- 1Åstrand Laboratory, The Swedish School of Sport and Health Sciences, Stockholm, Sweden
- 2Department of Physiology and Pharmacology, Karolinska Institutet, Stockholm, Sweden
- 3Department of Physical Education, University of Las Palmas de Gran Canaria, Las Palmas, Spain
- 4Research Institute of Biomedical and Health Sciences (IUIBS), Las Palmas de Gran Canaria, Gran Canaria, Spain
- 5School of Kinesiology, Faculty of Education, The University of British Columbia, Vancouver, BC, Canada
- 6Swedish Winter Sports Research Centre, Department of Health Sciences, Mid Sweden University, Östersund, Sweden
Sexual dimorphism is apparent in humans, however, to date no studies have investigated mitochondrial function focusing on intrinsic mitochondrial respiration (i.e., mitochondrial respiration for a given amount of mitochondrial protein) and mitochondrial oxygen affinity (p50mito) in relation to biological sex in human. A skeletal muscle biopsy was donated by nine active women, and ten men matched for maximal oxygen consumption (VO2max) and by nine endurance trained men. Intrinsic mitochondrial respiration, assessed in isolated mitochondria, was higher in women compared to men when activating complex I (CIP) and complex I+II (CI+IIP) (p < 0.05), and was similar to trained men (CIP, p = 0.053; CI+IIP, p = 0.066). Proton leak and p50mito were higher in women compared to men independent of VO2max. In conclusion, significant novel differences in mitochondrial oxidative function, intrinsic mitochondrial respiration and p50mito exist between women and men. These findings may represent an adaptation in the oxygen cascade in women to optimize muscle oxygen uptake to compensate for a lower oxygen delivery during exercise.
Introduction
Sexual dimorphism of anatomical and physiological characteristics is apparent in humans as the result of differential gene expression between males and females (Rigby and Kulathinal, 2015). Importantly, the phenotypic differences between women and men have repercussions on life expectancy (Seifarth et al., 2012), disease occurrence and aging (Popkov et al., 2015; Tower, 2017). Nevertheless, women are significantly less studied than men in all disciplines of medical research (Costello et al., 2014) which highlights the need for further research in this field (Clayton and Collins, 2014).
Since the seminal work of Holloszy (1967) it has been shown that endurance exercise training enhances muscle oxidative capacity, through upregulation of both the activity and content of several mitochondrial enzymes, adaptations of which are quickly reversed by detraining (Holloszy and Booth, 1976; Henriksson and Reitman, 1977; Granata et al., 2016). To date, most studies have been conducted in men, without attention to potential physiological differences between sexes (Della Torre and Maggi, 2017). There are known sex-specific metabolic differences in skeletal muscle. For example, it has been shown that women oxidize more fatty acids and less carbohydrates than men at the same relative exercise workload (Tarnopolsky et al., 1990; Horton et al., 1998) potentially due to a higher mitochondrial content (Montero et al., 2018), higher baseline lipoprotein lipase levels (Skelly et al., 2017), estrogen (Ruby et al., 1997), and glycerol (Roepstorff et al., 2002) compared to men with similar cardiorespiratory fitness. Transcription and translation of proteins involved in muscle lipid metabolism are also sex dependent (Kiens et al., 2004) with women possessing a higher number of intramyocellular lipid droplets compared to men (Tarnopolsky et al., 2007). Likewise, a bout of endurance exercise has been shown to acutely increase the mRNA content of citrate synthase (CS) and β-hydroxyacyl-CoA dehydrogenase (HAD) to a larger extent in women compared to men (Roepstorff et al., 2005). However, others have reported similar gene expression in women and men following a bout of sprint interval exercise (Scalzo et al., 2014; Skelly et al., 2017) and similar signaling responses (Fuentes et al., 2012) with the exception of the exercise response of mRNA content of glucose transport 4, lipoprotein lipase and Atrogin-1, which have been shown to be sex-specific (Skelly et al., 2017). 5′AMP-activated protein kinase (AMPK), which acts as a cellular energy sensor, responds more robustly in men than women following similar relative energetic stress suggesting that women better preserve muscle cellular homeostasis compared to men following an exercise bout (Roepstorff et al., 2006). While the activity of mitochondrial enzymes such as 3-beta-Hydroxyacyl CoA dehydrogenase, complex II-III, cytochrome c oxidase, and CS activity are similarly improved by endurance training in men and women (McKenzie et al., 2000; Carter et al., 2001; Skelly et al., 2017), a greater muscle protein synthesis and mitochondrial biogenesis has been reported in men compared to women following sprint interval training (Scalzo et al., 2014). Although animal models have consistently shown the superiority of mitochondrial function of females compare to males (e.g., higher oxidative capacity, lower ROS production, higher fatty acid utilization, lower ADP-stimulated respiration in female compared to male) (Ventura-Clapier et al., 2017), data in humans is limited.
One study compared oxidative phosphorylation in the isolated mitochondrial preparation from gastrocnemius muscle finding no differences between men and women (Thompson et al., 2013). However, men and women were not matched according to any physiological criteria and all subjects had endothelial dysfunction. These two factors may have influenced the study outcomes. Two recent studies using the permeabilized fiber technique found that women possess similar maximal oxidative phosphorylation capacity per unit muscle mass (Miotto et al., 2018; Montero et al., 2018), but lower ADP sensitivity and different substrate sensitivity independent of maximal oxidative phosphorylation and mitochondrial content or protein level compared to men.
Recent findings in Drosophila melanogaster indicate that male mitochondria harbor a higher mutational load due to the maternal transmission of mitochondrial genes (Innocenti et al., 2011) which impact mitochondrial respiration and intrinsic mitochondrial oxidative phosphorylation capacity (i.e., mitochondrial respiration for a given amount of mitochondrial protein). Intrinsic mitochondrial function has not been directly assessed and compared in women and men with similar fitness level. A critical mitochondrial property that is less often investigated is the mitochondrial affinity for oxygen defined as the oxygen tension were mitochondrial respiration is 50% of its maximum (p50mito). Seminal work by Gnaiger et al. (1995) demonstrated the interdependence between mitochondrial efficiency and p50mito; mitochondrial coupling and thus mitochondrial efficiency is directly related to p50mito.
Extending these findings to the whole-body level, it has been shown that a low O2 affinity (high p50mito), is associated with a low basal metabolic rate (Schiffer et al., 2016) and high aerobic efficiency during exercise (Larsen et al., 2011). Furthermore, it has been recently shown that a high mitochondrial O2 affinity enhances O2 extraction during exercise (Cardinale et al., 2018b). The mechanistic basis for the association between p50mito and efficiency can be explained by the presence of different isoforms of cytochrome c oxidase subunit IV where isoform IV-1 is associated with high affinity for oxygen but a low efficiency, whereas isoform IV-2 has a low affinity for oxygen but high efficiency (Schiffer et al., 2016). An alternative explanation based on thermodynamic processes indicates that a trade-off exists between the catalytic efficiency of an enzymatic process and its substrate affinity (Stucki et al., 1983). In other words, metabolic processes cannot achieve maximum efficiency and maximum power production simultaneously. Since it is well established that women have a higher efficiency during exercise (Åstrand et al., 2003; Fares et al., 2017) and lower basal metabolic rate per unit body mass (Benedict and Emmes, 1915), a logical premise is that women should have a higher p50mito than men. Lowering the basal metabolic rate and increasing exercise efficiency with a higher p50mito would be advantageous in women during periods of low energy availability. However, p50mito in human skeletal muscle has only been reported in four studies to date (Larsen et al., 2011; Boushel et al., 2015; Schiffer et al., 2016; Cardinale et al., 2018b) and any difference between sexes have not yet been reported.
In line with the US National Institutes of Health call on integrating sex as a biological variable into animal and human research (Clayton and Collins, 2014), the aim of this paper was to examine if sex-based differences exist in mass-specific mitochondrial oxidative phosphorylation, intrinsic mitochondrial capacity (i.e., mitochondrial quality; mitochondrial respiration for a given amount of mitochondrial protein) and p50mito. To address this question, we compared skeletal muscle mitochondrial function in women and men with a similar maximal oxygen uptake (VO2max), and men with a higher VO2max. We hypothesized that women would show similar mass-specific mitochondrial oxidative phosphorylation capacity, but a higher intrinsic mitochondrial respiration and higher p50mito compared to men with similar VO2max. We further hypothesized that women would have lower mass-specific and intrinsic mitochondrial respiration as well as a lower p50mito compared to men with higher VO2max.
Materials and Methods
Subjects
A group of active women (Women n = 9) was matched to a group of men (Men n = 10) with similar maximal oxygen consumption (VO2max) and compared for all measured outcomes. The group of women all exercised regularly mainly running and cycling three or four times each week and some women were involved in competitive endurance events. All of the women tested in this study had a menstrual cycle of normal length (self-reported) and did not use hormonal contraceptives during and 3 months prior to the study period. It has been demonstrated previously that significant inter-and intra-subject variability exists with respect to ovarian hormone levels throughout the menstrual cycle that has effects on whole-body metabolism (Macnutt et al., 2012). As such, we tested women at random points throughout their menstrual cycle. The group of men all exercised recreationally (running, cycling, etc.) two or three times each week but none trained regularly for any particular sporting event. Further comparisons were made including a third group of endurance trained men (Trained men n = 9) with higher VO2max compared to both the women and men groups. This third group was comprised of trained cyclists who trained about 7–9 times each week, 60–300 min per training session over the past 5–10 years and all competed in endurance events. Subject characteristics are presented in Table 1. All subjects were determined healthy based on a health screening survey and were informed about possible risks and discomfort involved before giving their written consent to participate in this study. The study was undertaken according to the Declaration of Helsinki and was approved by the Regional Ethical Review Board in Stockholm and in Umeå, Sweden.
Systemic Oxygen Consumption
A graded incremental exercise test until volitional exhaustion on a cycle ergometer was used to determine VO2max except for three women who performed a graded incremental exercise on a treadmill. These three women were later tested both on a cycle ergometer and on a treadmill for VO2max assessment and their VO2max was not different between exercise modes. VO2max leveling-off criteria was applied (i.e., a VO2 plateau, followed by exercise cessation or decrease of VO2 at higher work rates, with an RER > 1.10). The maximal power output (Wmax) pedaled during the graded incremental exercise test was taken as individual cycle work capacity. O2 consumption was measured with a metabolic cart with a mixing chamber (Oxycon Pro, Jaeger GmbH, Germany), calibrated prior to each test according to the manufacturer’s instructions, with high-grade calibration gases (Air Liquide, Sweden). Respiratory variables were measured and averaged every 10 s. The highest 30 s averaged VO2 recorded was taken as the VO2max.
Muscle Biopsy Sampling
In a resting state after a minimum of 48h without any strenuous physical activity, a muscle sample was obtained from the middle portion of the vastus lateralis muscle at a depth of 2–3 cm, about one-third of the distance from the upper margin of the patella to the anterior superior iliac spine. After local anesthesia (2–4 ml Carbocaine 20 mg ml-1; Astra Zeneca, Södertälje, Sweden) an incision (0.5–1 cm) was made through the skin and fascia and a muscle sample (50–100 mg) was obtained either with the Weil-Blakesley conchotome technique or modified Bergström needle with suction (Ekblom, 2017). A part of the specimen (∼50 mg) was rapidly placed in ice-cold mitochondrial isolation medium (Sucrose 100 mM, KCl 100 mM, Tris-HCl 50 mM, KH2PO4 1 mM, EGTA 100 μM, BSA 0.1%; final pH was set to 7.4) followed by mitochondria isolation.
Isolation of Mitochondria
Mitochondrial isolation was conducted as published (Tonkonogi and Sahlin, 1997) but with a minor modification as described by Larsen et al. (2011). Briefly, the muscle biopsy was first weighed and then cut in ice-cold isolation medium (Sucrose 100 mM, KCl 100 mM, Tris-HCl 50 mM, KH2PO4 1 mM, EGTA 100 μM, BSA 0.1%; final pH was set to 7.4) (Gnaiger and Kuznetsov, 2002). One milliliter of isolation medium containing 0.2 mg ml-1 bacterial protease was then added to the homogenate and then transferred to a glass jacket connected to an ice-cold bath pump for further homogenization with a hand held electrically driven drill (80 rpm). The final homogenate was centrifuged at 700 g at 4°C for 10 min. The supernatant was again centrifuged at 10000 g at 4°C and the resultant mitochondrial pellet was re-suspended in the same medium. The Eppendorf was then centrifuged at 7000 g for 5 min and the pellet was dissolved in 0.6 μl preservation medium (EGTA 0.5 mM, MgCl2 6H2O 3 mM, K-lactobionate 60 mM, Taurine 20 mM, KH2PO4 10 mM, HEPES 20 mM, Sucrose 110 mM, BSA 1 g L-1 Histidine 20 mM, Vitamin E succinate 20 μM, Glutathione 3 mM, Leupeptine 1 μM, Glutamate 2 mM, Malate 2 mM, Mg-ATP 2 mM) (Gnaiger and Kuznetsov, 2002) per initial wet weight of the sample from where mitochondria were isolated.
Mitochondrial respiration was performed in a two-channel high resolution respirometer (Oroboros Oxygraph, Paar, Graz, Austria). Data sampling was set for 1 s intervals and averaged over 40 s. Each experiment was run twice and the O2 flux of the two chambers were then averaged. MiR05 medium (EGTA 0.5 mM, MgCl2.6H2O 3 mM, K-lactobionate 60 mM, Taurine 20 mM, KH2P04 10 mM, HEPES 20 mM, Sucrose 110 mM, BSA 1 g L-1) was used to assess respiration. All experiments were performed at 37°C. O2 consumption and zero-drift of the O2 electrode were calculated using DatLab 5.2 software (Oroboros, Paar, Graz, Austria).
Mitochondrial Respiratory Protocols
Mitochondrial respiration was measured with the titration of substrates into the chambers to assess leak respiration (L) with malate (2 mM) and pyruvate (5 mM) in the absence of adenylates; complex I respiration (CIP) with addition of saturating ADP (5 mM); state 4 respiration [respiration after state 3 (Chance and Williams, 1955) when ADP is phosphorylated to ATP], followed by convergent complex I+II linked ADP-stimulated maximal respiration (CI+IIP) with addition of succinate (10 mM). In three women the substrate titration protocol used for assessment of leak respiration was obtained in the absence of adenylates titrating palmitoylcarnitine (0.2 mM) in addition to malate and pyruvate, and CI+IIP was obtained titrating glutamate (10 mM) in addition to succinate. The respiration data from these three women are included in the current figures. Based on several experiments conducted in our laboratory we have found no effect of the slightly different substrate combinations on the results we report here. We have done a separate analysis of the current data excluding these data points and we detect the same statistical differences between groups. Mitochondrial respiration rates (pmol s-1) were normalized for mitochondrial suspension protein levels (pmol s-1 μg-1) in the isolated mitochondria preparation using the Pierce 660 nm protein assay (Thermo Fisher Scientific) to obtain the intrinsic mitochondrial respiration and additionally normalized for initial wet weight of the muscle sample (pmol s-1 mg-1) to obtain mass-specific mitochondrial respiration. The latter mass-specific respiration expressed as pmol s-1 mg-1 initial wet weight are a calculation and as such are much lower and not comparable to those reported from direct respiration measures in permeabilized fibers (Cardinale et al., 2018a). The p50mito in isolated mitochondria was defined as the O2 tension where mitochondrial respiration proceeds at 50% of the maximum rate in presence of saturating ADP concentrations. p50mito was determined by smoothing the exponential O2 flux decay corrected for time-constant, background correction, and zero oxygen calibration using DatLab 2 software (Oroboros Oxygraph, Paar, Graz, Austria).
Statistics
Results are presented as mean ± SD. The data were initially tested for normal distribution and equal variance using the Shapiro–Wilk test of normality and Q-Q plots. Not-normally distributed parameters where log transformed. One-way analysis of variance was used to assess the between group differences for the normal distributed data. A p < 0.05 was considered significant. Statistical analyses were carried out using SPSS statistical software version 21 (SPSS Inc., Chicago, IL, United States).
Results
Intrinsic Mitochondrial Respiration Differs Between Men and Women With Similar VO2max
Mitochondrial oxidative phosphorylation capacity depends on mitochondrial content and quality (i.e., intrinsic mitochondrial respiration). To test the hypothesis that women possess a higher intrinsic mitochondrial respiration compared to men with similar VO2max, we measured maximal ADP-stimulated mitochondrial respiration in isolated mitochondria. Mitochondrial respiration when providing electron donors specific to either complex I (CIP) or complex I + II (CI+IIP) normalized for mitochondrial protein concentration was higher in women (p < 0.05, Figures 1C,D) than in men with similar VO2max. We therefore sought to test if the intrinsic mitochondrial respiration in the women would be similar to men with a higher VO2max (i.e., trained men group). Interestingly, mitochondrial respiration activating CIP and CI+IIP (Figures 1C,D) did not differ between women with a VO2max of 51.0 ± 4.1 mL O2min-1 kg-1 and trained men whose VO2max was 67.1 ± 3.4 mL O2min-1 kg-1.
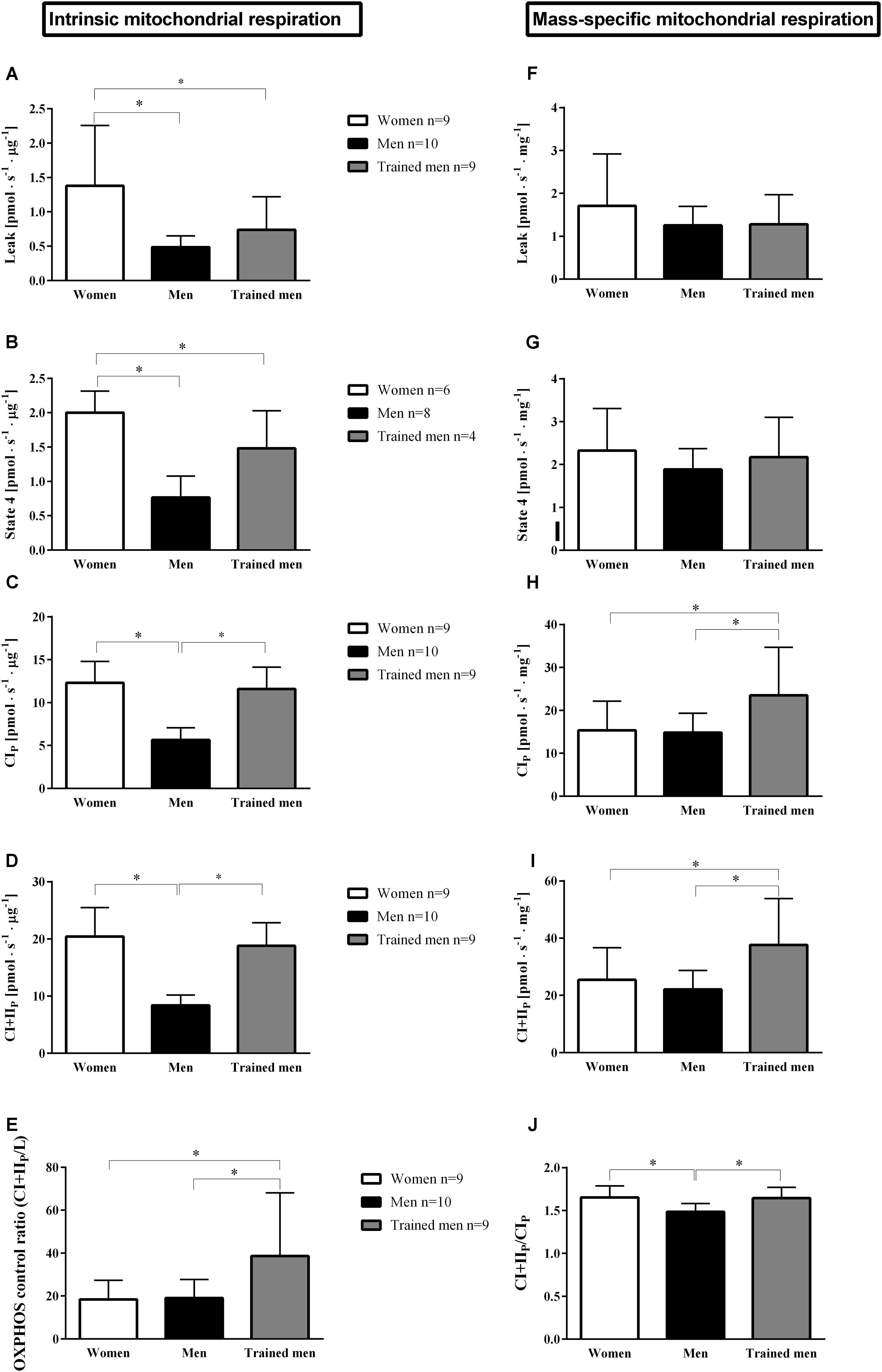
FIGURE 1. Mean ± SD of mitochondrial respiration rates normalized by protein levels of mitochondrial suspension (pmol s-1 μg protein-1; intrinsic mitochondrial oxidative respiration) and by the initial muscle wet weight (pmol s-1 mg-1; mass-specific mitochondrial respiration) in women, men, and trained men groups for: A,F, leak respiration is the respiratory rate in the presence of substrates without addition of adenylates; B,G, state 4 is the respiratory rate when ADP is phosphorylated maximally to ATP; C,H, complex IP is the maximum ADP stimulated respiration rate in the presence of complex I substrates; D,I, complex I+IIP is similar to C,H but with added convergent electron flux through complex II by adding succinate (CI+IIP); E, maximal oxidative phosphorylation capacity (OXPHOS) control ratio (CI+IIP/Leak) indicates the limitation of OXPHOS by the phosphorylation system; J, CI+IIP/CIP indicates the contribution of CII respiration to maximal respiration. ∗p < 0.05 between groups.
Mass-Specific Mitochondrial Respiration
Having established that intrinsic mitochondrial respiration was higher in women than in men with similar VO2max we tested if this factor was balanced out by a higher mitochondrial content in men compared to women. Therefore, we related the mitochondrial respiratory parameters to initial wet weight of the muscle (see section “Materials and Methods”) used in the mitochondrial isolation procedure (Figures 1F–I). Mass-specific mitochondrial respiration did not differ between women and men with similar VO2max. When compared to the trained men with higher VO2 max, women had lower mass-specific mitochondrial respiration. These results would indicate that women possess a higher intrinsic mitochondrial respiration, and a lower mitochondrial abundance resulting in similar mitochondrial respiration per wet weight for a given whole body aerobic capacity compared to men or that women do not need to increase their mitochondrial content to the same extent as men to have similar respiration per wet weight muscle since women possess higher intrinsic mitochondrial respiration.
Higher Intrinsic Proton Leak in Women Than in Men
Leak respiration (mitochondrial respiration in the absence of adenylates) has been used as a proxy for passive proton leak over the inner mitochondrial membrane. It has been estimated that proton leakage accounts for a major part of the basal metabolic rate (Porter and Brand, 1993). Since women have lower basal metabolic rate than men it is logical to assume that women should have lower mitochondrial leak respiration than men. Surprisingly, intrinsic leak respiration was significantly higher (p < 0.05) in women compared to men independent of VO2max (Figure 1A). Consistent with this finding, intrinsic state 4 respiration (respiratory rate obtained in isolated mitochondria when ADP has been phosphorylated to ATP) was higher in women than in men independent of VO2max (Figure 1B). However, when leak respiration and state 4 respiration were related to initial wet weight, no significant differences were found between sexes or groups.
The Relative Contribution of Complex II to the Total Electron Flux Is Higher in Women Compared to Men
Women and men of the same VO2max had similar respiratory control ratio (p > 0.05), measured as the ratio of leak respiration to maximal oxidative phosphorylation rate (CI+IIP/L), but respiratory control ratio was lower in women compared to the trained men (p < 0.05) (Figure 1E). When complex II is activated by addition of succinate, respiration increases compared to when respiration is only supported by complex I. The relative ratio of this increase is a measure of the relative contribution of complex II to the total electron flux and we found this ratio to be higher in women compared to men matched for VO2max, but similar to trained men (Figure 1J).
p50mito Differs Between Sexes
The discovery that women have higher mitochondrial intrinsic capacity (i.e., O2 flux per mitochondrial protein) than men with similar VO2max led us to test if this difference was linked to difference in mitochondrial O2 affinity (p50mito). We measured p50mito by titrating substrates which activate CIP and CI+IIP with saturating ADP concentrations. In women, the p50mito with complex I substrates (0.10 ± 0.05 kPa) and with complex I+II substrates (0.22 ± 0.07 kPa) was significantly higher (p < 0.05) than in men with similar VO2max (p50mito with CIP = 0.04 ± 0.01 kPa, p50mito with CI+IIP = 0.07 ± 0.02 kPa), and also higher compared to trained men with the higher VO2max (p50mito with CIP = 0.05 ± 0.02 kPa, p50mito with CI+IIP = 0.12 ± 0.03 kPa) (Figures 2A–C).
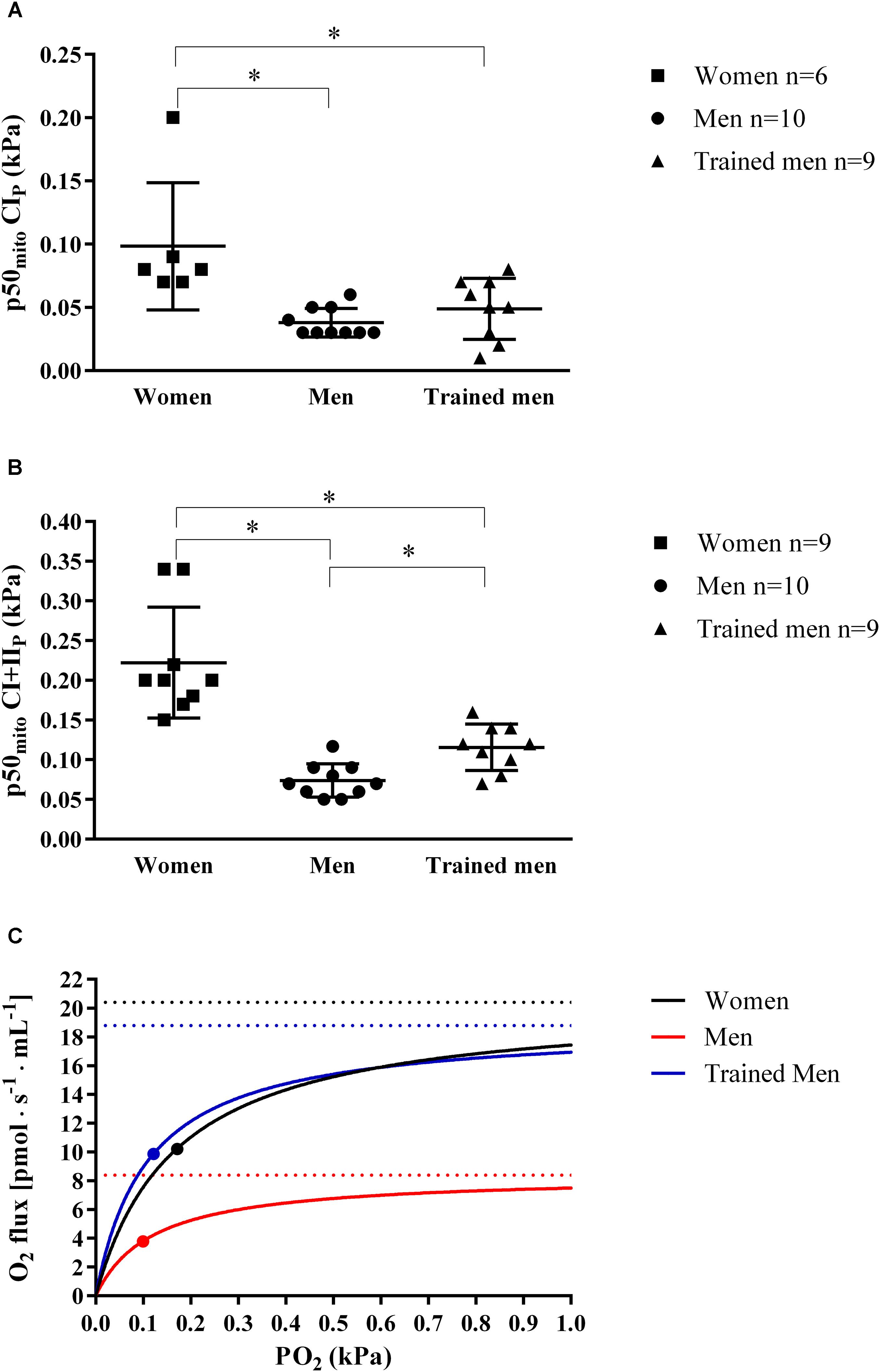
FIGURE 2. (A) Individual and mean ± SD values of ex vivo mitochondrial p50 (p50mito; kPa) at maximal ADP-induced activation measured in isolated mitochondria when activating complex I (CIP) for women, men, and trained men groups. (B) Individual and mean ± SD values of ex vivo p50mito as shown in A, but with complex I+II-linked substrate state (CI+IIP) for women, men, and trained men groups. ∗p < 0.05 between groups. (C) Hyperbolic curves showing the relation between PO2 (kPa) and mitochondrial O2 flux [pmol s-1 mL-1]. Curves were obtained inserting into the following equation [VO2 = (CI+IIP PO2)⋅(PO2 + p50mito)-1] continuous PO2 values, the ex vivo p50mito (graphically indicated with a dot) and CI+IIP (graphically indicated with a dashed lines) mean values measured in this study in women, men, and trained men groups. This figure shows the role of mitochondrial p50 for muscle oxygen consumption since for a given intracellular PO2, a lower p50mito would result in a higher tissue O2 consumption [VO2 = CI+IIP⋅PO2 (PO2 + p50mito)-1] and vice versa. The superior intrinsic mitochondrial respiration in women compared to men with similar VO2max shown in this study may be an important physiological adaptation that compensates for the higher mitochondrial p50 allowing a higher O2 extraction peripherally.
Cycle Work Capacity
It is well known that endurance trained athletes have both high cardiac output and mitochondrial respiratory capacity. It is well accepted that cardiac output is a major limiting factor in the oxygen cascade (Bassett and Howley, 2000; Saltin and Calbet, 2006). A greater mitochondrial capacity has instead been hypothesized to be more important for endurance performance and physical work capacity (Gollnick and Saltin, 1982). Since our subjects were matched for VO2max we wanted to test if cycling work capacity differed between groups due to the observed differences in mitochondrial characteristics. The maximal power output pedaled during the incremental cycling ergometer test did not differ between women and men for comparable VO2max but was significantly lower compared to the trained men group with the higher VO2max when normalized per individual body mass (Figure 3).
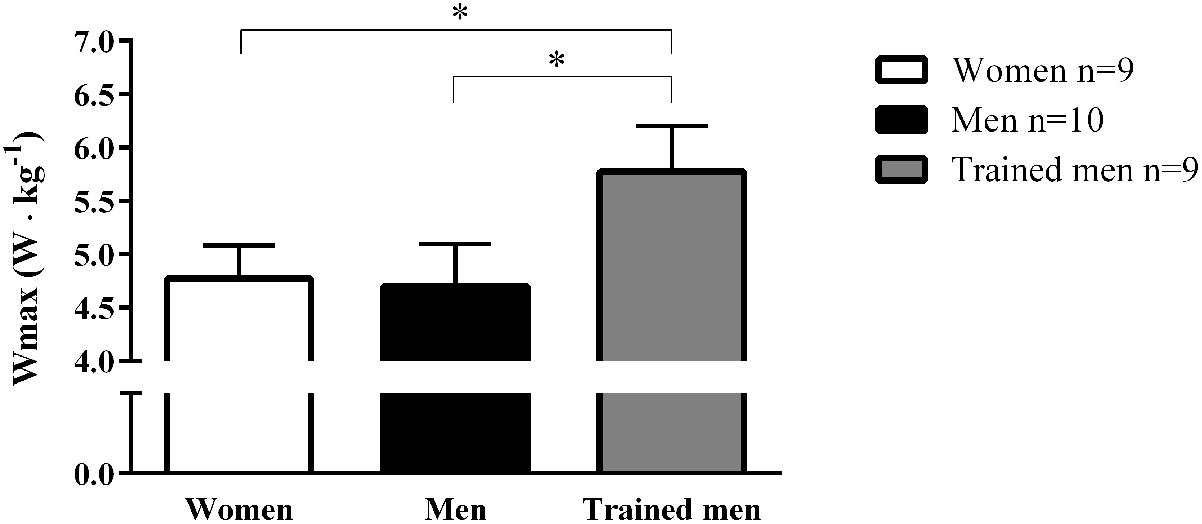
FIGURE 3. Mean ± SD values of maximal cycling power output (Wmax; W kg-1) achieved on a gradual incremental exercise test for women, men, and trained men groups. ∗p < 0.05 between groups.
In our subjects, the maximal power output pedaled during the incremental cycling was significantly correlated to the intrinsic and mass-specific mitochondrial respiration activating CI+IIP and as expected VO2max was better correlated to mass-specific mitochondrial respiration than intrinsic mitochondrial respiration (Figure 4).
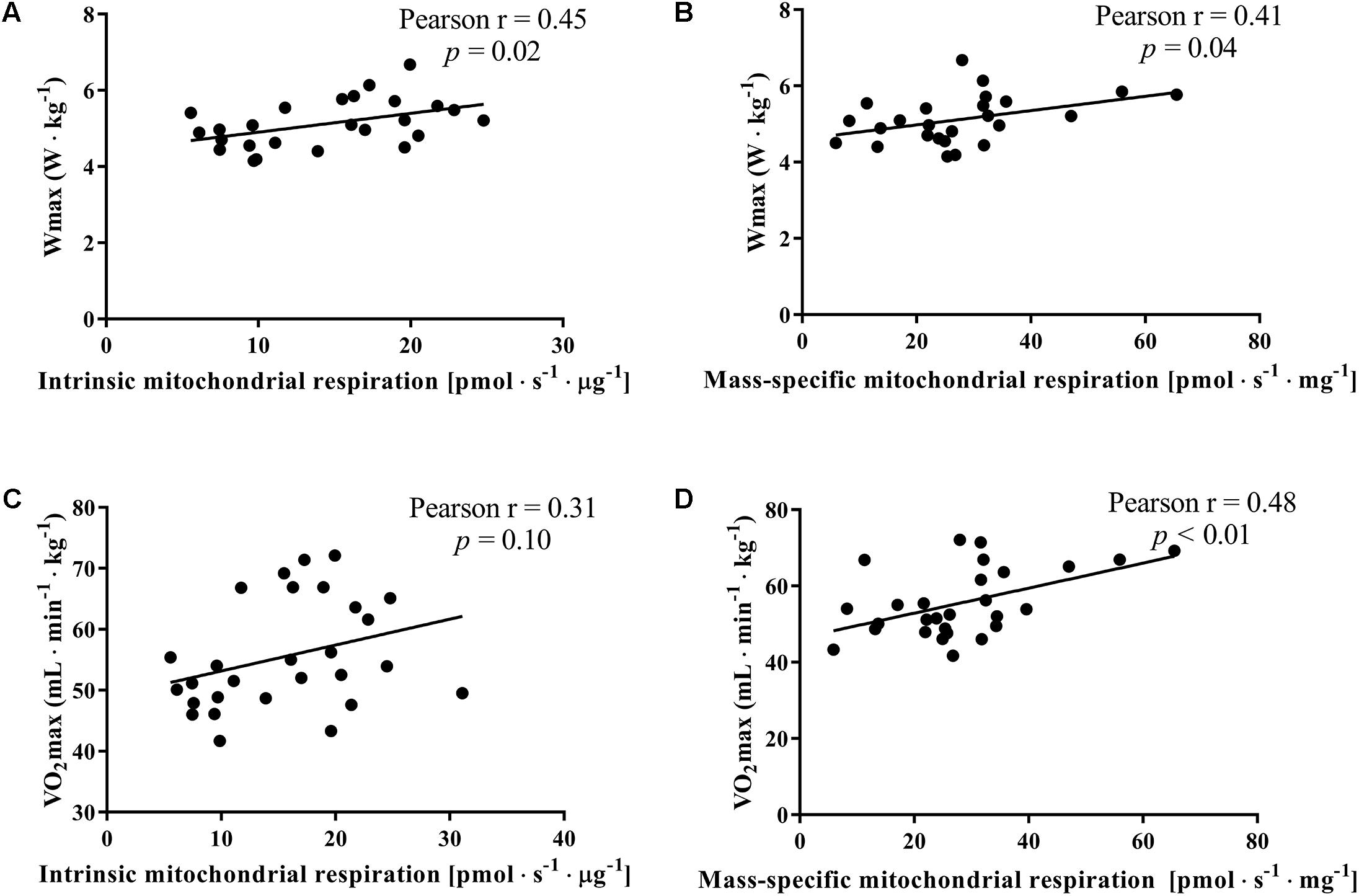
FIGURE 4. Correlation between the maximal mitochondrial oxidative phosphorylation (State 3 complex I+IIP), maximal cycling power output (Wmax) achieved on a gradual incremental exercise test and maximal oxygen consumption (VO2max) for the whole group of subjects. Maximal mitochondrial oxidative phosphorylation was normalized for mitochondrial protein content (intrinsic mitochondrial respiration; A,B) and for initial wet weight (mass-specific mitochondrial respiration; C,D).
Discussion
This study presents new insights on physiological sexual dimorphism in human skeletal muscle mitochondria. Here for the first time, we provide experimental evidence in humans that intrinsic and leak respiration are higher in women compared to men with similar mass-specific mitochondrial respiratory capacity. Furthermore, mitochondrial oxygen affinity is lower in women compared to men for a similar VO2max. When compared to endurance-trained men, women have similar intrinsic mitochondrial respiration but lower mass-specific respiration, lower mitochondrial oxygen affinity, higher intrinsic proton leak, and lower cycle work capacity.
Mitochondrial Quality in Women and Muscle Metabolism
Strong sexual dimorphism has been shown in rodent models with females exhibiting superior structural and functional mitochondria in different organs compared to males (Ventura-Clapier et al., 2017). It appears that the part of the genome regulating mitochondrial function is optimized in women since mitochondrial DNA is almost exclusively maternally inherited (Tower, 2015). The higher intrinsic mitochondrial respiration in women could be a physiological strategy to increase energy yield from fat oxidation which is usually found to be higher in women than in men during exercise at a given relative work rate (Tarnopolsky et al., 1990; Roepstorff et al., 2006). AMPK has been implicated in the regulation of fatty acid uptake, handling, and oxidation (Thomson and Winder, 2009; O’Neill et al., 2013) as well as mitochondrial biogenesis via direct phosphorylation of peroxisome proliferator activated receptor c co-activator-1a (Norrbom et al., 2011) (the transcriptional regulator of genes involved in oxidative metabolism). Therefore, a potential mechanism for the higher fat oxidation in women could reside in a greater AMPK signaling. However, no sex differences have been found in resting AMPK, while a lower AMPK activation following exercise has been reported in women compared to men (Roepstorff et al., 2006) indicating that AMPK signaling may not the be key regulator of fat oxidation during prolonged exercise in women and not differentially regulating intrinsic mitochondrial respiration in women and men. Furthermore, in a well-controlled study design, a 3h exercise bout on a cycle ergometer performed at a work intensity of 60–65% of VO2max (work intensity at which fat oxidation is expected to be maximized), resulted in no difference in fat oxidation between well-trained women and men, but a higher carbohydrate oxidation in well-trained men (Zehnder et al., 2005). It could be argued that if women have enhanced capacity for fat oxidation during exercise or possess any other muscle metabolic advantage this would give them an edge during long endurance events. However, racing times in endurance events ranging between 1500 m to marathon is ∼11% lower (faster) in men compared to women (Sparling et al., 1998). This performance gap between women and men increases to about 20% in ultra-events (mainly running and cycling events) (Knechtle et al., 2014; Zingg et al., 2014). There is some exception in swimming, especially open-water events, where women perform equally or better compared to men (Knechtle et al., 2015) likely due to the increased buoyancy in women attributed to the caudally located higher percentage of fat mass (McLean and Hinrichs, 1998). These differences are likely not explained by differences in running economy between women and men (Daniels et al., 1977; Davies and Thompson, 1979) although conflicting results have been reported (Bransford and Howley, 1977; Bhambhani and Singh, 1985).
VO2max Is a Stronger Determinant of Work Capacity Than Mitochondrial Capacity
An interesting observation is that the maximal work load during the graded incremental exercise test normalized for individual body mass, which highly relates to cycling endurance performance (Faria et al., 2005), did not differ between women and men with comparable VO2max despite a ∼143% higher intrinsic mitochondrial respiration in women. This would indicate that VO2max is a stronger determinant of work capacity than intrinsic mitochondrial respiration (Figure 4).
The Role of Skeletal Mitochondrial Oxidative Capacity and p50mito in Regulating Oxygen Consumption
The higher skeletal mitochondrial respiratory capacity in women compared to men relative to VO2max has important implications for our understanding of regulatory factors in the O2 cascade during exercise. Based on conservation of mass, a high mitochondrial respiratory capacity relative to O2 delivery indicates that at VO2max, mitochondria respire at a substantially lower relative mitochondrial respiratory capacity (Boushel et al., 2015). The higher maximal ADP-stimulated intrinsic mitochondrial respiration rate in women compared to men of similar VO2max indicates that during exercise, mitochondria isolated from women respire at a lower relative rate. In the present study we show that the in vitro maximally ADP-stimulated p50mito was higher in women (O2 affinity was lower) compared to men. However, the p50mito in vivo is also a function of the relative activation of mitochondria (at what fraction of the maximal respiratory rate the mitochondria respires at whole-body VO2max intensity). Since women show both a higher intrinsic mitochondrial respiration and a higher p50mito, these two variables may balance out in vivo. A lower relative activation of mitochondria at VO2max in vivo in women would also lower the p50 (Cardinale et al., 2018b). As recently demonstrated both mitochondrial capacity and p50mito have important implications for muscle oxygen consumption since for a given intracellular PO2, lower p50mito would result in a higher tissue O2 consumption [VO2 = CI+IIP PO2 (PO2 + p50mito)-1] (Cardinale et al., 2018b). The higher mitochondrial capacity in women may thus be an important physiological adaptation in women to permit a higher O2 extraction peripherally to counteract the more centrally mediated limitations of a higher work of breathing (Dominelli et al., 2013, 2017) and lower O2 carrying capacity of blood (Murphy, 2014). The lower relative activation of mitochondria in women during exercise could also preserve fat oxidation capacity, maintain mitochondrial efficiency, and lower ROS production.
Higher Intrinsic Proton Leak in Women Than in Men
Our results indicate an unexpected overall higher intrinsic proton leakage in women compared to men. This can be another strategy to lower ROS production by reducing the mitochondrial membrane potential and could also be linked to the longer life-span in women since the aging process has been linked to ROS-production (Finkel and Holbrook, 2000). Uncoupling protein-3 (Gong et al., 1997) and ANT (Andreyev et al., 1989; Azzu et al., 2008) are thought to dissipate energy as heat and affecting ATP production which could be responsible for the higher intrinsic proton leak in women compared to men. However, the higher proton leak compared to men did not compromise mitochondrial coupling efficiency which was similar between women and men with similar VO2max (Figure 1E). Interestingly, the contribution of complex II of the electron transfer system to the total coupled mitochondrial respiratory capacity (Figure 1J) was greater in women than in men with similar VO2max. This would indicate a functional difference of specific proteins of the electron transfer system between women and men.
These findings raise the important question of whether the observed differences between women and men are a true sex difference as a consequence of evolutionary pressure (Della Torre and Maggi, 2017) or are the result of environmental factors such as the response to exercise training or other factors such as nutrition and lifestyle.
Mitochondrial Quality: Born or Made?
Both high-volume low-intensity endurance exercise (Daussin et al., 2008; Vincent et al., 2015) and low-volume high-intensity endurance exercise (Granata et al., 2015) are strategies capable of improving mitochondrial oxidative capacity (Holloszy, 1967; Holloszy and Booth, 1976). However, cross-sectional data indicate that high-intensity training is a key factor to stimulate higher mitochondrial respiration whereas training volume is to a greater extent linked to change in mitochondrial content (i.e., higher CS activity) (Bishop et al., 2014; Vigelsø et al., 2014). Nevertheless, the improvement in mass-specific mitochondrial respiration usually reported at the end of an endurance exercise intervention usually disappears when mitochondrial respiration is normalized to a mitochondrial parameter such as mitochondrial protein content or CS activity (Granata et al., 2016; MacInnis et al., 2016). Accordingly, it is unclear which training regimen stimulates intrinsic mitochondrial respiration and which mitochondrial components underlie increased intrinsic mitochondrial respiration (Bartlett et al., 2017). A plausible locus of regulation is the mitochondrial cristae, which until recently has been thought to be of constant density relative to mitochondrial volume among individuals; however, differences between active individuals and elite athletes was recently discovered (Nielsen et al., 2016). It has been proposed that endurance exercise induces mitochondrial biogenesis and leads to the development of new mitochondria which at the initial stage become enlarged, followed by an increase in length (Glancy et al., 2015; Lundby and Jacobs, 2015), and lastly an increase in mitochondrial cristae density, since further increase in mitochondrial content would impair muscle contractile function (Nielsen et al., 2016). With this background, it is possible that women in our study may have had higher mitochondrial cristae density compared to men with similar VO2max.
In addition to the higher mitochondrial cristae density hypothesis, a second plausible explanation for the superior intrinsic mitochondrial respiration in women compared to men could be a higher abundance of supercomplexes and respirasomes (Schagger and Pfeiffer, 2000; Lobo-Jarne and Ugalde, 2018) in women. Supercomplexes are electron transport system proteins aggregated in a supermolecular assemblies which more rarely are constituted of all the required proteins to transfer electrons from NADH to molecular oxygen, termed respirasomes. The morphology of these supercomplexes is optimized to increase the mitochondrial catalytic efficiency (Bianchi et al., 2004) and their abundance can be altered by exercise training (Greggio et al., 2016).
Generally, women possess higher % body fat than men and therefore it can be speculated that the superior intrinsic mitochondrial respiration in women compared to men with similar VO2max in our study is the result of a chronic exposure of higher O2 delivery per lean muscle mass in women than in men. In support of this argument, it has been shown that mitochondria respire close to their maximal capacity when the exercised muscle is highly perfused such as in the case of one-legged knee exercise (Blomstrand et al., 2011). Furthermore, peripheral adaptations of skeletal muscle following one-legged cycling is greatly enhanced compared to double-leg cycling where lower O2 delivery per active muscle mass occur (Abbiss et al., 2011). Unfortunately, body composition assessment was not systematically measured in this study, therefore we are unable to present VO2max scaled per lean muscle mass. However, it is reasonable to assume the group of women had a higher percentage fat mass compared to trained men, and therefore women with a VO2max of 51.0 ± 4.1 mL O2 min-1 kg-1 had a similar mitochondrial quality compared to the trained men whose VO2max was 32% higher. In other words, it would not be expected that the women in this study had 32% higher body fat than the men with the higher VO2 max. This would indicate that O2 delivery per unit muscle mass should not account for the difference in mitochondrial quality observed in women and men recruited in this study.
Study Limitations
This study is not without limitations. First, we could not report VO2max scaled per lean muscle mass. Second, the research design did not control for diet between participants and menstrual cycle phase when testing women; thus, a greater variability in the measured outcomes may have been introduced by these factors. Nonetheless, these potential influences are unlikely to account for the robust magnitude of difference in intrinsic mitochondrial respiration and p50mito between women and men. The strength of this study includes the assessment of intrinsic mitochondrial respiration and p50mito which has not been previously reported when comparing women to men.
Conclusion
This study provides evidence that women may possess a superior mitochondrial quality than men with equal cardiorespiratory fitness and endurance performance. Such a difference could be due to compensatory adaptations in women in the oxygen cascade (Della Torre and Maggi, 2017). Considering the greater life expectancy (Seifarth et al., 2012), and lower disease occurrence and aging (Popkov et al., 2015; Tower, 2017) in women than in men, the present findings suggest that the reserve of mitochondrial oxidative capacity (Boushel et al., 2011) may be even more physiologically important in men (Tower, 2017). Future studies should focus on the possible in vivo physiological effects of a higher intrinsic mitochondrial respiration, identifying which mitochondrial components underlie a higher intrinsic mitochondrial respiration (e.g., supercomplexes, mitochondrial cristae density) as well as the implications of upregulating intrinsic mitochondrial respiration in diseased populations. Additional factors such as diet, potential hormonal effects associated with the menstrual cycle are important questions for future study. Whether the higher intrinsic mitochondrial function in women represents a compensatory peripheral adaptation to low blood oxygen content also remains an interesting question for related disciplines.
Author Contributions
DC, FL, and RB contributed to the conception of the study. All authors contributed to the data collection. DC analyzed, interpreted the data, and wrote the first draft of the manuscript which was reviewed by FL and RB. All authors read and approved the final manuscript. All persons designated as authors qualify for authorship, and all those who qualify for authorship are listed.
Funding
This study was funded by the Swedish National Centre for Research in Sports (P2017-0054 and P2015-0133); the Swedish Military Forces’ Research Authority (#AF9220914) and the Natural Sciences and Engineering Research Council of Canada (#15R76049).
Conflict of Interest Statement
The authors declare that the research was conducted in the absence of any commercial or financial relationships that could be construed as a potential conflict of interest.
Acknowledgments
The authors would like to thank Manne Godhe and Torbjörn Helge for their assistance during the data collection and the participants who volunteered in this study.
References
Abbiss, C. R., Karagounis, L. G., Laursen, P. B., Peiffer, J. J., Martin, D. T., Hawley, J. A., et al. (2011). Single-leg cycle training is superior to double-leg cycling in improving the oxidative potential and metabolic profile of trained skeletal muscle. J. Appl. Physiol. (1985) 110, 1248–1255. doi: 10.1152/japplphysiol.01247.2010
Andreyev, A., Bondareva, T. O., Dedukhova, V. I., Mokhova, E. N., Skulachev, V. P., Tsofina, L. M., et al. (1989). The ATP/ADP-antiporter is involved in the uncoupling effect of fatty acids on mitochondria. Eur. J. Biochem. 182, 585–592. doi: 10.1111/j.1432-1033.1989.tb14867.x
Åstrand, P.-O., Rodahl, K., Dahl, H. A., and Strømme, S. B. (2003). Textbook of Work Physiology. Champaign, IL: Human Kinetics.
Azzu, V., Parker, N., and Brand, M. D. (2008). High membrane potential promotes alkenal-induced mitochondrial uncoupling and influences adenine nucleotide translocase conformation. Biochem. J. 413, 323–332. doi: 10.1042/BJ20080321
Bartlett, M. F., Miehm, J. D., Fitzgerald, L. F., and Straight, C. R. (2017). Do changes in mitochondrial quality contribute to increases in skeletal muscle oxidative capacity following endurance training? J. Physiol. 595, 1861–1862. doi: 10.1113/JP273809
Bassett, D. R., and Howley, E. T. (2000). Limiting factors for maximum oxygen uptake and determinants of endurance performance. Med. Sci. Sports Exerc. 32, 70–84. doi: 10.1097/00005768-200001000-00012
Benedict, F. G., and Emmes, L. E. (1915). A comparison of the basal metabolism of normal men and women. Proc. Natl. Acad. Sci. U.S.A. 1, 104–105. doi: 10.1073/pnas.1.2.104
Bhambhani, Y., and Singh, M. (1985). Metabolic and cinematographic analysis of walking and running in men and women. Med. Sci. Sports Exerc. 17, 131–137. doi: 10.1249/00005768-198502000-00021
Bianchi, C., Genova, M. L., Parenti Castelli, G., and Lenaz, G. (2004). The mitochondrial respiratory chain is partially organized in a supercomplex assembly: kinetic evidence using flux control analysis. J. Biol. Chem. 279, 36562–36569. doi: 10.1074/jbc.M405135200
Bishop, D. J., Granata, C., and Eynon, N. (2014). Can we optimise the exercise training prescription to maximise improvements in mitochondria function and content? Biochim. Biophys. Acta 1840, 1266–1275. doi: 10.1016/j.bbagen.2013.10.012
Blomstrand, E., Krustrup, P., Sondergaard, H., Radegran, G., Calbet, J. A., and Saltin, B. (2011). Exercise training induces similar elevations in the activity of oxoglutarate dehydrogenase and peak oxygen uptake in the human quadriceps muscle. Pflugers. Arch. 462, 257–265. doi: 10.1007/s00424-011-0978-6
Boushel, R., Gnaiger, E., Calbet, J. A., Gonzalez-Alonso, J., Wright-Paradis, C., Sondergaard, H., et al. (2011). Muscle mitochondrial capacity exceeds maximal oxygen delivery in humans. Mitochondrion 11, 303–307. doi: 10.1016/j.mito.2010.12.006
Boushel, R., Gnaiger, E., Larsen, F. J., Helge, J. W., González-Alonso, J., Ara, I., et al. (2015). Maintained peak leg and pulmonary VO2 despite substantial reduction in muscle mitochondrial capacity. Scand. J. Med. Sci. Sports 25(Suppl. 4), 135–143. doi: 10.1111/sms.12613
Bransford, D. R., and Howley, E. T. (1977). Oxygen cost of running in trained and untrained men and women. Med. Sci. Sports 9, 41–44. doi: 10.1249/00005768-197721000-00007
Cardinale, D. A., Gejl, K. D., Ortenblad, N., Ekblom, B., Blomstrand, E., and Larsen, F. J. (2018a). Reliability of maximal mitochondrial oxidative phosphorylation in permeabilized fibers from the vastus lateralis employing high-resolution respirometry. Physiol. Rep. 6:e13611. doi: 10.14814/phy2.13611
Cardinale, D. A., Larsen, F. J., Jensen-Urstad, M., Rullman, E., Sondergaard, H., Morales-Alamo, D., et al. (2018b). Muscle mass and inspired oxygen influence oxygen extraction at maximal exercise: role of mitochondrial oxygen affinity. Acta Physiol. (Oxf.) 4:e13110. doi: 10.1111/apha.13110
Carter, S. L., Rennie, C. D., Hamilton, S. J., and Tarnopolsky. (2001). Changes in skeletal muscle in males and females following endurance training. Can. J. Physiol. Pharmacol. 79, 386–392. doi: 10.1139/y01-008
Chance, B., and Williams, G. R. (1955). Respiratory enzymes in oxidative phosphorylation. III. The steady state. J. Biol. Chem. 217, 409–427.
Clayton, J. A., and Collins, F. S. (2014). Policy: NIH to balance sex in cell and animal studies. Nature 509, 282–283. doi: 10.1038/509282a
Costello, J. T., Bieuzen, F., and Bleakley, C. M. (2014). Where are all the female participants in sports and exercise Medicine research? Eur. J. Sport Sci. 14, 847–851. doi: 10.1080/17461391.2014.911354
Daniels, J., Krahenbuhl, G., Foster, C., Gilbert, J., and Daniels, S. (1977). Aerobic responses of female distance runners to submaximal and maximal exercise. Ann. N. Y. Acad. Sci. 301, 726–733. doi: 10.1111/j.1749-6632.1977.tb38242.x
Daussin, F. N., Zoll, J., Dufour, S. P., Ponsot, E., Lonsdorfer-Wolf, E., Doutreleau, S., et al. (2008). Effect of interval versus continuous training on cardiorespiratory and mitochondrial functions: relationship to aerobic performance improvements in sedentary subjects. Am. J. Physiol. Regul. Integr. Comp. Physiol. 295, R264–R272. doi: 10.1152/ajpregu.00875.2007
Davies, C. T., and Thompson, M. W. (1979). Aerobic performance of female marathon and male ultramarathon athletes. Eur. J. Appl. Physiol. Occup. Physiol. 41, 233–245. doi: 10.1007/BF00429740
Della Torre, S., and Maggi, A. (2017). Sex differences: a resultant of an evolutionary pressure? Cell Metab. 25, 499–505. doi: 10.1016/j.cmet.2017.01.006
Dominelli, P. B., Archiza, B., Ramsook, A. H., Mitchell, R. A., Peters, C. M., Molgat-Seon, Y., et al. (2017). Effects of respiratory muscle work on respiratory and locomotor blood flow during exercise. Exp. Physiol. 102, 1535–1547. doi: 10.1113/EP086566
Dominelli, P. B., Foster, G. E., Dominelli, G. S., Henderson, W. R., Koehle, M. S., Mckenzie, D. C., et al. (2013). Exercise-induced arterial hypoxaemia and the mechanics of breathing in healthy young women. J. Physiol. 591, 3017–3034. doi: 10.1113/jphysiol.2013.252767
Ekblom, B. (2017). The muscle biopsy technique. Historical and methodological considerations. Scand. J. Med. Sci. Sports 27, 458–461. doi: 10.1111/sms.12808
Fares, E. J., Isacco, L., Monnard, C. R., Miles-Chan, J. L., Montani, J. P., Schutz, Y., et al. (2017). Reliability of low-power cycling efficiency in energy expenditure phenotyping of inactive men and women. Physiol. Rep. 5:e13233. doi: 10.14814/phy2.13233
Faria, E. W., Parker, D. L., and Faria, I. E. (2005). The science of cycling: physiology and training - part 1. Sports Med. 35, 285–312. doi: 10.2165/00007256-200535040-00002
Finkel, T., and Holbrook, N. J. (2000). Oxidants, oxidative stress and the biology of ageing. Nature 408, 239–247. doi: 10.1038/35041687
Fuentes, T., Guerra, B., Ponce-Gonzalez, J. G., Morales-Alamo, D., Guadalupe-Grau, A., Olmedillas, H., et al. (2012). Skeletal muscle signaling response to sprint exercise in men and women. Eur. J. Appl. Physiol. 112, 1917–1927. doi: 10.1007/s00421-011-2164-0
Glancy, B., Hartnell, L. M., Malide, D., Yu, Z. X., Combs, C. A., Connelly, P. S., et al. (2015). Mitochondrial reticulum for cellular energy distribution in muscle. Nature 523, 617–620. doi: 10.1038/nature14614
Gnaiger, E., and Kuznetsov, A. V. (2002). Mitochondrial respiration at low levels of oxygen and cytochrome c. Biochem. Soc. Trans. 30, 252–258. doi: 10.1042/bst0300252
Gnaiger, E., Steinlechner-Maran, R., Méndez, G., Eberl, T., and Margreiter, R. (1995). Control of mitochondrial and cellular respiration by oxygen. J. Bioenerg. Biomembr. 27, 583–596. doi: 10.1007/BF02111656
Gollnick, P. D., and Saltin, B. (1982). Significance of skeletal muscle oxidative enzyme enhancement with endurance training. Clin. Physiol. 2, 1–12. doi: 10.1111/j.1475-097X.1982.tb00001.x
Gong, D. W., He, Y., Karas, M., and Reitman, M. (1997). Uncoupling protein-3 is a mediator of thermogenesis regulated by thyroid hormone, beta3-adrenergic agonists, and leptin. J. Biol. Chem. 272, 24129–24132. doi: 10.1074/jbc.272.39.24129
Granata, C., Oliveira, R. S., Little, J. P., Renner, K., and Bishop, D. J. (2015). Training intensity modulates changes in PGC-1α and p53 protein content and mitochondrial respiration, but not markers of mitochondrial content in human skeletal muscle. FASEB J. 30, 929–970.
Granata, C., Oliveira, R. S., Little, J. P., Renner, K., and Bishop, D. J. (2016). Mitochondrial adaptations to high-volume exercise training are rapidly reversed after a reduction in training volume in human skeletal muscle. FASEB J. 30, 3413–3423. doi: 10.1096/fj.201500100R
Greggio, C., Jha, P., Kulkarni, S. S., Lagarrigue, S., Broskey, N. T., Boutant, M., et al. (2016). Enhanced respiratory chain supercomplex formation in response to exercise in human skeletal muscle. Cell Metab. 25, 301–311. doi: 10.1016/j.cmet.2016.11.004
Henriksson, J., and Reitman, J. S. (1977). Time course of changes in human skeletal muscle succinate dehydrogenase and cytochrome oxidase activities and maximal oxygen uptake with physical activity and inactivity. Acta Physiol. Scand. 99, 91–97. doi: 10.1111/j.1748-1716.1977.tb10356.x
Holloszy, J. O. (1967). Biochemical adaptations in muscle. Effects of exercise on mitochondrial oxygen uptake and respiratory enzyme activity in skeletal muscle. J. Biol. Chem. 242, 2278–2282.
Holloszy, J. O., and Booth, F. W. (1976). Biochemical adaptations to endurance exercise in muscle. Annu. Rev. Physiol. 38, 273–291. doi: 10.1146/annurev.ph.38.030176.001421
Horton, T. J., Pagliassotti, M. J., Hobbs, K., and Hill, J. O. (1998). Fuel metabolism in men and women during and after long-duration exercise. J. Appl. Physiol. (1985) 85, 1823–1832. doi: 10.1152/jappl.1998.85.5.1823
Innocenti, P., Morrow, E. H., and Dowling, D. K. (2011). Experimental evidence supports a sex-specific selective sieve in mitochondrial genome evolution. Science 332, 845–848. doi: 10.1126/science.1201157
Kiens, B., Roepstorff, C., Glatz, J. F., Bonen, A., Schjerling, P., Knudsen, J., et al. (2004). Lipid-binding proteins and lipoprotein lipase activity in human skeletal muscle: influence of physical activity and gender. J. Appl. Physiol. (1985) 97, 1209–1218. doi: 10.1152/japplphysiol.01278.2003
Knechtle, B., Rosemann, T., and Rust, C. A. (2015). Women cross the ‘Catalina Channel’ faster than men. Springerplus 4:332. doi: 10.1186/s40064-015-1086-4
Knechtle, B., Zingg, M. A., Rosemann, T., and Rüst, C. A. (2014). Sex difference in top performers from Ironman to double deca iron ultra-triathlon. Open Access J. Sports Med. 5, 159–172. doi: 10.2147/OAJSM.S65977
Larsen, F. J., Schiffer, T. A., Sahlin, K., Ekblom, B., Weitzberg, E., and Lundberg, J. O. (2011). Mitochondrial oxygen affinity predicts basal metabolic rate in humans. FASEB J. 25, 2843–2852. doi: 10.1096/fj.11-182139
Lobo-Jarne, T., and Ugalde, C. (2018). Respiratory chain supercomplexes: structures, function and biogenesis. Semin. Cell Dev. Biol 76, 179–190. doi: 10.1016/j.semcdb.2017.07.021
Lundby, C., and Jacobs, R. A. (2015). Adaptations of skeletal muscle mitochondria to exercise training. Exp. Physiol. 10, 17–22.
MacInnis, M. J., Zacharewicz, E., Martin, B. J., Haikalis, M. E., Skelly, L. E., Tarnopolsky, M. A., et al. (2016). Superior mitochondrial adaptations in human skeletal muscle after interval compared to continuous single-leg cycling matched for total work. J. Physiol. 595, 2955–2966. doi: 10.1113/JP272570
Macnutt, M. J., De Souza, M. J., Tomczak, S. E., Homer, J. L., and Sheel, A. W. (2012). Resting and exercise ventilatory chemosensitivity across the menstrual cycle. J. Appl. Physiol. 112, 737–747. doi: 10.1152/japplphysiol.00727.2011
McKenzie, S., Phillips, S. M., Carter, S. L., Lowther, S., Gibala, M. J., and Tarnopolsky, M. A. (2000). Endurance exercise training attenuates leucine oxidation and BCOAD activation during exercise in humans. Am. J. Physiol. Endocrinol. Metab. 278, E580–E587. doi: 10.1152/ajpendo.2000.278.4.E580
McLean, S. P., and Hinrichs, R. N. (1998). Sex differences in the centre of buoyancy location of competitive swimmers. J. Sports Sci. 16, 373–383. doi: 10.1080/02640419808559365
Miotto, P. M., Mcglory, C., Holloway, T. M., Phillips, S. M., and Holloway, G. P. (2018). Sex-differences in mitochondrial respiratory function in human skeletal muscle. Am. J. Physiol. Regul. Integr. Comp. Physiol. 314, R909–R915. doi: 10.1152/ajpregu.00025.2018
Montero, D., Madsen, K., Meinild-Lundby, A. K., Edin, F., and Lundby, C. (2018). Sex dimorphism of substrate utilization: differences in skeletal muscle mitochondrial volume density and function. Exp. Physiol. 103, 851–859. doi: 10.1113/EP087007
Murphy, W. G. (2014). The sex difference in haemoglobin levels in adults - mechanisms, causes, and consequences. Blood Rev. 28, 41–47. doi: 10.1016/j.blre.2013.12.003
Nielsen, J., Gejl, K. D., Hey-Mogensen, M., Holmberg, H. C., Suetta, C., Krustrup, P., et al. (2016). Plasticity in mitochondrial cristae density allows metabolic capacity modulation in human skeletal muscle. J. Physiol. 595, 2839–2847. doi: 10.1113/JP273040
Norrbom, J., Sallstedt, E. K., Fischer, H., Sundberg, C. J., Rundqvist, H., and Gustafsson, T. (2011). Alternative splice variant PGC-1alpha-b is strongly induced by exercise in human skeletal muscle. Am. J. Physiol. Endocrinol. Metab. 301, E1092–E1098. doi: 10.1152/ajpendo.00119.2011
O’Neill, H. M., Holloway, G. P., and Steinberg, G. R. (2013). AMPK regulation of fatty acid metabolism and mitochondrial biogenesis: implications for obesity. Mol. Cell. Endocrinol. 366, 135–151. doi: 10.1016/j.mce.2012.06.019
Popkov, V. A., Plotnikov, E. Y., Silachev, D. N., Zorova, L. D., Pevzner, I. B., Jankauskas, S. S., et al. (2015). Diseases and aging: gender matters. Biochemistry (Mosc) 80, 1560–1570. doi: 10.1134/S0006297915120032
Porter, R. K., and Brand, M. D. (1993). Body mass dependence of H+ leak in mitochondria and its relevance to metabolic rate. Nature 362, 628–630. doi: 10.1038/362628a0
Rigby, N., and Kulathinal, R. J. (2015). Genetic architecture of sexual dimorphism in humans. J. Cell. Physiol. 230, 2304–2310. doi: 10.1002/jcp.24979
Roepstorff, C., Schjerling, P., Vistisen, B., Madsen, M., Steffensen, C. H., Rider, M. H., et al. (2005). Regulation of oxidative enzyme activity and eukaryotic elongation factor 2 in human skeletal muscle: influence of gender and exercise. Acta Physiol. Scand. 184, 215–224. doi: 10.1111/j.1365-201X.2005.01442.x
Roepstorff, C., Steffensen, C. H., Madsen, M., Stallknecht, B., Kanstrup, I. L., Richter, E. A., et al. (2002). Gender differences in substrate utilization during submaximal exercise in endurance-trained subjects. Am. J. Physiol. Endocrinol. Metab. 282, E435–E447. doi: 10.1152/ajpendo.00266.2001
Roepstorff, C., Thiele, M., Hillig, T., Pilegaard, H., Richter, E. A., Wojtaszewski, J. F., et al. (2006). Higher skeletal muscle alpha2AMPK activation and lower energy charge and fat oxidation in men than in women during submaximal exercise. J. Physiol. 574, 125–138. doi: 10.1113/jphysiol.2006.108720
Ruby, B. C., Robergs, R. A., Waters, D. L., Burge, M., Mermier, C., and Stolarczyk, L. (1997). Effects of estradiol on substrate turnover during exercise in amenorrheic females. Med. Sci. Sports Exerc. 29, 1160–1169. doi: 10.1097/00005768-199709000-00007
Saltin, B., and Calbet, J. A. L. (2006). Point: In health and in a normoxic environment, VO2 max is limited primarily by cardiac output and locomotor muscle blood flow. J. Appl. Physiol. 100, 744–745. doi: 10.1152/japplphysiol.01395.2005
Scalzo, R. L., Peltonen, G. L., Binns, S. E., Shankaran, M., Giordano, G. R., Hartley, D. A., et al. (2014). Greater muscle protein synthesis and mitochondrial biogenesis in males compared with females during sprint interval training. FASEB J. 28, 2705–2714. doi: 10.1096/fj.13-246595
Schagger, H., and Pfeiffer, K. (2000). Supercomplexes in the respiratory chains of yeast and mammalian mitochondria. EMBO J. 19, 1777–1783. doi: 10.1093/emboj/19.8.1777
Schiffer, T. A., Peleli, M., Sundqvist, M. L., Ekblom, B., Lundberg, J. O., Weitzberg, E., et al. (2016). Control of human energy expenditure by cytochrome c oxidase subunit IV-2. Am. J. Physiol. Cell Physiol. 311, C452–C461. doi: 10.1152/ajpcell.00099.2016
Seifarth, J. E., Mcgowan, C. L., and Milne, K. J. (2012). Sex and life expectancy. Gend Med. 9, 390–401. doi: 10.1016/j.genm.2012.10.001
Skelly, L. E., Gillen, J. B., Macinnis, M. J., Martin, B. J., Safdar, A., Akhtar, M., et al. (2017). Effect of sex on the acute skeletal muscle response to sprint interval exercise. Exp. Physiol. 102, 354–365. doi: 10.1113/EP086118
Sparling, P. B., O’donnell, E. M., and Snow, T. K. (1998). The gender difference in distance running performance has plateaued: an analysis of world rankings from 1980 to 1996. Med. Sci. Sports Exerc. 30, 1725–1729. doi: 10.1097/00005768-199812000-00011
Stucki, J. W., Compiani, M., and Caplan, S. R. (1983). Efficiency of energy conversion in model biological pumps. Optimization by linear nonequilibrium thermodynamic relations. Biophys. Chem. 18, 101–109. doi: 10.1016/0301-4622(83)85003-0
Tarnopolsky, L. J., Macdougall, J. D., Atkinson, S. A., Tarnopolsky, M. A., and Sutton, J. R. (1990). Gender differences in substrate for endurance exercise. J. Appl. Physiol. (1985) 68, 302–308. doi: 10.1152/jappl.1990.68.1.302
Tarnopolsky, M. A., Rennie, C. D., Robertshaw, H. A., Fedak-Tarnopolsky, S. N., Devries, M. C., and Hamadeh, M. J. (2007). Influence of endurance exercise training and sex on intramyocellular lipid and mitochondrial ultrastructure, substrate use, and mitochondrial enzyme activity. Am. J. Physiol. Regul. Integr. Comp. Physiol. 292, R1271–R1278. doi: 10.1152/ajpregu.00472.2006
Thompson, J. R., Swanson, S. A., Casale, G. P., Johanning, J. M., Papoutsi, E., Koutakis, P., et al. (2013). Gastrocnemius mitochondrial respiration: are there any differences between men and women? J. Surg. Res. 185, 206–211. doi: 10.1016/j.jss.2013.05.054
Thomson, D. M., and Winder, W. W. (2009). AMP-activated protein kinase control of fat metabolism in skeletal muscle. Acta Physiol. (Oxf.) 196, 147–154. doi: 10.1111/j.1748-1716.2009.01973.x
Tonkonogi, M., and Sahlin, K. (1997). Rate of oxidative phosphorylation in isolated mitochondria from human skeletal muscle: effect of training status. Acta Physiol. Scand. 161, 345–353. doi: 10.1046/j.1365-201X.1997.00222.x
Tower, J. (2015). Mitochondrial maintenance failure in aging and role of sexual dimorphism. Arch. Biochem. Biophys. 576, 17–31. doi: 10.1016/j.abb.2014.10.008
Tower, J. (2017). Sex-specific gene expression and life span regulation. Trends Endocrinol. Metab. 28, 735–747. doi: 10.1016/j.tem.2017.07.002
Ventura-Clapier, R., Moulin, M., Piquereau, J., Lemaire, C., Mericskay, M., Veksler, V., et al. (2017). Mitochondria: a central target for sex differences in pathologies. Clin. Sci. (Lond.) 131, 803–822. doi: 10.1042/CS20160485
Vigelsø, A., Andersen, N. B., and Dela, F. (2014). The relationship between skeletal muscle mitochondrial citrate synthase activity and whole body oxygen uptake adaptations in response to exercise training. Int. J. Physiol. Pathophysiol. Pharmacol. 6, 84–101.
Vincent, G., Lamon, S., Gant, N., Vincent, P. J., Macdonald, J. R., Markworth, J. F., et al. (2015). Changes in mitochondrial function and mitochondria associated protein expression in response to 2-weeks of high intensity interval training. Front. Physiol. 6:51. doi: 10.3389/fphys.2015.00051
Zehnder, M., Ith, M., Kreis, R., Saris, W., Boutellier, U., and Boesch, C. (2005). Gender-specific usage of intramyocellular lipids and glycogen during exercise. Med. Sci. Sports Exerc. 37, 1517–1524. doi: 10.1249/01.mss.0000177478.14500.7c
Keywords: sexual dimorphism, mitochondria, endurance performance, mitochondrial function, skeletal muscle, OXPHOS
Citation: Cardinale DA, Larsen FJ, Schiffer TA, Morales-Alamo D, Ekblom B, Calbet JAL, Holmberg H-C and Boushel R (2018) Superior Intrinsic Mitochondrial Respiration in Women Than in Men. Front. Physiol. 9:1133. doi: 10.3389/fphys.2018.01133
Received: 12 April 2018; Accepted: 30 July 2018;
Published: 17 August 2018.
Edited by:
François Billaut, Laval University, CanadaReviewed by:
Mary C. McKenna, University of Maryland, Baltimore, United StatesCéline Aguer, Institut du Savoir Montfort (ISM), Canada
Copyright © 2018 Cardinale, Larsen, Schiffer, Morales-Alamo, Ekblom, Calbet, Holmberg and Boushel. This is an open-access article distributed under the terms of the Creative Commons Attribution License (CC BY). The use, distribution or reproduction in other forums is permitted, provided the original author(s) and the copyright owner(s) are credited and that the original publication in this journal is cited, in accordance with accepted academic practice. No use, distribution or reproduction is permitted which does not comply with these terms.
*Correspondence: Robert Boushel, robert.boushel@ubc.ca
†These authors have contributed equally to this work