllin -D (Li et al., 2001; Lee et al., 2005; Tian et al., 2020). It has relatively strong antitumor effects on a variety of tumors, such as HCC (Cheung et al., 2005), lung cancer (Siu et al., 2008), prostate cancer (Xiang et al., 2018), breast cancer (Li et al., 2017), and malignant glioma (Liu et al., 2017). Polyphyllin I exerts anticancer effects including induction of apoptosis, autophagy and cycle arrest, and inhibition of cell migration and invasion (Figure 3).
Anti-HCC activities of polyphyllin I
One study showed that after treating HepG2 cells with polyphyllin I, protein levels of p21 and cyclin E were significantly increased whereas the expression of CDK2 and cyclin A2 was significantly decreased, thereby arresting the cell cycle in the G2/M phase and inhibiting tumor cells. In addition, polyphyllin I induced the generation of reactive oxygen species (ROS) and depolarization of MMPs in HepG2 cells, thereby increasing the release of mitochondrial cytochrome c, the Bax/BCL2 ratio, and the activation of caspase-3, caspase-8, and caspase-9, leading to apoptosis of the tumor cells (Zeng et al., 2020). Another study suggested that polyphyllin I inhibited both the formation of angiogenic mimicry by blocking the PI3K-Akt-Twist1-VE-cadherin pathway and transcriptional activation of the Twist1 promoter and interfered with the binding of Twist1 to the VE-cadherin promoter to block the formation of vasculogenic mimicry, thereby inhibiting the metastasis of various cancer cells, including HCC (Xiao et al., 2018). In addition, an animal study confirmed the therapeutic effect of polyphyllin I against HCC and showed that polyphyllin I inhibited HCC by activating caspase-dependent and caspase-independent apoptosis pathways and inhibiting the PI3K/Akt signaling pathway, thereby suppressing tumor growth in a xenograft mouse model injected with HepG2 cells (Chen et al., 2014). The results of the above studies suggest that polyphyllin I has strong potential as an HCC treatment.
Anti-lung cancer activities of polyphyllin I
Lung cancer, especially non-small cell lung cancer (NSCLC), is considered to be the leading cause of death worldwide and the most prevalent cancer (Siegel et al., 2020). The therapeutic effect of polyphyllin I on lung cancer has been verified in numerous studies. HOX transcript antisense RNA (HOTAIR) is considered to be a potential biomarker for patients with NSCLC that is associated with tumor metastasis and poor prognosis and is highly expressed in NSCLC (Yang et al., 2018). Polyphyllin I reduces the expression of HOTAIR, increases the protein level of the transcription factor c-Jun, and also induces the protein expression and promoter activity of cyclin-dependent kinase inhibitor p21, thereby inhibiting human lung cancer cell growth, migration, and differentiation (Zhao et al., 2019). Another study showed that polyphyllin I increased phosphorylation of stress-induced stimulation of stress-activated protein kinase (SAPK)/c-Jun N-terminal kinase (JNK) and decreased the expression of P65 and DNMT1 protein. Reduction of P65 and DNMT1 protein expression resulted in decreased expression of enhancer of Zeste 2 polycomb repressive complex 2 subunit gene (EZH2). As genes that are highly expressed in various cancers, regulating the expression of DNMT1 and EZH2 may become novel targets in anticancer therapy (Lin and Wang, 2014). The results of the above studies have clarified that polyphyllin I inhibits the proliferation and differentiation of lung cancer cells by activating SAPK/JNK, reducing the expression of p65 and DNMT1, and inhibiting the expression of EZH2 (Yu et al., 2016).
Numerous studies have shown that adenosine monophosphate-activated protein kinase (AMPK) is a key energy-sensitive kinase that is widely involved in autophagy (Shi et al., 2012). Polyphyllin I directly binds to allosteric drugs and metabolite sites of AMPK, induces autophagy through AMPK/mTOR signaling, and inhibits the growth of NSCLC cells (Wu et al., 2020). Moreover, after polyphyllin I treatment, the expression of BCL2 in NSCLC cells is reduced, and the expression of Bax and caspase 3 is increased, thereby inducing apoptosis of lung cancer cells (Jiang et al., 2014). Overcoming drug resistance in lung cancer cells is an important research topic. Polyphyllin I reverses resistance of NSCLC cells to osimertinib by regulating the PI3K/Akt signaling pathway (Lai et al., 2021) and overcomes epithelial mesenchymal transition (EMT)-associated resistance to erlotinib in lung cancer cells by inhibiting the IL-6/STAT3 pathway (Lou et al., 2017). Polyphyllin I also induces apoptosis of gefitinib-resistant NSCLC cells by regulating the MALAT1/STAT3 signaling pathway (Yang Q. et al., 2018) and enhances chemosensitivity of cisplatin-resistant NSCLC cells by inhibiting the cellular inhibitor of the protein phosphatase 2 A (CIP2A)/Akt/mTOR signaling axis (Feng F. et al., 2019; Feng F. F. et al., 2019). Interestingly, polyphyllin I combined with hyperthermia arrests the cell cycle in the G2/M phase and promotes apoptosis by regulating the expression of BCL2, Bax, and Caspase-3, ultimately inhibiting the proliferation of NSCLC cells (Zhao et al., 2015). In short, polyphyllin I is expected to be useful in anti-lung cancer therapy.
Other antitumor activities of polyphyllin I
Many studies have shown the anti-gastric cancer effects of polyphyllin I. For example, application of inhibitors targeting the Janus kinase 2/signal transducer and activator of transcription 3 (JAK/STAT3) pathway (e.g., AG490) may have a strong effect in anti-gastric cancer therapy (Bogani et al., 2013). Studies have confirmed that polyphyllin I mainly inhibits the phosphorylation of STAT3 in a way that inhibits the expression of apoptosis-related protein, such as BCL2, and induces apoptosis of gastric cancer cells (Han et al., 2020). Another experimental study confirmed that polyphyllin I promotes the transformation of mesenchymal cells to epithelial cells, thereby partially inhibiting the migration and invasion of gastric cancer cells. In addition, polyphyllin I downregulates expression of the cellular inhibitor of the CIP2A gene and induces degradation of CIP2A protein, downregulation of Akt phosphorylation, and apoptosis of gastric cancer cells. Knocking out of CIP2A shows the same effect as polyphyllin I, which also indirectly supports the above findings (Zhang Y. et al., 2018). According to another experimental study, polyphyllin I acts as an inhibitor of pyruvate dehydrogenase kinase 1/Akt/mTOR signaling by promoting the conversion of microtubule-associated protein 1A/1B-light chain 3 (LC3)-I to LC3-II and downregulating cyclin B1 to induce autophagy and cell cycle arrest in gastric cancer HGC-27 cells (He et al., 2019). This also provides an important basis for the anti-gastric cancer effect of polyphyllin I. Moreover, studies have shown that polyphyllin I inhibits prostate cancer invasion and induces apoptosis of prostate cancer cells through the CIP2A/protein phosphatase 2A (polyphyllin 2A)/extracellular signal-regulated kinase (ERK) signaling pathway (Liu X. et al., 2018); it also has a certain inhibitory effect on ovarian cancer cells in mice (Gu et al., 2016).
Antitumor effects of polyphyllin II
Polyphyllin II is one of the main active ingredients of steroidal saponins in Rhizoma Paridis and has strong antitumor activities. The main mechanisms by which polyphyllin II exerts antitumor effects include induction of apoptosis, autophagy, and cell cycle arrest and inhibition of metastasis (Figure 4). However, few studies report the effects of polyphyllin II and are mainly all on ovarian cancer. Angiogenesis refers to the growth of new blood vessels from pre-existing vascular endothelial cells. Pathological angiogenesis is best known for its role in gynecological tumor growth and metastasis (Gómez-Raposo et al., 2009). Polyphyllin II inhibits vascular endothelial growth factor (VEGF)-induced phosphorylation of various intracellular proangiogenic kinases, such as extracellular signal-related kinases, AKT kinases, focal adhesion kinases, and Src family kinases, by blocking the activation of VEGF receptor 2 in endothelial cells, thereby inhibiting angiogenesis in the ovarian cancer mouse model and achieving an inhibitory effect on the growth of ovarian cancers. These results suggest that polyphyllin II treatment inhibits almost all essential elements of angiogenesis (i.e., VEGF-induced endothelial cell proliferation, migration, and angiogenesis), with good potential therapeutic importance (Xiao et al., 2014). There is also evidence that polyphyllin II inhibits ovarian cancer cell angiogenesis by regulating NF-κB signaling, thereby inhibiting ovarian cancer cell growth (Yang et al., 2015). Polyphyllin II also increases the expression of pro-apoptotic elements, Bax, cytoplasmic cytochrome c, activated caspase-3, and activated caspase-9 in polyphyllin II-treated SKOV3 cells and reduces the phosphorylation of ERK1/2 and the expression of antiapoptotic BCL2, resulting in cell cycle arrest and apoptosis in ovarian cancer cells (Xiao et al., 2012).
Polyphyllin II also has a good therapeutic effect on HCC by inducing cell cycle arrest and apoptosis through the mitochondrial pathway (Long et al., 2015; Wang et al., 2019b). Experimental data have shown that polyphyllin II also inhibits invasion and metastasis in human bladder cancer by regulating the expression of EMT-related factors and MMPs. Snail family transcriptional repressor 2 (SNAI2) and Twist1 are major transcription factors regulating EMT that may promote tumor metastasis by enhancing cell invasion (Kudo-Saito et al., 2009). A study showed that the expression of SNAI2, Twist1, MMP-2, and MMP-9 in bladder cancer cells was significantly decreased, and cell scratch experiments showed significant inhibition of the migration and invasion of bladder cancer cells after polyphyllin II treatment (Niu et al., 2020). Polyphyllin II also induces autophagy through the Akt/mTOR signaling pathway, thereby promoting apoptosis of breast cancer cells (Xie et al., 2017). In addition, polyphyllin II treatment enhances the sensitivity of lung cancer cells to cisplatin, which provides important data support for polyphyllin II as a chemosensitizer (Man et al., 2020).
Antitumor effects of polyphyllin VI
Polyphyllin VI is a saponin active ingredient extracted from the root of Rhizoma Paridis and its main anticancer mechanisms include induction of apoptosis, autophagy, and cell cycle arrest (Figure 5). A previous study suggested that polyphyllin VI inhibited glioma growth by increasing the accumulation of ROS in glioma cells and activating the ROS-regulated JNK and P38 pathway, thereby inducing apoptosis and autophagy in cells; applications of the ROS inhibitor N-acetylcysteine significantly attenuated polyphyllin VI-mediated apoptosis and autophagy. These results confirmed that the antitumor activity of polyphyllin VI in glioma cells was via the accumulation of ROS and activation of the JNK and P38 pathway (Liu et al., 2020). Polyphyllin VI also effectively inhibited the proliferation of osteosarcoma cells by regulating ROS/JNK activities, blocking the human osteosarcoma cell cycle in the G2/M phase, and inducing apoptosis and autophagy (Yuan et al., 2019). In addition, polyphyllin VI exhibited a strong anti-metastatic effect in a breast cancer 4T1 mouse model by significantly inhibiting cancer cell migration and invasion (Wang P. et al., 2019). Polyphyllin VI also significantly inhibited the proliferation of A549 and NCI-H1299 lung cancer cells by inducing cell cycle arrest in the G2/M phase and apoptosis (Lin et al., 2015).
Unlike other compounds, polyphyllin VI has significant hepatotoxicity. Evaluation of drug hepatotoxicity in HepaRG liver stem cells showed that polyphyllin VI promoted ROS production to induce the release of cytochrome c from mitochondria to the cytoplasm and to activate Fas, caspase-3, caspase-8, caspase-9, and poly (ADP-ribose) polymerase proteins, thereby leading to morphological changes in HepaRG cells and inducing apoptosis. These results suggest that safety evaluation of polyphyllin VI is necessary (Liu Y. et al., 2018).
Antitumor effects of polyphyllin VII
Polyphyllin VII is one of the main monomer components of polyphyllin steroid saponins and its anticancer mechanisms mainly include the induction of apoptosis and autophagy (Figure 6). A previous study showed that polyphyllin VII significantly increased the phosphorylation of AMPK and BCL2 and inhibited the phosphorylation of PI3K, AKT, and mTOR in HepG2 cells, thereby inducing autophagy and apoptosis; SP600125 JNK inhibitor pretreatment reversed polyphyllin VII-induced autophagy and apoptosis. These results suggest that polyphyllin VII may induce autophagic cell death in HepG2 cells by inhibiting the PI3K/AKT/mTOR and activating the JNK pathways (Zhang et al., 2016b). Moreover, polyphyllin VII induced apoptosis and autophagy in human osteosarcoma U2OS cells by regulating the JNK pathway (Li X. et al., 2021). Another study showed that polyphyllin VII promoted ROS production in HepG2 cells, leading to depolarization of mitochondrial membrane potential, upregulation of the Bax/BCL2 ratio and protein levels in cleaved forms of caspase-3, caspase-8, and caspase-9, and poly (ADP ribose) polymerase, which eventually led to apoptosis. Polyphyllin VII also significantly enhanced the expression of P53 and phosphatase and tensin homolog (PTEN) and the phosphorylation levels of JNK, ERK, and P38, suggesting that the MAPK and PTEN/P53 signaling pathways are also involved in polyphyllin VII-induced apoptosis of HepG2 cells (Zhang et al., 2016a). Polyphyllin VII also inhibited the growth of human cervical cancer HeLa cells by inducing apoptosis (Zhang et al., 2014).
Notably, polyphyllin VII induces mitochondrial dysfunction not only by promoting reactive oxygen species (ROS) production, but also increasing mitochondrial fission. Dynein-related protein 1 (Drp1) plays an important role in regulating mitochondrial function (Karbowski et al., 2002). Polyphyllin VII enhances the mitochondrial localization of Drp1 by increasing polyphyllin 2A activity and decreasing AKT activity; LB100, a specific polyphyllin 2A inhibitor, attenuates polyphyllin VII-induced mitochondrial fission and apoptosis in SKOV3 ovarian cancer cells. These findings further confirm the role of the polyphyllin 2A/Akt pathway in the regulation of mitochondrial function by polyphyllin VII (Zhao et al., 2021). In addition, polyphyllin VII induces apoptosis in human lung cancer A549 cells by inhibiting the PI3K/Akt and NF-κB pathways, leading to mitochondrial dysfunction (He et al., 2020). Polyphyllin VII also enhances the sensitivity of glioma cells to temozolomide by inhibiting the decreased expression of MGMT (Pang et al., 2019).
Antitumor effects of other active ingredients in Rhizoma Paridis
In addition to the main active ingredients in Rhizoma Paridis, including RPS, polyphyllin I, and polyphyllin II, some less-abundant active ingredients, such as saponins polyphyllin E, polyphyllin H, Paris polyphylla-22, gracillin, and formosanin, also have antitumor effects. According to a previous study, polyphyllin E inhibits ovarian cancer cells by downregulating the AKT/NF-κB pathway, reducing the expression of MMP2 and MMP9, and inhibiting the proliferation, migration, and invasion of ovarian cancer cells, thereby achieving an inhibitory effect in ovarian cancer cells (Liu Y. et al., 2022). In another study, polyphyllin H inhibited the growth of hepatoma cells and xenografts by suppressing the Wnt/β-catenin pathway, while knocking out of the β-catenin gene significantly inhibited this phenomenon (Chen T. et al., 2019). In addition, polyphyllin H-treated U251 human glioma cells had upregulated P21 and P27 expression and downregulated cyclin D expression, which further induced cell cycle arrest of U251 cells at the G1 phase. Further experimental data showed that polyphyllin H reduced U251 cell survival by inhibiting the expression of ARA1 and ARA3, subsequently suppressing the phosphorylation of Akt and MAPK, inducing apoptosis and cell cycle arrest, and inhibiting the proliferation of glioma cells (Bi et al., 2021). Another active ingredient, polyphyllin-22, is reported to promote autophagy and apoptosis in CNE-2 nasopharyngeal carcinoma cells by inducing endoplasmic reticulum stress, downregulating the STAT3 signaling pathway, and regulating the MAPK pathway (Tan et al., 2019). In addition, polyphyllin-22 induced cell cycle arrest in S-phase and G2/M-phase and apoptosis in SCC-15 human tongue squamous cell carcinoma cells by activating the p38 and caspase-8/caspase-3 pathways (Ke et al., 2016). Some researchers showed that formosanin C inhibited metastasis of lung adenocarcinoma in mice by inhibiting MMPs through wound healing and migration assays. The anticancer effect of formosanin C is significantly better than that of cisplatin whereas the side effects of formosanin C were fewer (Man et al.). Formosanin C also has an inhibitory effect on HCC cells (Qin et al., 2012; Li et al., 2014). Lastly, a study showed that as a TIPE2 inducer, gracillin inhibits the proliferation and migration of BGC823 gastric cancer cells (Liu et al., 2021).
The other effects of active ingredients in Rhizoma Paridis
Previous studies investigated that besides anti-tumor effects, some active ingredients of Rhizoma Paridis have various biological activities such as anti-inflammation, anti-fibrosis, hemostasis, and antibiosis. For instance, polyphyllin I, polyphyllin D, and polyphyllin G were verified have potential anti-inflammatory effects in various inflammatory animal models (Wang Q. et al., 2018; Zhang et al., 2019; Zhu et al., 2019). In additional, CCl4-induced hepatic fibrosis were significantly improved by polyphyllin D, polyphyllin G, polyphyllin VI, and formosanin C (Man et al., 2014a; Hong et al., 2016; Han et al., 2019). The mouse tail snipping model demonstrated that Paris saponin H, polyphyllin I, polyphyllin II, and polyphyllin VII could serve as favorable hemostatic agents (Sun et al., 2014; Wen et al., 2019; Qiao et al., 2021). Polyphyllin V and polyphyllin VII showed powerful antibacterial activity against propionibacterium acnes (Qin et al., 2012).
Outlook for future research
The number of cancer patients increases every year as chemotherapy and radiotherapy fail to address the root cause of cancers. Prolonged administration and increased doses of chemotherapeutic drugs also increase the drug resistance of tumor cells and the possibility of serious side effects in the human body, resulting in treatment failure (Cao et al., 2017). For example, patients are often intolerant to cisplatin-based therapeutic regimens, resulting in poor therapeutic outcomes and the associated adverse reactions, such as hair loss, nausea, and vomiting (Dasari and Tchounwou, 2014). Even after surgical treatments, the chance of metastasis and cancer recurrence is very high (Niibe and Hayakawa, 2010). Under these circumstances, TCM, which has been used for thousands of years, is gradually becoming recognized by oncologists and cancer patients as an option. As an anticancer TCM, Rhizoma Paridis has shown good results in clinical applications for many years. This is of great importance for the scientific and rational application of Rhizoma Paridis and the unique antitumor effects of TCM. Nevertheless, no anticancer drugs use Rhizoma Paridis as the main therapeutic agent in clinical practice. Hence, there is an urgent need to clarify the underlying therapeutic mechanism of the active ingredients in Rhizoma Paridis to lay the foundation for future clinical applications.
Studies have shown that some active ingredients of Rhizoma Paridis exhibit cytotoxicity, such as hepatoxicity. In particular, the saponin component polyphyllin I has relatively strong cytotoxicity. Previous research on the order of cytotoxicity among four saponins in HL-7702 cells showed that polyphyllin I and polyphyllin VII had similar cytotoxicity, followed by polyphyllin II and polyphyllin VI. These four saponins induce apoptosis in liver cells by activating ROS stress and death receptor pathways (Wang et al., 2019c). Animal studies have shown that Rhizoma Paridis causes side effects, such as nausea, vomiting, diarrhea (Liu et al., 2012a), and even hemolysis (Liu J. et al., 2022). Other studies have shown that the water extract of turmeric enhanced the antitumor effects of trichosanthesin and significantly reduced gastric irritation of trichosanthesin, thereby reducing its toxicity (Man et al., 2013; Liu et al., 2014; Man et al., 2016). To comprehensively assess the toxicity of polyphyllin II in the intestinal tract, pharmacologists used the Swiss-rolling technique of intestinal tissue preparation for immunohistochemistry to observe histopathological changes in the entire intestinal tract. The results showed that polyphyllin II had no obvious toxicity at a dose of 20 mg/kg in vivo (Chen M. et al., 2019). Intravesical instillation has also been proposed to avoid gastrointestinal toxicity and intravenous incompatibility (Guo et al., 2018). Thus, future research and development of the TCM Rhizoma Paridis should not only focus on enhancing its antitumor activities and clarifying its antitumor mechanism but also on reducing its toxicity and side effects. More clinical studies of the therapeutic safety of Rhizoma Paridis should be carried out to ensure the safety and efficacy of this TCM medicine and its active ingredients in anticancer therapy.
Conclusion
Many chemotherapeutic drugs cause serious adverse reactions in clinical application, resulting in poor patient outcomes (Islam et al., 2019). There is an urgent need to develop new therapeutic drugs against cancers with high efficacy and less toxicity. Recent evidence has shown that the combination of chemotherapy drugs and TCM has better therapeutic efficacy and reduces the side effects of chemotherapy (Zhang and Xiao, 2021). Under these conditions, anticancer components extracted from natural medicines may become the most promising anticancer drugs. As a representative antitumor drug in TCM, Rhizoma Paridis has a wide range of clinical applications. In this review article, we discussed the antitumor effects and molecular mechanisms of the main active ingredients in Rhizoma Paridis. According to previous literature, the inhibitory effects of these active ingredients on tumor cells are achieved through various ways, such as apoptosis, autophagy, cell cycle arrest, inhibiting metastasis, and reversing drug resistance (Table 1). These findings suggest that active ingredients, such as Rhizoma Paridis saponins, may be potential drugs for the clinical treatment of cancer in the future. However, further experimental studies are needed to elucidate the exact molecular mechanisms prior to its clinical application. In addition, the complex composition of compounds extracted from Rhizoma Paridis and the toxicity of Rhizoma Paridis itself also limit its clinical application. Hence, in-depth research is required to apply the active ingredients extracted from Rhizoma Paridis to clinical settings. This article aims to provide theoretical support and medication guidance for the clinical application of Rhizoma Paridis by summarizing the therapeutic efficacies of the active ingredients of Rhizoma Paridis in anticancer therapy.
Author contributions
Conceptualization, JL, QZ, and DL; Data curation, JJ, WZ, and JC; Writing–original draft, JL, JJ, and WZ; Writing–review and editing, QZ and DL.
Funding
This research was funded by the National Natural Science Foundation of China (82073313 to DL, 31870338 to QZ), Taishan Scholars Construction Engineering of Shandong Province (to DL), the Yantai High-End Talent Introduction Plan “Double Hundred” (to DL).
Conflict of interest
The authors declare that the research was conducted in the absence of any commercial or financial relationships that could be construed as a potential conflict of interest.
Publisher’s note
All claims expressed in this article are solely those of the authors and do not necessarily represent those of their affiliated organizations, or those of the publisher, the editors and the reviewers. Any product that may be evaluated in this article, or claim that may be made by its manufacturer, is not guaranteed or endorsed by the publisher.
References
Bi, L., Liu, Y., Yang, Q., Zhou, X., Li, H., Liu, Y., et al. (2021). Paris saponin H inhibits the proliferation of glioma cells through the A1 and A3 adenosine receptor-mediated pathway. Int. J. Mol. Med. 47 (4), 30. doi:10.3892/ijmm.2021.4863
Bogani, C., Bartalucci, N., Martinelli, S., Tozzi, L., Guglielmelli, P., Bosi, A., et al. (2013). mTOR inhibitors alone and in combination with JAK2 inhibitors effectively inhibit cells of myeloproliferative neoplasms. PLoS One 8 (1), e54826. doi:10.1371/journal.pone.0054826
Cao, L., Chen, J., Ou, B., Liu, C., Zou, Y., and Chen, Q. (2017). GAS5 knockdown reduces the chemo-sensitivity of non-small cell lung cancer (NSCLC) cell to cisplatin (DDP) through regulating miR-21/PTEN axis. Biomed. Pharmacother. 93, 570–579. doi:10.1016/j.biopha.2017.06.089
Chang, Y., He, L., Li, Z., Zeng, L., Song, Z., Li, P., et al. (2017). Designing core-shell gold and selenium nanocomposites for cancer radiochemotherapy. ACS Nano 11 (5), 4848–4858. doi:10.1021/acsnano.7b01346
Chen, M., Ye, K., Zhang, B., Xin, Q., Li, P., Kong, A. N., et al. (2019a). Paris Saponin II inhibits colorectal carcinogenesis by regulating mitochondrial fission and NF-κB pathway. Pharmacol. Res. 139, 273–285. doi:10.1016/j.phrs.2018.11.029
Chen, T., Lin, J., Tang, D., Zhang, M., Wen, F., Xue, D., et al. (2019b). Paris saponin H suppresses human hepatocellular carcinoma (HCC) by inactivation of Wnt/β-catenin pathway in vitro and in vivo. Int. J. Clin. Exp. Pathol. 12 (8), 2875–2886.
Chen, Y. S., He, Y., Chen, C., Zeng, Y., Xue, D., Wen, F. Y., et al. (2014). Growth inhibition by pennogenyl saponins from Rhizoma paridis on hepatoma xenografts in nude mice. Steroids 83, 39–44. doi:10.1016/j.steroids.2014.01.014
Cheng, Z. X., Liu, B. R., Qian, X. P., Ding, Y. T., Hu, W. J., Sun, J., et al. (2008). Proteomic analysis of anti-tumor effects by Rhizoma Paridis total saponin treatment in HepG2 cells. J. Ethnopharmacol. 120 (2), 129–137. doi:10.1016/j.jep.2008.07.030
Cheung, J. Y., Ong, R. C., Suen, Y. K., Ooi, V., Wong, H. N., Mak, T. C., et al. (2005). Polyphyllin D is a potent apoptosis inducer in drug-resistant HepG2 cells. Cancer Lett. 217 (2), 203–211. doi:10.1016/j.canlet.2004.06.042
Commission, C. P. (2015). Pharmacopoeia of People’s Republic of China (I). Beijing: China Medical Science and Technology Press.
Cunningham, A. B., Brinckmann, J. A., Bi, Y. F., Pei, S. J., Schippmann, U., and Luo, P. (2018). Paris in the spring: A review of the trade, conservation and opportunities in the shift from wild harvest to cultivation of Paris polyphylla (trilliaceae). J. Ethnopharmacol. 222, 208–216. doi:10.1016/j.jep.2018.04.048
Dasari, S., and Tchounwou, P. B. (2014). Cisplatin in cancer therapy: Molecular mechanisms of action. Eur. J. Pharmacol. 740, 364–378. doi:10.1016/j.ejphar.2014.07.025
Ding, Y. G., Zhao, Y. L., Zhang, J., Zuo, Z. T., Zhang, Q. Z., and Wang, Y. Z. (2021). The traditional uses, phytochemistry, and pharmacological properties of Paris L. (Liliaceae): A review. J. Ethnopharmacol. 278, 114293. doi:10.1016/j.jep.2021.114293
Feng, F., Cheng, P., Wang, C., Wang, Y., and Wang, W. (2019a). Polyphyllin I and VII potentiate the chemosensitivity of A549/DDP cells to cisplatin by enhancing apoptosis, reversing EMT and suppressing the CIP2A/AKT/mTOR signaling axis. Oncol. Lett. 18 (5), 5428–5436. doi:10.3892/ol.2019.10895
Feng, F. F., Cheng, P., Sun, C., Wang, H., and Wang, W. (2019b). Inhibitory effects of polyphyllins I and VII on human cisplatin-resistant NSCLC via p53 upregulation and CIP2A/AKT/mTOR signaling axis inhibition. Chin. J. Nat. Med. 17 (10), 768–777. doi:10.1016/s1875-5364(19)30093-7
Gómez-Raposo, C., Mendiola, M., Barriuso, J., Casado, E., Hardisson, D., and Redondo, A. (2009). Angiogenesis and ovarian cancer. Clin. Transl. Oncol. 11 (9), 564–571. doi:10.1007/s12094-009-0406-y
Gu, L., Feng, J., Zheng, Z., Xu, H., and Yu, W. (2016). Polyphyllin I inhibits the growth of ovarian cancer cells in nude mice. Oncol. Lett. 12 (6), 4969–4974. doi:10.3892/ol.2016.5348
Guo, Y., Liu, Z., Li, K., Cao, G., Sun, C., Cheng, G., et al. (2018). Paris polyphylla-derived saponins inhibit growth of bladder cancer cells by inducing mutant P53 degradation while up-regulating CDKN1A expression. Curr. Urol. 11 (3), 131–138. doi:10.1159/000447207
Han, J., Kan, J., Wang, X., Yuan, Q., Song, X., Huang, M., et al. (2020). Polyphyllin I suppresses proliferation and promotes apoptosis of gastric cancer cell by inhibiting stat3 phosphorylation. Transl. Cancer Res. 9 (8), 4715–4725. doi:10.21037/tcr-20-66
Han, Y., Pan, L., Ran, S., Song, Y., Sun, F. F., Wang, Y. Z., et al. (2019). Rhizoma Paridis saponins ameliorates hepatic fibrosis in rats by downregulating expression of angiogenesis‑associated growth factorsfibrosis in rats by downregulating expression of angiogenesis-associated growth factors. Mol. Med. Rep. 19 (5), 3548–3554. doi:10.3892/mmr.2019.10006
He, H., Xu, C., Zheng, L., Wang, K., Jin, M., Sun, Y., et al. (2020). Polyphyllin VII induces apoptotic cell death via inhibition of the PI3K/Akt and NF-κB pathways in A549 human lung cancer cells. Mol. Med. Rep. 21 (2), 597–606. doi:10.3892/mmr.2019.10879
He, H., Zheng, L., Sun, Y. P., Zhang, G. W., and Yue, Z. G. (2014). Steroidal saponins from Paris polyphylla suppress adhesion, migration and invasion of human lung cancer A549 cells via down-regulating MMP-2 and MMP-9. Asian Pac J. Cancer Prev. 15 (24), 10911–10916. doi:10.7314/apjcp.2014.15.24.10911
He, J., Yu, S., Guo, C., Tan, L., Song, X., Wang, M., et al. (2019). Polyphyllin I induces autophagy and cell cycle arrest via inhibiting PDK1/Akt/mTOR signal and downregulating cyclin B1 in human gastric carcinoma HGC-27 cells. Biomed. Pharmacother. 117, 109189. doi:10.1016/j.biopha.2019.109189
He, K., Wang, G. X., Zhao, L. N., Cui, X. F., Su, X. B., Shi, Y., et al. (2021). Cinobufagin is a selective anti-cancer agent against tumors with EGFR amplification and PTEN deletion. Front. Pharmacol. 12, 775602. doi:10.3389/fphar.2021.775602
Hidalgo, M., and Eckhardt, S. G. (2001). Development of matrix metalloproteinase inhibitors in cancer therapy. J. Natl. Cancer Inst. 93 (3), 178–193. doi:10.1093/jnci/93.3.178
Hong, Y., Han, Y.-Q., Wang, Y.-Z., Gao, J.-R., Li, Y.-X., Liu, Q., et al. (2016). Paridis Rhizoma Sapoinins attenuates liver fibrosis in rats by regulating the expression of RASAL1/ERK1/2 signal pathway. J. Ethnopharmacol. 192, 114–122. doi:10.1016/j.jep.2016.07.010
Islam, K. M., Anggondowati, T., Deviany, P. E., Ryan, J. E., Fetrick, A., Bagenda, D., et al. (2019). Patient preferences of chemotherapy treatment options and tolerance of chemotherapy side effects in advanced stage lung cancer. BMC Cancer 19 (1), 835. doi:10.1186/s12885-019-6054-x
Jiang, H., Zhao, P. J., Su, D., Feng, J., and Ma, S. L. (2014). Paris saponin I induces apoptosis via increasing the Bax/Bcl-2 ratio and caspase-3 expression in gefitinib-resistant non-small cell lung cancer in vitro and in vivo. Mol. Med. Rep. 9 (6), 2265–2272. doi:10.3892/mmr.2014.2108
Kang, L. P., Liu, Y. X., Eichhorn, T., Dapat, E., Yu, H. S., Zhao, Y., et al. (2012). Polyhydroxylated steroidal glycosides from Paris polyphylla. J. Nat. Prod. 75 (6), 1201–1205. doi:10.1021/np300045g
Karbowski, M., Lee, Y. J., Gaume, B., Jeong, S. Y., Frank, S., Nechushtan, A., et al. (2002). Spatial and temporal association of Bax with mitochondrial fission sites, Drp1, and Mfn2 during apoptosis. J. Cell Biol. 159 (6), 931–938. doi:10.1083/jcb.200209124
Ke, J. Y., Zhang, W., Gong, R. S., Cen, W. J., Huang, H. Q., Li, Y. R., et al. (2016). A monomer purified from Paris polyphylla (PP-22) triggers S and G2/M phase arrest and apoptosis in human tongue squamous cell carcinoma SCC-15 by activating the p38/cdc25/cdc2 and caspase 8/caspase 3 pathways. Tumour Biol. 37 (11), 14863–14872. doi:10.1007/s13277-016-5376-4
Kudo-Saito, C., Shirako, H., Takeuchi, T., and Kawakami, Y. (2009). Cancer metastasis is accelerated through immunosuppression during Snail-induced EMT of cancer cells. Cancer Cell 15 (3), 195–206. doi:10.1016/j.ccr.2009.01.023
Lai, L., Shen, Q., Wang, Y., Chen, L., Lai, J., Wu, Z., et al. (2021). Polyphyllin I reverses the resistance of osimertinib in non-small cell lung cancer cell through regulation of PI3K/Akt signaling. Toxicol. Appl. Pharmacol. 419, 115518. doi:10.1016/j.taap.2021.115518
Lai, S. W., Huang, B. R., Liu, Y. S., Lin, H. Y., Chen, C. C., Tsai, C. F., et al. (2018). Differential characterization of temozolomide-resistant human glioma cells. Int. J. Mol. Sci. 19 (1), 127. doi:10.3390/ijms19010127
Lee, M. S., Yuet-Wa, J. C., Kong, S. K., Yu, B., Eng-Choon, V. O., Nai-Ching, H. W., et al. (2005). Effects of polyphyllin D, a steroidal saponin in Paris polyphylla, in growth inhibition of human breast cancer cells and in xenograft. Cancer Biol. Ther. 4 (11), 1248–1254. doi:10.4161/cbt.4.11.2136
Li, B., Yu, B., Hui, Y., Li, M., Han, X., and Fung, K. P. (2001). An improved synthesis of the saponin, polyphyllin D. Carbohydr. Res. 331 (1), 1–7. doi:10.1016/s0008-6215(01)00014-3
Li, G. B., Fu, R. Q., Shen, H. M., Zhou, J., Hu, X. Y., Liu, Y. X., et al. (2017). Polyphyllin I induces mitophagic and apoptotic cell death in human breast cancer cells by increasing mitochondrial PINK1 levels. Oncotarget 8 (6), 10359–10374. doi:10.18632/oncotarget.14413
Li, J. H., Li, S. Y., Shen, M. X., Qiu, R. Z., Fan, H. W., and Li, Y. B. (2021a). Anti-tumor effects of Solanum nigrum L. extraction on C6 high-grade glioma. J. Ethnopharmacol. 274, 114034. doi:10.1016/j.jep.2021.114034
Li, X., Liu, Y., Liao, S., Lin, C., Moro, A., Liu, J., et al. (2021b). Polyphyllin VII induces apoptosis and autophagy via mediating H2O2 levels and the JNK pathway in human osteosarcoma U2OS cells. Oncol. Rep. 45 (1), 180–190. doi:10.3892/or.2020.7866
Li, Y., Gu, J. F., Zou, X., Wu, J., Zhang, M. H., Jiang, J., et al. (2013). The anti-lung cancer activities of steroidal saponins of P. Polyphylla smith var. chinensis (franch.) hara through enhanced immunostimulation in experimental lewis tumor-bearing C57bl/6 mice and induction of apoptosis in the A549 cell line. Molecules 18 (10), 12916–12936. doi:10.3390/molecules181012916
Li, Y., Man, S., Li, J., Chai, H., Fan, W., Liu, Z., et al. (2014). The antitumor effect of formosanin C on HepG2 cell as revealed by 1H-NMR based metabolic profiling. Chem. Biol. Interact. 220, 193–199. doi:10.1016/j.cbi.2014.06.023
Liao, Z., Tan, Z. W., Zhu, P., and Tan, N. S. (2019). Cancer-associated fibroblasts in tumor microenvironment - accomplices in tumor malignancy. Cell Immunol. 343, 103729. doi:10.1016/j.cellimm.2017.12.003
Lin, L. T., Shi, Y. C., Choong, C. Y., and Tai, C. J. (2021). The fruits of Paris polyphylla inhibit colorectal cancer cell migration induced by fusobacterium nucleatum-derived extracellular vesicles. Molecules 26 (13), 4081. doi:10.3390/molecules26134081
Lin, L. T., Uen, W. C., Choong, C. Y., Shi, Y. C., Lee, B. H., Tai, C. J., et al. (2019). Paris polyphylla inhibits colorectal cancer cells via inducing autophagy and enhancing the efficacy of chemotherapeutic drug doxorubicin. Molecules 24 (11), 2102. doi:10.3390/molecules24112102
Lin, R. K., and Wang, Y. C. (2014). Dysregulated transcriptional and post-translational control of DNA methyltransferases in cancer. Cell Biosci. 4, 46. doi:10.1186/2045-3701-4-46
Lin, Z., Liu, Y., Li, F., Wu, J., Zhang, G., Wang, Y., et al. (2015). Anti-lung cancer effects of polyphyllin VI and VII potentially correlate with apoptosis in vitro and in vivo. Phytother. Res. 29 (10), 1568–1576. doi:10.1002/ptr.5430
Liu, J., Liu, Y., Li, H., Wei, C., Mao, A., Liu, W., et al. (2022a). Polyphyllin D induces apoptosis and protective autophagy in breast cancer cells through JNK1-Bcl-2 pathway. J. Ethnopharmacol. 282, 114591. doi:10.1016/j.jep.2021.114591
Liu, J., Zhang, Y., Chen, L., Yu, F., Li, X., Dan, T., et al. (2017). Polyphyllin I induces G2/M phase arrest and apoptosis in U251 human glioma cells via mitochondrial dysfunction and the JNK signaling pathway. Acta Biochim. Biophys. Sin. (Shanghai) 49 (6), 479–486. doi:10.1093/abbs/gmx033
Liu, Q. F., Shi, Y., Zhang, Y. Z., Song, Z. M., Su, Q., Zhang, Y., et al. (2009). A simple and rapid method for the determination of pennogenin diglycoside in rat plasma by HPLC-MS: Application to the pharmacokinetics of the extract in Gongxuening capsules. J. Chromatogr. Sci. 47 (8), 728–732. doi:10.1093/chromsci/47.8.728
Liu, W., Chai, Y., Hu, L., Wang, J., Pan, X., Yuan, H., et al. (2020). Polyphyllin VI induces apoptosis and autophagy via reactive oxygen species mediated JNK and P38 activation in glioma. Onco Targets Ther. 13, 2275–2288. doi:10.2147/ott.S243953
Liu, W., Wang, Y., Chen, J., Lin, Z., Lin, M., Lin, X., et al. (2021). Beneficial effects of gracillin from rhizoma paridis against gastric carcinoma via the potential TIPE2-mediated induction of endogenous apoptosis and inhibition of migration in BGC823 cells. Front. Pharmacol. 12, 669199. doi:10.3389/fphar.2021.669199
Liu, X., Sun, Z., Deng, J., Liu, J., Ma, K., Si, Y., et al. (2018a). Polyphyllin I inhibits invasion and epithelial-mesenchymal transition via CIP2A/PP2A/ERK signaling in prostate cancer. Int. J. Oncol. 53 (3), 1279–1288. doi:10.3892/ijo.2018.4464
Liu, Y., Cao, Y., Kai, H., Han, Y., Huang, M., Gao, L., et al. (2022b). Polyphyllin E inhibits proliferation, migration and invasion of ovarian cancer cells by down-regulating the AKT/NF-κB pathway. Biol. Pharm. Bull. 45 (5), 561–568. doi:10.1248/bpb.b21-00691
Liu, Y., Dong, X., Wang, W., You, L., Yin, X., Yang, C., et al. (2018b). Molecular mechanisms of apoptosis in HepaRG cell line induced by polyphyllin VI via the Fas death pathway and mitochondrial-dependent pathway. Toxins (Basel) 10 (5), 201. doi:10.3390/toxins10050201
Liu, Z., Gao, W., Man, S., Wang, J., Li, N., Yin, S., et al. (2012a). Pharmacological evaluation of sedative-hypnotic activity and gastro-intestinal toxicity of Rhizoma Paridis saponins. J. Ethnopharmacol. 144 (1), 67–72. doi:10.1016/j.jep.2012.08.027
Liu, Z., Gao, W., Man, S., Zhang, Y., Li, H., Wu, S., et al. (2014). Synergistic effects of Rhizoma Paridis and Rhizoma Curcuma longa on different animal tumor models. Environ. Toxicol. Pharmacol. 38 (1), 31–40. doi:10.1016/j.etap.2014.04.026
Liu, Z., Li, N., Gao, W., Man, S., Yin, S., and Liu, C. (2012b). Comparative study on hemostatic, cytotoxic and hemolytic activities of different species of Paris L. J. Ethnopharmacol. 142 (3), 789–794. doi:10.1016/j.jep.2012.05.065
Long, F. Y., Chen, Y. S., Zhang, L., Kuang, X., Yu, Y., Wang, L. F., et al. (2015). Pennogenyl saponins induce cell cycle arrest and apoptosis in human hepatocellular carcinoma HepG2 cells. J. Ethnopharmacol. 162, 112–120. doi:10.1016/j.jep.2014.12.065
Lou, W., Chen, Y., Zhu, K. Y., Deng, H., Wu, T., and Wang, J. (2017). Polyphyllin I overcomes EMT-associated resistance to erlotinib in lung cancer cells via IL-6/STAT3 pathway inhibition. Biol. Pharm. Bull. 40 (8), 1306–1313. doi:10.1248/bpb.b17-00271
Man, S., Fan, W., Gao, W., Li, Y., Wang, Y., Liu, Z., et al. (2014a). Anti-fibrosis and anti-cirrhosis effects of Rhizoma paridis saponins on diethylnitrosamine induced rats. J. Ethnopharmacol. 151 (1), 407–412. doi:10.1016/j.jep.2013.10.051
Man, S., Fan, W., Liu, Z., Gao, W., Li, Y., Zhang, L., et al. (2014b). Antitumor pathway of Rhizoma Paridis Saponins based on the metabolic regulatory network alterations in H22 hepatocarcinoma mice. Steroids 84, 17–21. doi:10.1016/j.steroids.2014.03.005
Man, S., Gao, W., Yan, Y., Liu, Z., and Liu, C. (2011a). Inhibition of matrix metalloproteinases related to metastasis by diosgenyl and pennogenyl saponins. J. Ethnopharmacol. 137 (3), 1221–1227. doi:10.1016/j.jep.2011.07.045
Man, S., Gao, W., Zhang, Y., Liu, Z., Yan, L., Huang, L., et al. (2011b). Formosanin C-inhibited pulmonary metastasis through repression of matrix metalloproteinases on mouse lung adenocarcinoma. Cancer Biol. Ther. 11 (6), 592–598. doi:10.4161/cbt.11.6.14668
Man, S., Gao, W., Zhang, Y., Yan, L., Ma, C., Liu, C., et al. (2009). Antitumor and antimetastatic activities of Rhizoma Paridis saponins. Steroids 74 (13-14), 1051–1056. doi:10.1016/j.steroids.2009.08.004
Man, S., Li, J., Fan, W., Chai, H., Liu, Z., and Gao, W. (2015). Inhibition of pulmonary adenoma in diethylnitrosamine-induced rats by Rhizoma paridis saponins. J. Steroid Biochem. Mol. Biol. 154, 62–67. doi:10.1016/j.jsbmb.2015.07.004
Man, S., Li, J., Liu, J., Chai, H., Liu, Z., Wang, J., et al. (2016). Curcumin alleviated the toxic reaction of Rhizoma Paridis saponins in a 45-day subchronic toxicological assessment of rats. Environ. Toxicol. 31 (12), 1935–1943. doi:10.1002/tox.22194
Man, S., Li, J., Qiu, P., Liu, J., Liu, Z., Ma, L., et al. (2017). Inhibition of lung cancer in diethylnitrosamine-induced mice by Rhizoma paridis saponins. Mol. Carcinog. 56 (5), 1405–1413. doi:10.1002/mc.22601
Man, S., Li, Y., Fan, W., Gao, W., Liu, Z., Li, N., et al. (2013). Curcuma increasing antitumor effect of Rhizoma paridis saponins through absorptive enhancement of paridis saponins. Int. J. Pharm. 454 (1), 296–301. doi:10.1016/j.ijpharm.2013.06.079
Man, S., Lv, P., Cui, J., Liu, F., Peng, L., Ma, L., et al. (2020). Paris saponin II-induced paraptosis-associated cell death increased the sensitivity of cisplatin. Toxicol. Appl. Pharmacol. 406, 115206. doi:10.1016/j.taap.2020.115206
Misra, S., and Sharma, K. (2014). COX-2 signaling and cancer: New players in old arena. Curr. Drug Targets 15 (3), 347–359. doi:10.2174/1389450115666140127102915
Mrugala, M. M. (2013). Advances and challenges in the treatment of glioblastoma: A clinician's perspective. Discov. Med. 15 (83), 221–230.
Niibe, Y., and Hayakawa, K. (2010). Oligometastases and oligo-recurrence: The new era of cancer therapy. Jpn. J. Clin. Oncol. 40 (2), 107–111. doi:10.1093/jjco/hyp167
Niu, W., Xu, L., Li, J., Zhai, Y., Sun, Z., Shi, W., et al. (2020). Polyphyllin II inhibits human bladder cancer migration and invasion by regulating EMT-associated factors and MMPs. Oncol. Lett. 20 (3), 2928–2936. doi:10.3892/ol.2020.11839
Pang, D., Li, C., Yang, C., Zou, Y., Feng, B., Li, L., et al. (2019). Polyphyllin VII promotes apoptosis and autophagic cell death via ROS-inhibited AKT activity, and sensitizes glioma cells to temozolomide. Oxid. Med. Cell Longev. 2019, 1805635. doi:10.1155/2019/1805635
Potente, M., Gerhardt, H., and Carmeliet, P. (2011). Basic and therapeutic aspects of angiogenesis. Cell 146 (6), 873–887. doi:10.1016/j.cell.2011.08.039
Qian, X., Zhu, L., Hu, J., Li, M., Xie, L., Wang, L., et al. (2012). Rhizoma Paridis ethanol extract selectively inhibits the proliferation of HUVECs comparing to Lovo cells and shows anti-angiogenesis effects in a mouse model. J. Ethnopharmacol. 143 (1), 256–261. doi:10.1016/j.jep.2012.06.032
Qiao, X., Qu, C., Luo, Q., Wang, Y., Yang, J., Yang, H., et al. (2021). UHPLC-qMS spectrum-effect relationships for Rhizoma Paridis extracts. J. Pharm. Biomed. Analysis 194, 113770. doi:10.1016/j.jpba.2020.113770
Qin, X.-J., Sun, D.-J., Ni, W., Chen, C.-X., Hua, Y., He, L., et al. (2012). Steroidal saponins with antimicrobial activity from stems and leaves of Paris polyphylla var. yunnanensis. Steroids 77 (12), 1242–1248. doi:10.1016/j.steroids.2012.07.007
Qin, X. J., Ni, W., Chen, C. X., and Liu, H. Y. (2018). Seeing the light: Shifting from wild rhizomes to extraction of active ingredients from above-ground parts of Paris polyphylla var. yunnanensis. J. Ethnopharmacol. 224, 134–139. doi:10.1016/j.jep.2018.05.028
Qin, X. J., Yu, M. Y., Ni, W., Yan, H., Chen, C. X., Cheng, Y. C., et al. (2016). Steroidal saponins from stems and leaves of Paris polyphylla var. yunnanensis. Phytochemistry 121, 20–29. doi:10.1016/j.phytochem.2015.10.008
Qiu, P., Man, S., Yang, H., Fan, W., Yu, P., and Gao, W. (2016). Utilization of metabonomics to identify serum biomarkers in murine H22 hepatocarcinoma and deduce antitumor mechanism of Rhizoma Paridis saponins. Chem. Biol. Interact. 256, 55–63. doi:10.1016/j.cbi.2016.06.026
Shi, W. Y., Xiao, D., Wang, L., Dong, L. H., Yan, Z. X., Shen, Z. X., et al. (2012). Therapeutic metformin/AMPK activation blocked lymphoma cell growth via inhibition of mTOR pathway and induction of autophagy. Cell Death Dis. 3 (3), e275. doi:10.1038/cddis.2012.13
Shuli, M., Wenyuan, G., Yanjun, Z., Chaoyi, M., Liu, Y., and Yiwen, L. (2011). Paridis saponins inhibiting carcinoma growth and metastasis in vitro and in vivo. Arch. Pharm. Res. 34 (1), 43–50. doi:10.1007/s12272-011-0105-4
Siegel, R. L., Miller, K. D., and Jemal, A. (2020). Cancer statistics, 2020. CA Cancer J. Clin. 70 (1), 7–30. doi:10.3322/caac.21590
Siu, F. M., Ma, D. L., Cheung, Y. W., Lok, C. N., Yan, K., Yang, Z., et al. (2008). Proteomic and transcriptomic study on the action of a cytotoxic saponin (polyphyllin D): Induction of endoplasmic reticulum stress and mitochondria-mediated apoptotic pathways. Proteomics 8 (15), 3105–3117. doi:10.1002/pmic.200700829
Su, F., Ye, L., Zhou, Z., Su, A., Gu, J., Guo, Z., et al. (2022). Study of chemical compositions and anticancer effects of Paris polyphylla var. Chinensis leaves. Molecules 27 (9), 2724. doi:10.3390/molecules27092724
Sun, C.-L., Ni, W., Yan, H., Liu, Z.-H., Yang, L., Si, Y.-A., et al. (2014). Steroidal saponins with induced platelet aggregation activity from the aerial parts of Paris verticillata. Steroids 92, 90–95. doi:10.1016/j.steroids.2014.09.008
Sun, J., Liu, B. R., Hu, W. J., Yu, L. X., and Qian, X. P. (2007). In vitro anticancer activity of aqueous extracts and ethanol extracts of fifteen traditional Chinese medicines on human digestive tumor cell lines. Phytother. Res. 21 (11), 1102–1104. doi:10.1002/ptr.2196
Tan, G. X., Wang, X. N., Tang, Y. Y., Cen, W. J., Li, Z. H., Wang, G. C., et al. (2019). PP-22 promotes autophagy and apoptosis in the nasopharyngeal carcinoma cell line CNE-2 by inducing endoplasmic reticulum stress, downregulating STAT3 signaling, and modulating the MAPK pathway. J. Cell Physiol. 234 (3), 2618–2630. doi:10.1002/jcp.27076
Teng, W. J., Chen, P., Zhu, F. Y., Di, K., Zhou, C., Zhuang, J., et al. (2015). Effect of Rhizoma paridis total saponins on apoptosis of colorectal cancer cells and imbalance of the JAK/STAT3 molecular pathway induced by IL-6 suppression. Genet. Mol. Res. 14 (2), 5793–5803. doi:10.4238/2015.May.29.11
Tian, Y., Gong, G. Y., Ma, L. L., Wang, Z. Q., Song, D., and Fang, M. Y. (2020). Anti-cancer effects of Polyphyllin I: An update in 5 years. Chem. Biol. Interact. 316, 108936. doi:10.1016/j.cbi.2019.108936
Torre, L. A., Siegel, R. L., Ward, E. M., and Jemal, A. (2016). Global cancer incidence and mortality rates and trends--an update. Cancer Epidemiol. Biomarkers Prev. 25 (1), 16–27. doi:10.1158/1055-9965.Epi-15-0578
Wang, G., Liu, Y., Wang, Y., and Gao, W. (2018a). Effect of Rhizoma Paridis saponin on the pain behavior in a mouse model of cancer pain. RSC Adv. 8 (31), 17060–17072. doi:10.1039/c8ra00797g
Wang, P., Yang, Q., Du, X., Chen, Y., and Zhang, T. (2019a). Targeted regulation of Rell2 by microRNA-18a is implicated in the anti-metastatic effect of polyphyllin VI in breast cancer cells. Eur. J. Pharmacol. 851, 161–173. doi:10.1016/j.ejphar.2019.02.041
Wang, Q., Zhou, X., Zhao, Y., Xiao, J., Lu, Y., Shi, Q., et al. (2018b). Polyphyllin I ameliorates collagen-induced arthritis by suppressing the inflammation response in macrophages through the NF-κB pathway. Front. Immunol. 9, 2091. doi:10.3389/fimmu.2018.02091
Wang, W., Dong, X., You, L., Sai, N., Leng, X., Yang, C., et al. (2019b). Apoptosis in HepaRG and HL-7702 cells inducted by polyphyllin II through caspases activation and cell-cycle arrest. J. Cell Physiol. 234 (5), 7078–7089. doi:10.1002/jcp.27462
Wang, W., Liu, Y., Sun, M., Sai, N., You, L., Dong, X., et al. (2019c). Hepatocellular toxicity of Paris saponins I, II, VI and VII on two kinds of hepatocytes-HL-7702 and HepaRG cells, and the underlying mechanisms. Cells 8 (7), 690. doi:10.3390/cells8070690
Wei, J. C., Gao, W. Y., Yan, X. D., Wang, Y., Jing, S. S., and Xiao, P. G. (2014). Chemical constituents of plants from the genus Paris. Chem. Biodivers. 11 (9), 1277–1297. doi:10.1002/cbdv.201300083
Wen, F., Chen, T., Yin, H., Lin, J., and Zhang, H. (2019). In vitro effects on thrombin of Paris saponins and in vivo hemostatic activity evaluation of Paris fargesii var. brevipetala. Mol. [Online] 24 (7), 1420. doi:10.3390/molecules24071420
Wu, X., Wang, L., Wang, H., Dai, Y., Ye, W. C., and Li, Y. L. (2012). Steroidal saponins from Paris polyphylla var. yunnanensis. Phytochemistry 81, 133–143. doi:10.1016/j.phytochem.2012.05.034
Wu, Y., Si, Y., Xiang, Y., Zhou, T., Liu, X., Wu, M., et al. (2020). Polyphyllin I activates AMPK to suppress the growth of non-small-cell lung cancer via induction of autophagy. Arch. Biochem. Biophys. 687, 108285. doi:10.1016/j.abb.2020.108285
Xiang, S., Zou, P., Wu, J., Zheng, F., Tang, Q., Zhou, J., et al. (2018). Crosstalk of NF-κB/P65 and LncRNA HOTAIR-mediated repression of MUC1 expression contribute to synergistic inhibition of castration-resistant prostate cancer by polyphyllin 1-enzalutamide combination treatment. Cell Physiol. Biochem. 47 (2), 759–773. doi:10.1159/000490028
Xiang, Y., Guo, Z., Zhu, P., Chen, J., and Huang, Y. (2019). Traditional Chinese medicine as a cancer treatment: Modern perspectives of ancient but advanced science. Cancer Med. 8 (5), 1958–1975. doi:10.1002/cam4.2108
Xiao, T., Zhong, W., Zhao, J., Qian, B., Liu, H., Chen, S., et al. (2018). Polyphyllin I suppresses the formation of vasculogenic mimicry via Twist1/VE-cadherin pathway. Cell Death Dis. 9 (9), 906. doi:10.1038/s41419-018-0902-5
Xiao, X., Yang, M., Xiao, J., Zou, J., Huang, Q., Yang, K., et al. (2014). Paris Saponin II suppresses the growth of human ovarian cancer xenografts via modulating VEGF-mediated angiogenesis and tumor cell migration. Cancer Chemother. Pharmacol. 73 (4), 807–818. doi:10.1007/s00280-014-2408-x
Xiao, X., Zou, J., Bui-Nguyen, T. M., Bai, P., Gao, L., Liu, J., et al. (2012). Paris saponin II of Rhizoma Paridis--a novel inducer of apoptosis in human ovarian cancer cells. Biosci. Trends 6 (4), 201–211. doi:10.5582/bst.2012.v6.4.201
Xie, Z. Z., Li, M. M., Deng, P. F., Wang, S., Wang, L., Lu, X. P., et al. (2017). Paris saponin-induced autophagy promotes breast cancer cell apoptosis via the Akt/mTOR signaling pathway. Chem. Biol. Interact. 264, 1–9. doi:10.1016/j.cbi.2017.01.004
Yan, S., Tian, S., Kang, Q., Xia, Y., Li, C., Chen, Q., et al. (2015). Rhizoma paridis saponins suppresses tumor growth in a rat model of N-Nitrosomethylbenzylamine-Induced esophageal cancer by inhibiting cyclooxygenases-2 pathway. PLoS One 10 (7), e0131560. doi:10.1371/journal.pone.0131560
Yang, M., Zou, J., Zhu, H., Liu, S., Wang, H., Bai, P., et al. (2015). Paris saponin II inhibits human ovarian cancer cell-induced angiogenesis by modulating NF-κB signaling. Oncol. Rep. 33 (5), 2190–2198. doi:10.3892/or.2015.3836
Yang, Q., Chen, W., Xu, Y., Lv, X., Zhang, M., and Jiang, H. (2018a). Polyphyllin I modulates MALAT1/STAT3 signaling to induce apoptosis in gefitinib-resistant non-small cell lung cancer. Toxicol. Appl. Pharmacol. 356, 1–7. doi:10.1016/j.taap.2018.07.031
Yang, Y., Jiang, C., Yang, Y., Guo, L., Huang, J., Liu, X., et al. (2018b). Silencing of LncRNA-HOTAIR decreases drug resistance of Non-Small Cell Lung Cancer cells by inactivating autophagy via suppressing the phosphorylation of ULK1. Biochem. Biophys. Res. Commun. 497 (4), 1003–1010. doi:10.1016/j.bbrc.2018.02.141
Yao, J., Man, S., Dong, H., Yang, L., Ma, L., and Gao, W. (2018). Combinatorial treatment of Rhizoma Paridis saponins and sorafenib overcomes the intolerance of sorafenib. J. Steroid Biochem. Mol. Biol. 183, 159–166. doi:10.1016/j.jsbmb.2018.06.010
Yao, N., Zhou, J., Jiang, Y., Jin, Q., Zhu, H., Zhang, J., et al. (2022). Rhizoma Paridis saponins suppresses vasculogenic mimicry formation and metastasis in osteosarcoma through regulating miR-520d-3p/MIG-7 axis. J. Pharmacol. Sci. 150 (3), 180–190. doi:10.1016/j.jphs.2022.08.005
Yao, Q., Chang, B. T., Chen, R., Wei, Y. J., Gong, Q. J., Yu, D., et al. (2021). Research advances in Pharmacology, safety, and clinical applications of yunnan Baiyao, a traditional Chinese medicine formula. Front. Pharmacol. 12, 773185. doi:10.3389/fphar.2021.773185
You, L. J., Geng, H., Yang, X. F., Wei, G. L., Zhu, Y. D., Ge, G. B., et al. (2021). The comparison analysis of polyphyllin I and its analogues induced apoptosis of colon and lung cancer cells via mitochondrial dysfunction. Basic Clin. Pharmacol. Toxicol. 129 (1), 15–25. doi:10.1111/bcpt.13596
Yu, L., Ma, J., Han, J., Wang, B., Chen, X., Gao, C., et al. (2016). Licochalcone B arrests cell cycle progression and induces apoptosis in human breast cancer MCF-7 cells. Recent Pat. Anticancer Drug Discov. 11 (4), 444–452. doi:10.2174/1574892811666160906091405
Yuan, Y. L., Jiang, N., Li, Z. Y., Song, Z. Z., Yang, Z. H., Xue, W. H., et al. (2019). Polyphyllin VI induces apoptosis and autophagy in human osteosarcoma cells by modulation of ROS/JNK activation. Drug Des. Devel Ther. 13, 3091–3103. doi:10.2147/dddt.S194961
Zeng, Y., Zhang, Z., Wang, W., You, L., Dong, X., Yin, X., et al. (2020). Underlying mechanisms of apoptosis in HepG2 cells induced by polyphyllin I through Fas death and mitochondrial pathways. Toxicol. Mech. Methods 30 (6), 397–406. doi:10.1080/15376516.2020.1747125
Zhang, C., Jia, X., Bao, J., Chen, S., Wang, K., Zhang, Y., et al. (2016a). Polyphyllin VII induces apoptosis in HepG2 cells through ROS-mediated mitochondrial dysfunction and MAPK pathways. BMC Complement. Altern. Med. 16, 58. doi:10.1186/s12906-016-1036-x
Zhang, C., Jia, X., Wang, K., Bao, J., Li, P., Chen, M., et al. (2016b). Polyphyllin VII induces an autophagic cell death by activation of the JNK pathway and inhibition of PI3K/AKT/mTOR pathway in HepG2 cells. PLoS One 11 (1), e0147405. doi:10.1371/journal.pone.0147405
Zhang, C., Li, C., Jia, X., Wang, K., Tu, Y., Wang, R., et al. (2019). In vitro and in vivo anti-inflammatory effects of polyphyllin VII through downregulating MAPK and NF-κB pathways. Mol. [Online] 24 (5), 875. doi:10.3390/molecules24050875
Zhang, D., Li, K., Sun, C., Cao, G., Qi, Y., Lin, Z., et al. (2018a). Anti-cancer effects of Paris polyphylla ethanol extract by inducing cancer cell apoptosis and cycle arrest in prostate cancer cells. Curr. Urol. 11 (3), 144–150. doi:10.1159/000447209
Zhang, J., Yang, Y., Lei, L., and Tian, M. (2015). Rhizoma paridis saponins induces cell cycle arrest and apoptosis in non-small cell lung carcinoma A549 cells. Med. Sci. Monit. 21, 2535–2541. doi:10.12659/msm.895084
Zhang, N., and Xiao, X. H. (2021). Integrative medicine in the era of cancer immunotherapy: Challenges and opportunities. J. Integr. Med. 19 (4), 291–294. doi:10.1016/j.joim.2021.03.005
Zhang, S., Lu, Y., Li, H., Ji, Y., Fang, F., Tang, H., et al. (2020). A steroidal saponin form Paris vietnamensis (Takht.) reverses temozolomide resistance in glioblastoma cells via inducing apoptosis through ROS/PI3K/Akt pathway. Biosci. Trends 14 (2), 123–133. doi:10.5582/bst.2020.01005
Zhang, T., Liu, H., Liu, X. T., Xu, D. R., Chen, X. Q., and Wang, Q. (2010). Qualitative and quantitative analysis of steroidal saponins in crude extracts from Paris polyphylla var. yunnanensis and P. polyphylla var. chinensis by high performance liquid chromatography coupled with mass spectrometry. J. Pharm. Biomed. Anal. 51 (1), 114–124. doi:10.1016/j.jpba.2009.08.020
Zhang, W., Zhang, D., Ma, X., Liu, Z., Li, F., and Wu, D. (2014). Paris saponin VII suppressed the growth of human cervical cancer Hela cells. Eur. J. Med. Res. 19 (1), 41. doi:10.1186/2047-783x-19-41
Zhang, X. B., Shen, T., Zhou, X., Tang, X. H., Gao, R., Xu, L., et al. (2020). Network pharmacology based virtual screening of active constituents of Prunella vulgaris L. and the molecular mechanism against breast cancer. Sci. Rep. 10 (1), 15730. doi:10.1038/s41598-020-72797-8
Zhang, Y., Huang, P., Liu, X., Xiang, Y., Zhang, T., Wu, Y., et al. (2018b). Polyphyllin I inhibits growth and invasion of cisplatin-resistant gastric cancer cells by partially inhibiting CIP2A/PP2A/Akt signaling axis. J. Pharmacol. Sci. 137 (3), 305–312. doi:10.1016/j.jphs.2018.07.008
Zhao, L., Liu, Z., Deng, X., Wang, J., Sun, L., Fan, L., et al. (2021). Polyphyllin VII induces mitochondrial apoptosis by regulating the PP2A/AKT/DRP1 signaling axis in human ovarian cancer. Oncol. Rep. 45 (2), 513–522. doi:10.3892/or.2020.7879
Zhao, P., Jiang, H., Su, D., Feng, J., Ma, S., and Zhu, X. (2015). Inhibition of cell proliferation by mild hyperthermia at 43˚C with Paris Saponin I in the lung adenocarcinoma cell line PC-9. Mol. Med. Rep. 11 (1), 327–332. doi:10.3892/mmr.2014.2655
Zhao, Y., Kang, L. P., Liu, Y. X., Liang, Y. G., Tan, D. W., Yu, Z. Y., et al. (2009). Steroidal saponins from the rhizome of Paris polyphylla and their cytotoxic activities. Planta Med. 75 (4), 356–363. doi:10.1055/s-0028-1088380
Zhao, Y., Tang, X., Huang, Y., Tang, Q., Ma, C., Zheng, F., et al. (2019). Interaction of c-jun and HOTAIR- increased expression of p21 converge in polyphyllin I-inhibited growth of human lung cancer cells. Onco Targets Ther. 12, 10115–10127. doi:10.2147/ott.S226830
Keywords: Chinese medicine Rhizoma paridis, Rhizoma paridis saponins, polyphyllin I, polyphyllin II, polyphyllin VI, polyphyllin VII
Citation: Li J, Jia J, Zhu W, Chen J, Zheng Q and Li D (2023) Therapeutic effects on cancer of the active ingredients in rhizoma paridis. Front. Pharmacol. 14:1095786. doi: 10.3389/fphar.2023.1095786
Received: 11 November 2022; Accepted: 10 February 2023;
Published: 21 February 2023.
Edited by:
Junmin Zhang, Lanzhou University, ChinaReviewed by:
Chao Zhang, China Pharmaceutical University, ChinaIti Gupta, Indian Institute of Technology Gandhinagar, India
Copyright © 2023 Li, Jia, Zhu, Chen, Zheng and Li. This is an open-access article distributed under the terms of the Creative Commons Attribution License (CC BY). The use, distribution or reproduction in other forums is permitted, provided the original author(s) and the copyright owner(s) are credited and that the original publication in this journal is cited, in accordance with accepted academic practice. No use, distribution or reproduction is permitted which does not comply with these terms.
*Correspondence: Qiusheng Zheng, zqsyt@sohu.com; Defang Li, lidefang@163.com
†These authors have contributed equally to this work