Assessment of global sorghum production, tolerance, and climate risk
- Ralph M. Parsons Laboratory, Department of Civil and Environmental Engineering (CEE), Massachusetts Institute of Technology, Cambridge, MA, United States
Some cereal crops can withstand harsh growing conditions, and, hence, can be considered an important line of defense against food shortage under climate change. Sorghum (Sorghum bicolor (L.) Monech) is the main food crop for millions of people, especially in sub-Saharan Africa and South Asia. This crop performs well under input constraints (e.g., limited water) and exhibits high resilience to adverse climate conditions (e.g., high temperature, drought, and waterlogging), making it a star crop for combating hunger under climate change. However, sorghum and other similar crops are not tolerant in absolute terms. Climate change could push the growing conditions of these crops beyond the limits they can tolerate, jeopardizing the food security of millions of people around the world. In this research, we analyzed the current status of sorghum production, trends, and factors controlling sorghum yield using empirical approaches. Especially in sub-Saharan Africa, this crop is cultivated widely under unfavorable climate conditions and sub-optimal input levels. Our findings raise multiple concerns as well as highlight opportunities for the sustainability of sorghum cultivation in a future with climate change. Sorghum yield seems to increase persistently despite global warming due to an improved inputs approach, offering hope that similar adaptation approaches can be fruitful, especially in sub-Saharan Africa. A combination of interventions including adequate use of fertilizers and technology adoption (e.g., tolerant cultivars), efficient management (e.g. improved irrigation), and better agronomic practices, is the key to boosting sorghum yield and ensuring the sustainability of this important crop under a warmer climate.
1. Sorghum, a “star” crop
Globally, sorghum (Sorghum bicolor (L.) Moench) is the fifth most important cereal crop (Iqbal et al., 2010; Huang, 2018; Hao et al., 2021; Ndlovu et al., 2021). It is a C4 carbon cycle plant with high photosynthetic efficiency and productivity (Tari et al., 2013). This crop originated and was domesticated in sub-Saharan Africa and spread to India and China (de Wet, 1978). In sub-Saharan Africa, several closely-related wild species of sorghum are found (Legwaila et al., 2003; Ananda et al., 2020). Recent studies reported that the earliest evidence of domestication can be found in eastern Sudan around the fourth Millennium BC (Winchell et al., 2017). This crop is widely cultivated in more than 100 countries around the world (Upadhyaya et al., 2019; Hao et al., 2021). Sorghum is well-known for its strong resistance and wide adaptability to multiple biotic and abiotic stresses (Machado and Paulsen, 2001; Tari et al., 2013; Huang, 2018; Zhang et al., 2019). The wide popularity of this crop is due to its (i) diverse end-uses as human food, livestock feed, biofuel, and forage, (ii) high returns, (iii) more resilience to adverse environmental conditions compared to many other cereal crops, and (iv) well-performance under conditions of water and temperature constraints, especially in marginal lands (Igartua et al., 1994; Machado and Paulsen, 2001; Tari et al., 2013; Monk et al., 2014; Huang, 2018; Zhang et al., 2019; Hao et al., 2021). These characteristics have attracted attention to sorghum as a potential “star” crop to tackle the challenge of the global food security (Tari et al., 2013; Kadam et al., 2017; Huang, 2018; Hao et al., 2021; Ndlovu et al., 2021).
2. Sustainability of sorghum cultivation: sources of concern
Although sorghum has a relatively higher tolerance to biotic and abiotic stresses compared to other crops, there are multiple sources of concern regarding the sustainability of its cultivation. Below is a brief discussion of these major concerns.
• First, sorghum is the main staple crop for more than 500 million people in more than 30 countries (Abreha et al., 2021), especially in sub-Saharan Africa (Prasad et al., 2021). Therefore, discrepancies in its production can potentially jeopardize the food security of millions of poor people.
• Second, there is a considerable reduction in the area under cultivation and a severe decline in crop yield in some of the major sorghum-producing countries. India and Sudan represent, on average, approximately 34% of the global harvested area and produce together nearly 17.6% of the total world sorghum’s production. Using the FAOSTAT dataset (FAO, 2022a), we estimated a large reduction in crop yield in Sudan. Over the last 60 years (1961–2020), the average yield of sorghum in Sudan has decreased by 55% (from 1.0 t/ha in 1961 to 0.4 t/ha in 2020). The rate of reduction is −4.7 kg/ha/year (Figure 1A). On the other hand, we estimated a drop of 70% (from 18.3 million t/ha to 5.5 million t/ha) in the harvested area of sorghum in India over 60 years (1961–2020). The reduction rate is estimated to be −0.25 million ha/year (Figure 1A). This large drop in the harvested area could be a result of large shifts to rice, wheat, and other commercial crops (Rao et al., 2010; Nagaraj et al., 2013).
• Third, compared to other cereal crops such as maize, rice, and wheat, sorghum yield is relatively low (Müller et al., 2020; Hao et al., 2021; Ndlovu et al., 2021). Refer to Figure 1C for a yield comparison. The average global yield of sorghum (~2.5 t/ha) is just at the lower level of the optimum yield, which ranges between 2.5 and 5 t/ha.
• Fourth, there is a serious imbalance in the global yield of sorghum. It is typically high in developed countries. Low yield persists in the countries of South Asia and sub-Saharan Africa, which are mostly low-income countries. In these countries, sorghum is used mainly as human food compared to its uses for livestock feed and biofuel production in developed countries. More information about this imbalance will follow in section (4.1).
• Fifth, although large progress has been made in sorghum breeding in the past decades, the rate of improvement in crop yield due to breeding programs is relatively slow. According to Pfeiffer et al. (2019), sorghum breeding programs in the United States and Argentina are progressing at an annual rate of nearly 0.01 t/ha compared to rates of approximately 0.09, 0.02, and 0.02 for corn, soybean, and cotton, respectively (Figure 1D).
• Sixth, there is an increasing trend in cultivating sorghum for biofuel production, generating competition with food production on limited water and land resources (Zhuang et al., 2011; Damerau et al., 2016). Some researchers argue that the rise in biofuel production is considered the main driver of the recent increase in food prices (Graham-Rowe, 2011; Shrestha et al., 2019), in particular during the period 2001–2008 (Hochman et al., 2012). The increase in the prices of food commodities can be devastating for poor people, especially in developing countries (Stein, 2007; Rosegrant and Msangi, 2014).
• Seventh, there is a great variation in yield and tolerance to biotic and abiotic stressors across genotypes and under different climate conditions (Pfeiffer et al., 2019).
• Finally, there are multiple biotic (e.g., pests, diseases, birds, and parasitic weeds) and abiotic (e.g., heat stress, soil moisture deficit, and waterlogging) that affect the health of the sorghum plant, and, in turn, might reduce its productivity (Perumal et al., 2018; Abreha et al., 2021; Fakrudin et al., 2021) or even result in crop failure in extreme cases.
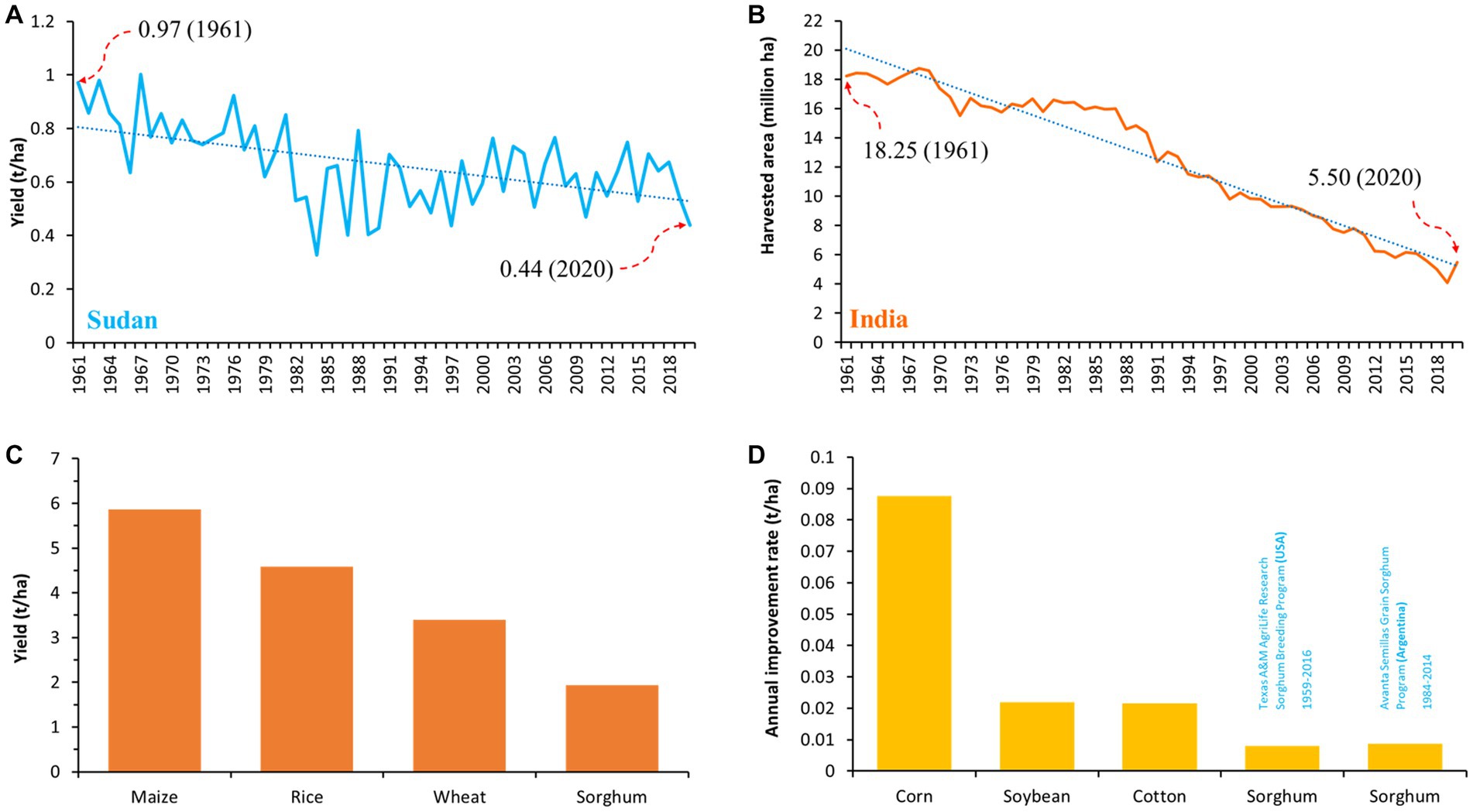
Figure 1. Some sources of concern regarding the sustainability of sorghum cultivation. Trends in sorghum for the period 1961–2020 in two major sorghum-producing countries: Severe drop in yield in Sudan (A) and substantial reduction in harvested area in India (B). Compared to other cereal crops, sorghum exhibits a relatively lower global average yield in terms of production (tonnes, t) per area (hectare, ha), and genetic yield gain in sorghum is significantly slower than in other major cereal crops (D). Source of data used to produce these graphs: (a and b) from the FAOSTAT database (FAO, 2022a), (C) constructed based on data from Hao et al. (2021) and Müller et al. (2020), and (D) visualized based on information from Pfeiffer et al. (2019).
A critical assessment of the production of sorghum on a global scale, its tolerance and vulnerability to climate risk is, therefore, crucial, but not available. Against this background, the current research aims at providing a comprehensive evaluation of the current status and historical development of sorghum production, abiotic stresses, and its tolerance mechanisms. In addition, it studies the vulnerability of sorghum to climate risk. This assessment will help identify challenges and opportunities for ensuring the sustainability of cultivating this important food crop and identify entry points that are helpful to ensure the sustainability of sorghum cultivation under the threat of climate change. This research includes an analysis of datasets from more than 100 countries where sorghum is cultivated, supported by a thorough literature review.
3. Materials and methods
3.1. Sorghum data
FAOSTAT database is developed and maintained by the United Nations Food Agriculture Organization (FAO). This database provides one of the most comprehensive datasets for crop production and other types of data like data on import and export of agricultural commodities on a country level. In the current study, we used FAOSTAT data concerning sorghum harvested area in hectares (ha), yield in tonnes per hectare (t/ha), and production in tonnes (t). These time series were downloaded from the FAOSTAT dissemination website (Supplementary Table S1). In addition, the time series of the use rates of fertilizers in kilograms per hectare (kg/ha) in these countries were also retrieved from the same database. These data include three main types of fertilizers, namely, nutrient nitrogen (N), nutrient phosphate (P2O5), and nutrient potash (K2O). For all data listed above, the downloaded data cover the period between 1961 and 2020. For some analyses (e.g., trends of yield, harvested area, and production), we used the multi-year (1960–2020) country average values of these parameters. We confined our analysis to the last 20 years (2001–2020) for other assessments (e.g., yield gap and climate risk assessments), as some countries show considerable changes in some of these variables in the last two decades compared to their magnitudes in the 1960s. The percentage of irrigated areas in each country to all cultivated areas was collected from the AQUASTAT database (FAO, 2022b).
As sorghum seasons vary among countries, we used three main sources to derive sorghum calendars (approximate sowing and harvesting dates) for the main cultivation season in each country. These sources are the International Production Assessment Division (IPAD) of the United States Department of Agriculture (USDA) and the Global Information and Early Warning System (GIEWS) maintained by the FAO. When absent from these data sources, sorghum calendars were collected from the available literature or country-specific and regional databases.
To confine gridded climate data geographically to only areas where sorghum is cultivated, we used the global crop distribution layer of sorghum provided by the International Food Policy Research Institute (IFPRI). This dataset is constructed using the IFPRI’s Spatial Production Allocation Model (SPAM) to provide global distribution of crop-harvested areas at a grid resolution (IFPRI, 2019).
3.1.1. Climate data
For climate, we utilized gridded data on rainfall and temperature from the Climatic Research Unit Gridded Time Series (CRU TS). CRU TS provides a long-time series of climate variables covering the period from 1901 to the present. This data comes in a NetCDF format with a spatial resolution of 0.5o × 0.5o. For the current research, we used monthly data from version 4.05 for the period 1961–2020 to overlap with FAOSTAT data on sorghum. Using CRU TS v. 4.05 dataset, we generated seasonal time series of rainfall and temperature for each country based on its sorghum calendar. Rainfall rates and temperature averages were aggregated using functions of the Climate Data Operator (CDO; Schulzweida, 2022) and clipped to the sorghum cultivation area in each country using the Extract by Mask function in the ArcGIS 10.3.1 (ESRI, 2014).
3.2. Methods
The current investigation involves four components (Figure 2): (i) evaluation of sorghum production status, historical development, and trends, (ii) estimation of yield gap in sub-Saharan Africa, (iii) assessment of soil nutrient replenishment, and (iv) analysis of sorghum vulnerability to climate risk. A brief description of these four components is provided in the following sub-sections.
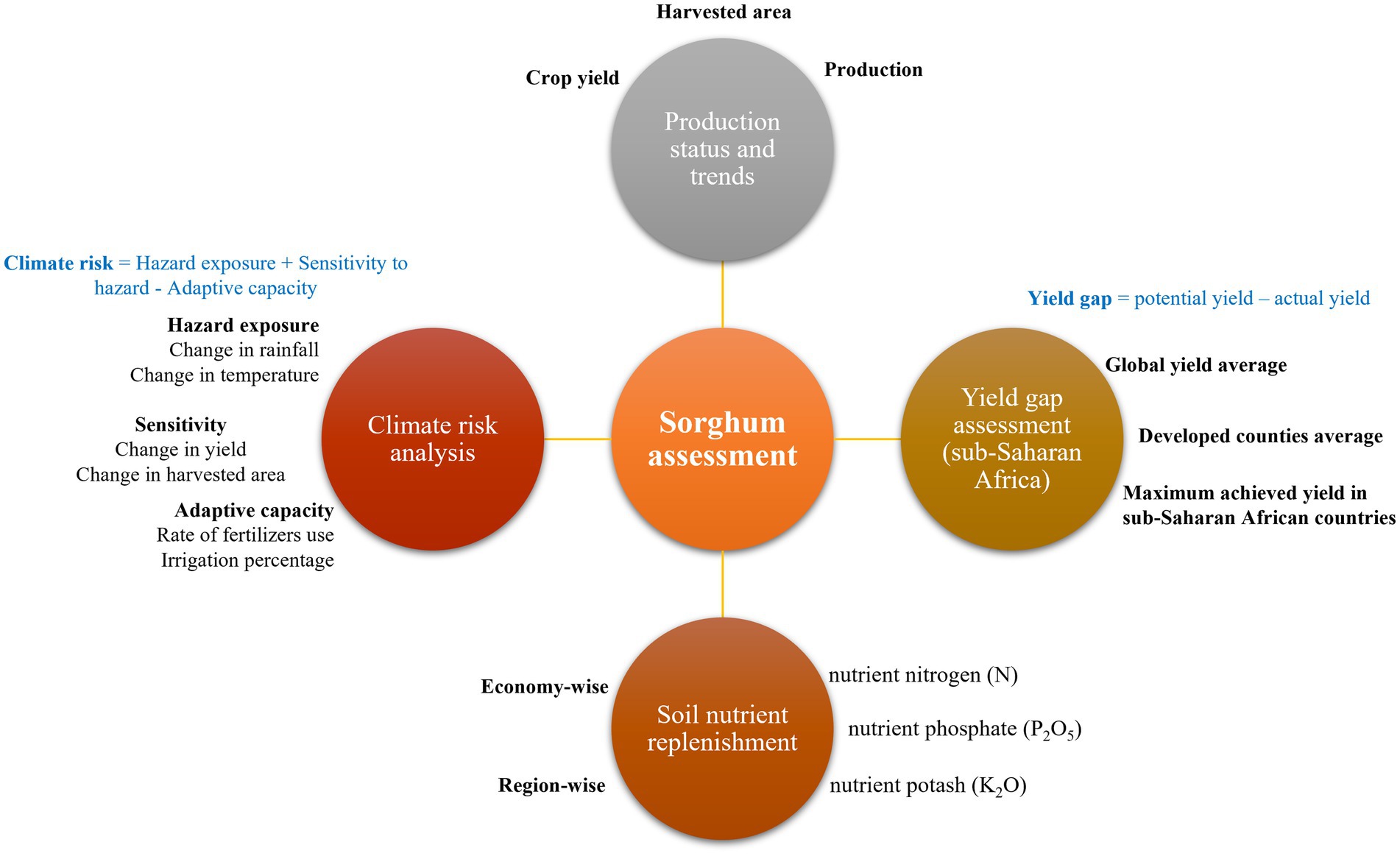
Figure 2. Four components adopted in the current study along with input data used in the involved assessments.
3.2.1. Evaluation of the global status of sorghum production
In this part, we assessed three variables regarding sorghum in 102 countries for the last two decades, i.e., 2001–2020. These variables were crop yield, cultivated area, and production. The analysis was conducted for (i) individual countries as well as for aggregated estimates on (ii) region-wise and (iii) economy-wise. For the region-wise and country-wise assessments, we adopted the country classification of the World Bank. This classification groups countries into seven regions and four economic (income level) groups. The seven regions are North America, Middle East & North Africa, Europe & Central Asia, Latin America & Caribbean, East Asia & Pacific, South Asia, and sub-Saharan Africa. The four economy groups are high-income, upper-middle-income, lower middle income and low-income. A detailed list of countries with their groups can be found in the Supporting Information (Supplementary Table S1).
3.2.2. Yield gap assessment in sub-Saharan Africa
As mentioned earlier, sub-Saharan Africa exhibits generally low magnitudes of sorghum yield compared to other sorghum-producing countries despite the importance of sorghum for food security in this region. This analysis focused on 31 sub-Saharan African countries in which sorghum is cultivated. The analysis aimed to estimate the magnitude of the yield gap of sorghum, highlighting the hidden potentials for increasing sorghum production in these countries. The yield gap is defined as the difference between potential yield and actual yield (Equation 1).
For this purpose, we followed three approaches to calculate the yield gap for the period 2001–2020. Three proxies for potential yield were considered: (1) the maximum achieved yield in each country’s time series (for Yield gap 1), (2) the global average (for Yield gap 2) and developed countries’ average (for Yield gap 3).
3.2.3. Soil nutrient replenishment
We analyzed the use rates of three types of fertilizers, namely, nutrient nitrogen (N), nutrient phosphate (P2O5), and nutrient potash (K2O) in all considered countries. This assessment was conducted also in country-wise and economy-wise approaches. The average use levels of these three types of fertilizers were correlated with the corresponding average yield magnitudes. This analysis can reflect and provide insights into the impact of fertilizer use on sorghum yield.
3.2.4. Climate risk analysis
In this part, we correlated two climate variables, i.e., average seasonal rainfall and temperature, with the sorghum yield in each country to infer insights on how sorghum yield behaves under different climate conditions. Plotting countries based on their average seasonal rainfall and temperature was carried out to identify countries where sorghum production is cultivated under unfavorable climate conditions. The unfavorable conditions adopted herein are those with an average temperature of more than 30°C, and less than 600 m of average rainfall per season. These limits were identified from our climate-yield relationships established in the current study, which correlate well with the critical temperature and rainfall zones under which sorghum growth thrives (Tack et al., 2017; Prasad et al., 2019; Elramlawi et al., 2020; Krishna, 2021). Investigating sorghum in countries where sorghum is cultivated under unfavorable conditions, especially if they show different yield levels, potentially provides transferrable insights on how to increase sorghum yield under these climate conditions. Data of the identified countries where sorghum is growing under climate risk went through a climate risk assessment using three risk components, i.e., exposure to hazards, the sensitivity of systems, and adaptive capacity using Equation (2). This three-dimensional framework is widely accepted and applied to assess climate risk (Jurgilevich et al., 2017; Sharma and Ravindranath, 2019; Fernandes et al., 2022).
For exposure, we used the change in rainfall and temperature. These two climate variables are widely controlling crop production (Lobell and Burke, 2008; Khan et al., 2022). For sensitivity, we used the change in yield and harvested area. According to literature, the response of agricultural systems to climate hazards is usually translated to changes in yield (Moore et al., 2017; Raza et al., 2019; Liu et al., 2021) and/or agricultural land use pattern (Iizumi and Ramankutty, 2015; Arora et al., 2020; Nainggolan et al., 2023). As for adaptive capacity, we used the change in fertilizer use rate and the percentage of irrigated cropland (% irrigation) in the country. The change in time series was calculated as a slope of the corresponding data for the period 1961–2020, except for %irrigation, in which we adopted the most recent figure for each country as reported by FAO (2022b). Fertilizers and irrigation (as will be shown later) are adding positively to the adaptive capacity of agricultural systems. Although other variables can be used in the assessment of climate risk on crop production, we think using other variables will not result in a significant deviation from the results obtained by using these two variables. This is mainly because many other variables (e.g., use of pesticides and adoption of improved seeds) display global patterns that are similar to those shown by fertilizer use and irrigation. In conclusion, we believe that the selected variables largely represent the three elements of climate risk assessment and are adequate for the current climate risk analysis.
4. Results
4.1. Current status and historical development of global sorghum production
Sorghum is cultivated widely around the globe (Figure 3). The multi-year average harvested area globally in the last two decades (2001–2020) is 40.9 million ha. Around 80% of the global sorghum area of sorghum is harvested in only 10 countries. The top 10 countries in harvested areas (arranged from the largest to smallest) are India (7.3 million ha), Sudan (6.8 million ha), Nigeria (6.1 million ha), Niger (3.1 million ha), the United States (2.5 million ha), Mexico (1.8 million ha), Burkina Faso (1.7 million ha), Ethiopia (1.6 million ha), Mali (1.2 million ha), and Brazil (0.7 million ha).
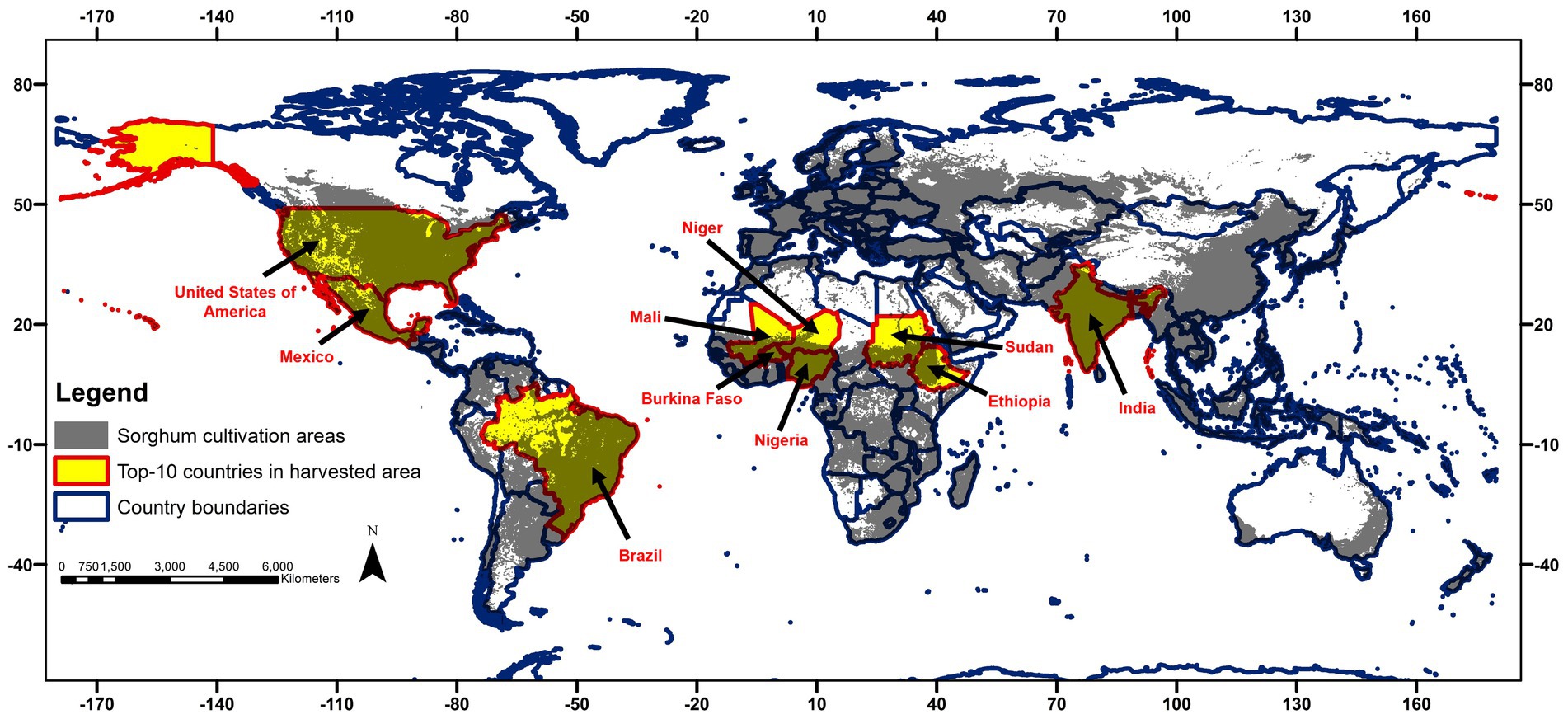
Figure 3. Map of the global distribution of sorghum cultivation areas. Sorghum distribution in this map is derived from the layer produced by the Spatial Production Allocation Model (SPAM) and distributed by IFPRI (2019). The top 10 countries with the largest harvested areas of sorghum are highlighted in yellow. Political boundaries on this map were adopted from the Database of Global Administrative Areas, GADM (https://gadm.org/index.html).
The multi-year (2001–2020) average production of sorghum is nearly 58.7 million t per year. The top 10 sorghum-producing countries (arranged from the largest to lowest) are the United States (10.1 million t), Nigeria (7.3 million t), India (6.2 million t), Mexico (5.9 million t), Sudan (4.2 million t), Ethiopia (3.4 million t), Argentina (2.8 million t), China (2.6 million t), Brazil (1.9 million t), Australia (1.8 million t). The total production of these 10 countries represents around 78.6% (46.1 million t) of the total global production of sorghum.
Using the sorghum yield data of all countries where the crop is cultivated, the global average (2001–2020) yield is 2.5 t/ha. Sorghum yield shows high variation across regions and income country groups. While the average yield of sorghum is larger than or comparable to this global average in North America (4.1 t/ha), the Middle East and North Africa (5.7 t/ha), Europe and Central Asia (3.1 t/ha), Latin America and the Caribbean (2.6 t/ha), and East Asia and Pacific (2.5 t/ha), sorghum yield in South Asia (0.9 t/ha) and sub-Saharan Africa (0.9 t/ha) is far below this global average (Figure 4A). Aggregating the data of all countries according to their income levels indicates that compared to high income and upper-middle-income countries, lower middle income and low-income countries exhibit lower yield (Figure 4B). Countries of low-income levels are predominantly located in South Asia and sub-Saharan Africa. The difference in yield between the top 10 countries with the highest and lowest yield is quite large (Figure 4C). This difference in yield plays a key role in limiting the global production of sorghum. While seven out of the top 10 countries in sorghum harvested areas are located in South Asia (India) and sub-Saharan Africa (Niger, Sudan, Mali, Burkina Faso, Nigeria, and Ethiopia), their low yield compared to the other top sorghum producers, i.e., the United States, Mexico, and Brazil (Figure 4D), largely inhibiting boosting the total global production.
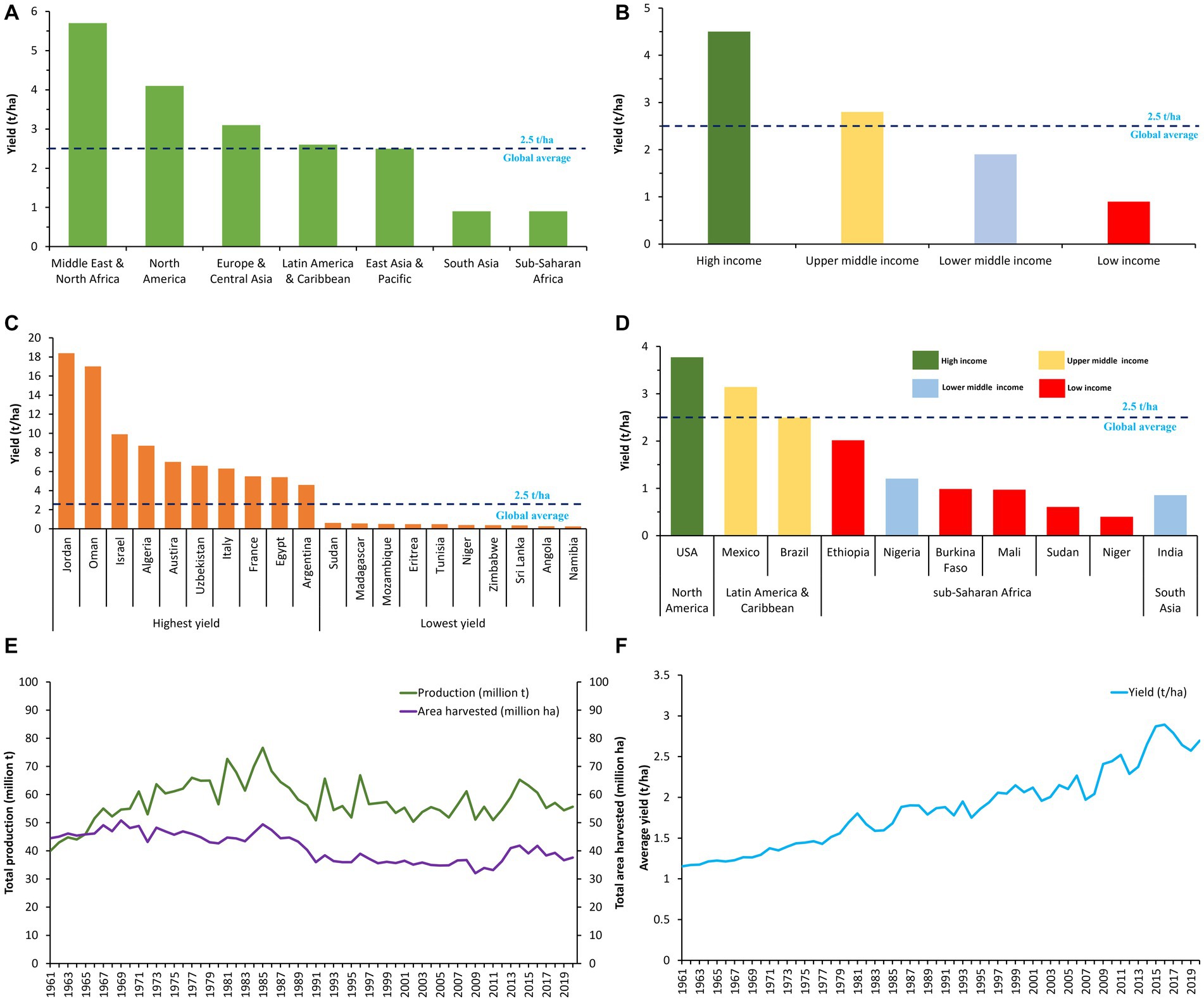
Figure 4. Multi-year (2001–2020) average yield of sorghum: region-wise (A); economy-wise (B); 10 countries with the highest and lowest yield (C); top 10 countries with sorghum harvested area (D); time series of global sorghum production (in million tonnes) and area harvested (in million ha) for 1961-2020 (E); and time series of average yield on a global scale for 1961-2020 (F). Surce of data: FAOSTAT database (FAO, 2022a).
Comparing the historical development of sorghum cultivation globally for three periods (1961–1980, 1981–2000, and 2001–2020) indicates that the noticeable increase in the total global production is mainly attributed to the noticeable increase in yield rather than in harvested area See Figures 4E,F. While the global average yield of sorghum increased from 1.4 t/ha (1961–1980) to 1.8 t/ha (1981–2000) and 2.5 (2001–2020), harvested area exhibited a remarkable decrease from 46.3 million ha to 43.2 million ha and 40.9 million ha for the three periods, respectively.
4.2. Yield gap in sub-Saharan Africa
In sub-Saharan Africa, sorghum is cultivated in 31 countries with a total harvested area of around 24.5 million ha, as an average for 2001–2020. More than 70% of this area (~17.3 million ha) is located in Nigeria, Sudan, Niger, Ethiopia, Mali, and Burkina Faso (Figure 5A). The multi-year average sorghum yield for the 31 sub-Saharan Africa is estimated to be nearly 0.9 t/ha. Our analysis shows differences in sorghum yield in sub-Saharan Africa (Figure 5B). The highest average yield of sorghum among these countries can be found in South Africa and Ethiopia, with an average yield of 2.8 and 2.0 t/ha, respectively. Countries such as Benin, Cameroon, Gambia, Ghana, Guinea, Nigeria, Rwanda, Tanzania, and Uganda show average yields well above 1.0 t/ha. On the other hand, the lowest sorghum yield in this region can be found in Namibia, Angola, Sri Lanka, Zimbabwe, Niger, Tunisia, and Eritrea, with yield magnitudes below 0.5 t/ha. The maximum achieved yield follows the same patterns, emphasizing the prevailing local conditions that inhibit sorghum yield in these countries. The multi-year average of the maximum achieved yield of sub-Saharan Africa is 1.3 t/ha (Figure 5B). The highest maximum yield over the 20 years (2001–2020) was achieved in South Africa (~4.0 t/ha).
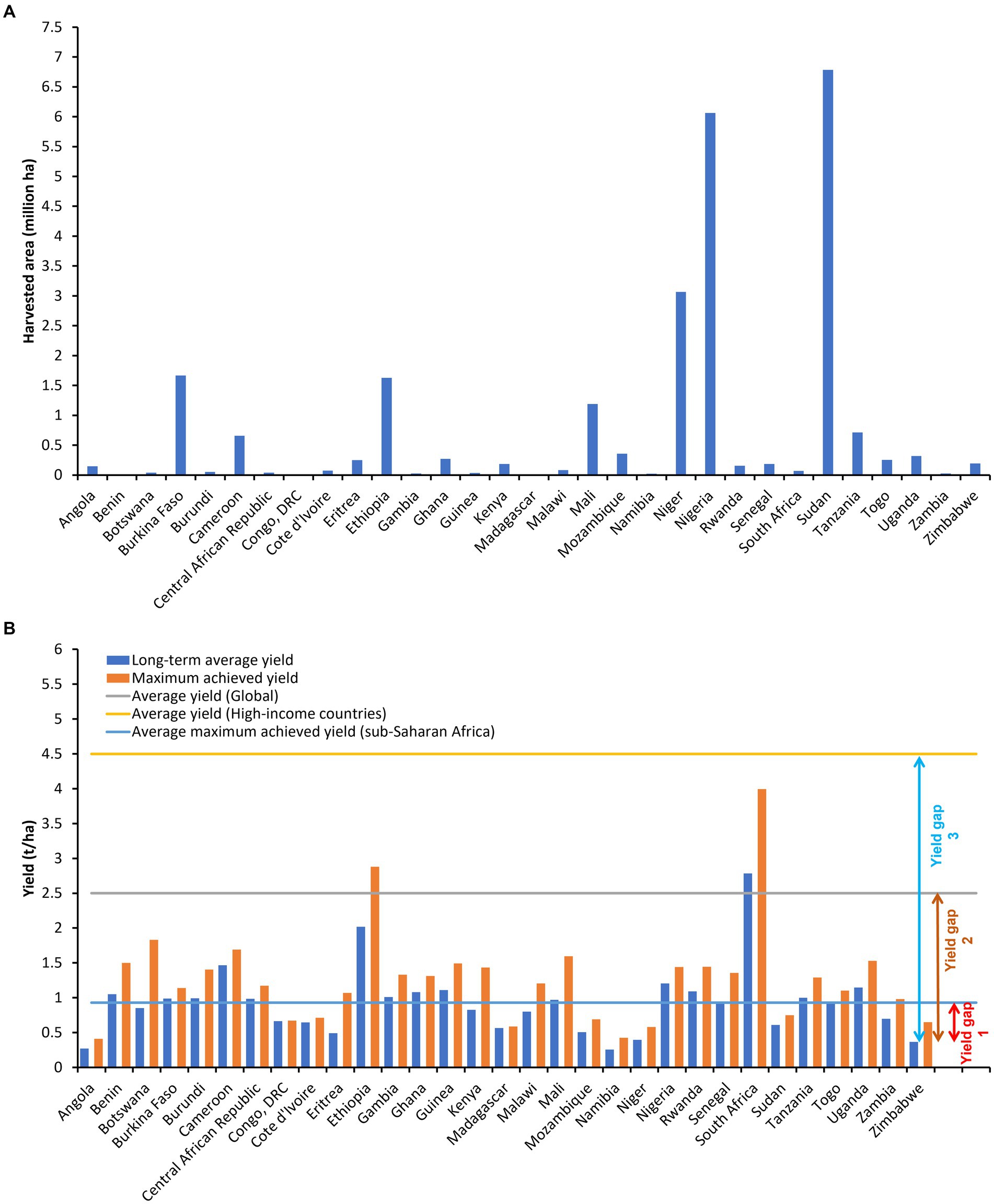
Figure 5. Status of sorghum production in sub-Saharan African countries for the period 2001–2020: multi-year average harvested area (A), multi-year yield average with three yield gaps based on: maximum achieved yield (yield gap 1), global average (yield gap 2), and high-income countries (yield gap 3) (B). Data used in the current analysis are those obtained from the FAOSTAT database (FAO, 2022a).
Our results indicate massive yield gaps (the difference between actual and potential yield) in sorghum cultivated in sub-Saharan Africa (Figure 5B). The average calculated yield gap is nearly 0.4 t/ha, when the maximum achieved yield in considered (Yield gap 1 in Figure 5B). The largest yield gap is noticed in South Africa, Botswana, and Ethiopia, with average yield gaps, equal to 1.2, 1.0, and 0.9 t/ha, respectively. Calculating the yield gap based on the global yield average (2.5 t/ha) and the high-income countries’ average (4.5 t/ha) reveals a much higher regional yield gap in sub-Saharan Africa (around 1.0 and 3.5 t/ha, respectively). These two yield gaps are shown in Figure 5B as yield 2 and 3, respectively.
4.3. Deficit in soil nutrient replenishment
In the current investigation, we noticed a large variation in levels of fertilizer use rates in the studied countries. Using FAOSTAT data on sorghum yield and levels of fertilizer use per cropland area, high-income countries exhibit considerably higher rates of fertilizer used per cropland area compared to low-income countries. The average use rate of nutrient nitrogen (N) per cropland was calculated as follows: East Asia and Pacific = 73.7 kg/ha, Europe and Central Asia = 57.2 kg/ha, Latin America and Caribbeans = 53.5 kg/ha, Middle East and North Africa = 52.2 kg/ha, North America = 54.1 kg/ha, South Asia = 51.6 kg/ha, and sub-Saharan Africa = 5.5 kg/ha (Figure 6A). The difference in use rate of N fertilizers between the high-income and low income countries is remarkable, i.e., 84.7 versus 4.3 kg/ha, respectively (Figure 6B). The low-income countries are mostly located in South Asia and sub-Saharan Africa. Correlating average sorghum yield with the average levels of fertilizer use revealed a positive linear relationship. This is true for all three main fertilizer types based on both regional and economic classifications of countries. Refer to Figures 6C,D for nitrogen (N), Figures 6E,F for phosphate (P2O5), and Figures 6G,H for potash (K2O).
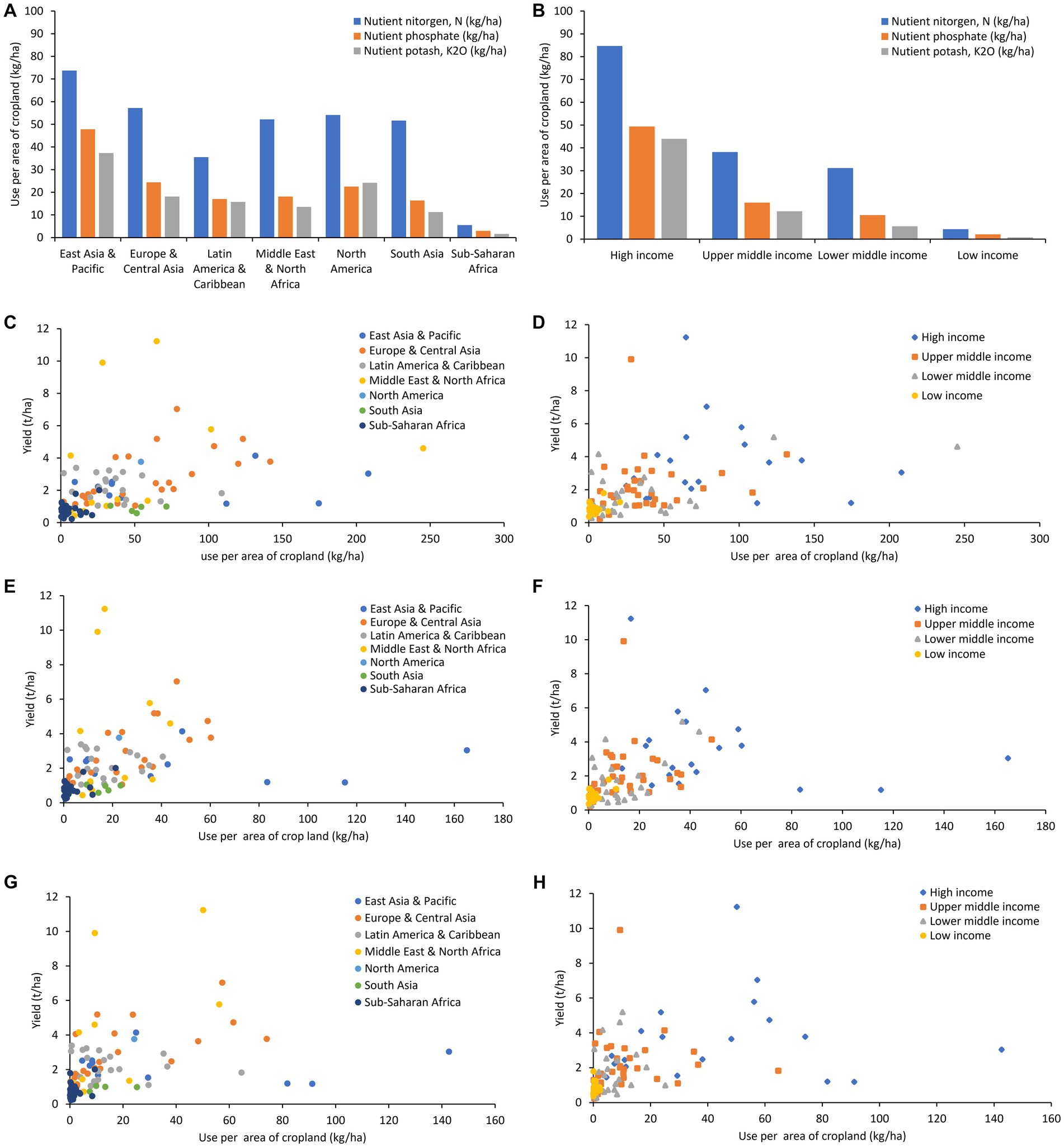
Figure 6. Relationship between the use rates of three main types of fertilizers and sorghum yield as averages over the period on a region-wise (left panel) and economy-wise (right panel aggregation): average use of fertilizer types per cropland area (A,B); use of nutrient nitrogen (N) and yield (C,D); nutrient phosphate (P2O5) and yield (E,F); nutrient potash (K2O) and yield (G,H). Countries in sub-Saharan Africa and South Asia, which are largely low-income countries, exhibit the lowest levels of fertilizer application and sorghum yield.
4.4. Climate risk
Spatially, there is a large variation in climate regimes under which sorghum is cultivated globally. Generally, the impact of rainfall is positive. However, at some point (nearly above 800 mm), yield seems to be flattened and does not show an increase in response to enhanced rainfall (Figure 7A). On the other hand, high temperatures (~ higher than 30°C) are limiting sorghum yield (Figure 7B). The highest yield is observed in areas where temperature and rainfall are optimum. More specifically, where the seasonal temperature is between 18 and 26°C and seasonal rainfall is around 600 mm. In many countries, sorghum is cultivated under unfavorable conditions (Figure 8A). For plots of individual countries in each region, the reader is referred to the supporting information file (Supplementary Figure S1).
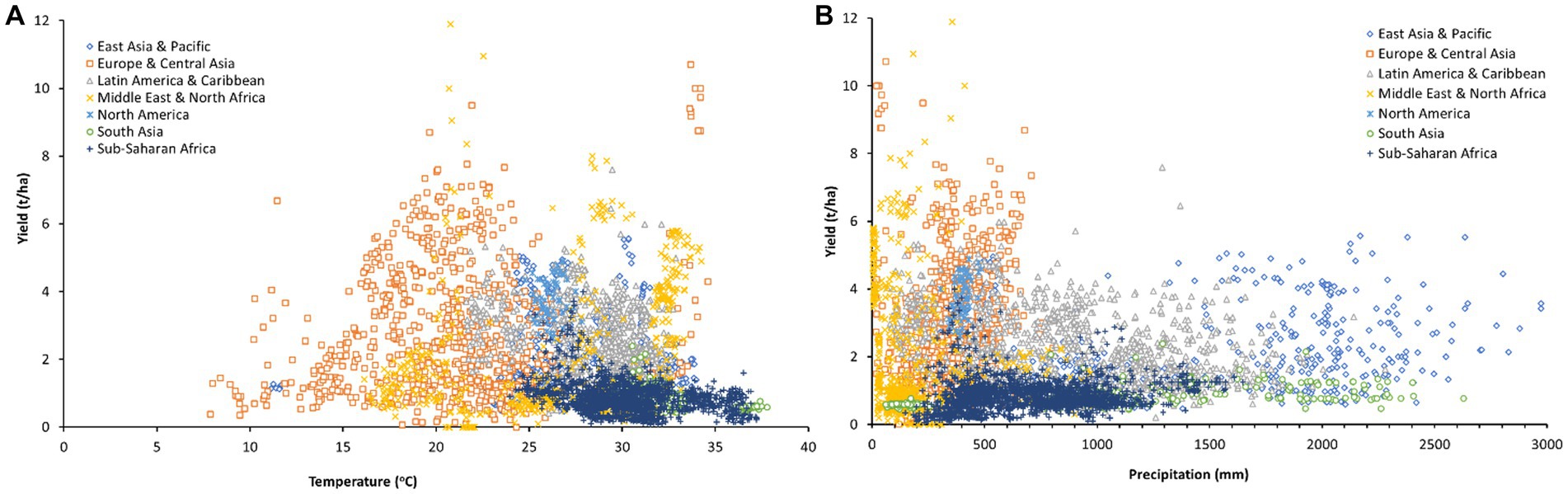
Figure 7. Plots of climate variables versus sorghum yield: seasonal maximum daily temperature (A) and seasonal rainfall (B). Every point plotted in these graphs represents one year of data in a specific country.
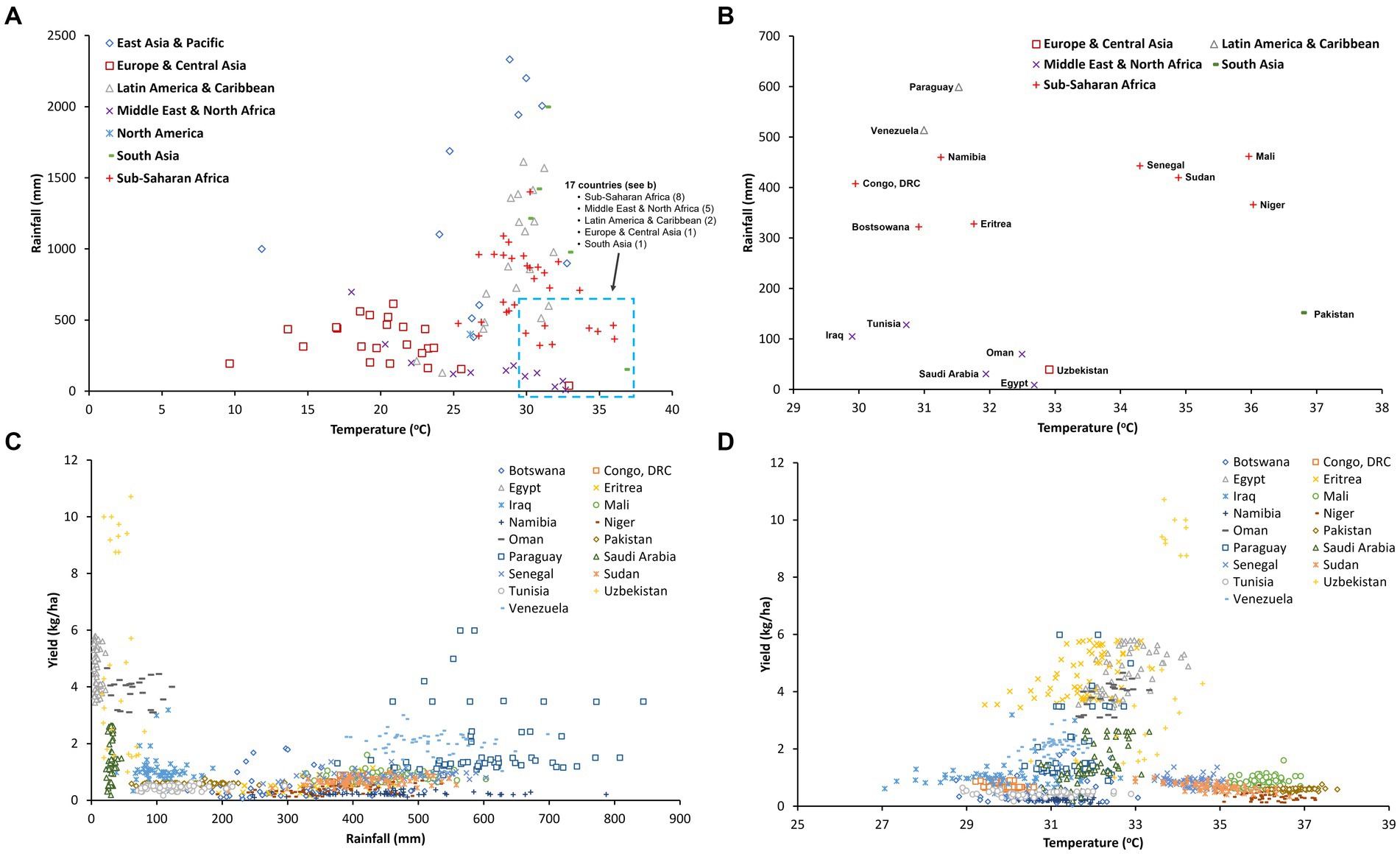
Figure 8. Identifying countries where sorghum is cultivated under unfavorable climate conditions: region-wise distribution of all sorghum cultivating countries based on the prevailing rainfall and temperature (A); 17 countries with sorghum cultivated under unfavorable climate conditions (B); precipitation versus yield in these 17 countries (C); and temperature versus yield in the same 17 countries (D). Data used for this analysis were derived from FAOSTAT (yield), and CRU TS (rainfall and temperature).
Using the data on temperature and precipitation, we identified 17 countries that are cultivating sorghum under unfavorable climate conditions (Figures 8A,B). These countries are Botswana, Congo DRC, Egypt, Eritrea, Iraq, Mali, Namibia, Niger, Oman, Pakistan, Paraguay, Saudi Arabia, Senegal, Sudan, Tunisia, Uzbekistan, and Venezuela. Eight out of these 17 countries are located in sub-Saharan Africa. Pakistan, Senegal, Sudan, Mali, and Niger are the most vulnerable to the risk of high temperatures among these countries. Especially in countries where sorghum is cultivated under a rainfed system, sorghum seems to be highly controlled by these climate conditions. Most of these 17 countries display low sorghum yield and these countries display noticeable variations in total sorghum production (Table 1). However, there are many exceptions to this observation. For instance, sorghum yield in many countries such as Egypt, Uzbekistan, Paraguay, Saudi Arabia, and Oman exhibit higher magnitudes than in other countries despite being located in hot temperature conditions and receiving negligible amounts of rain.
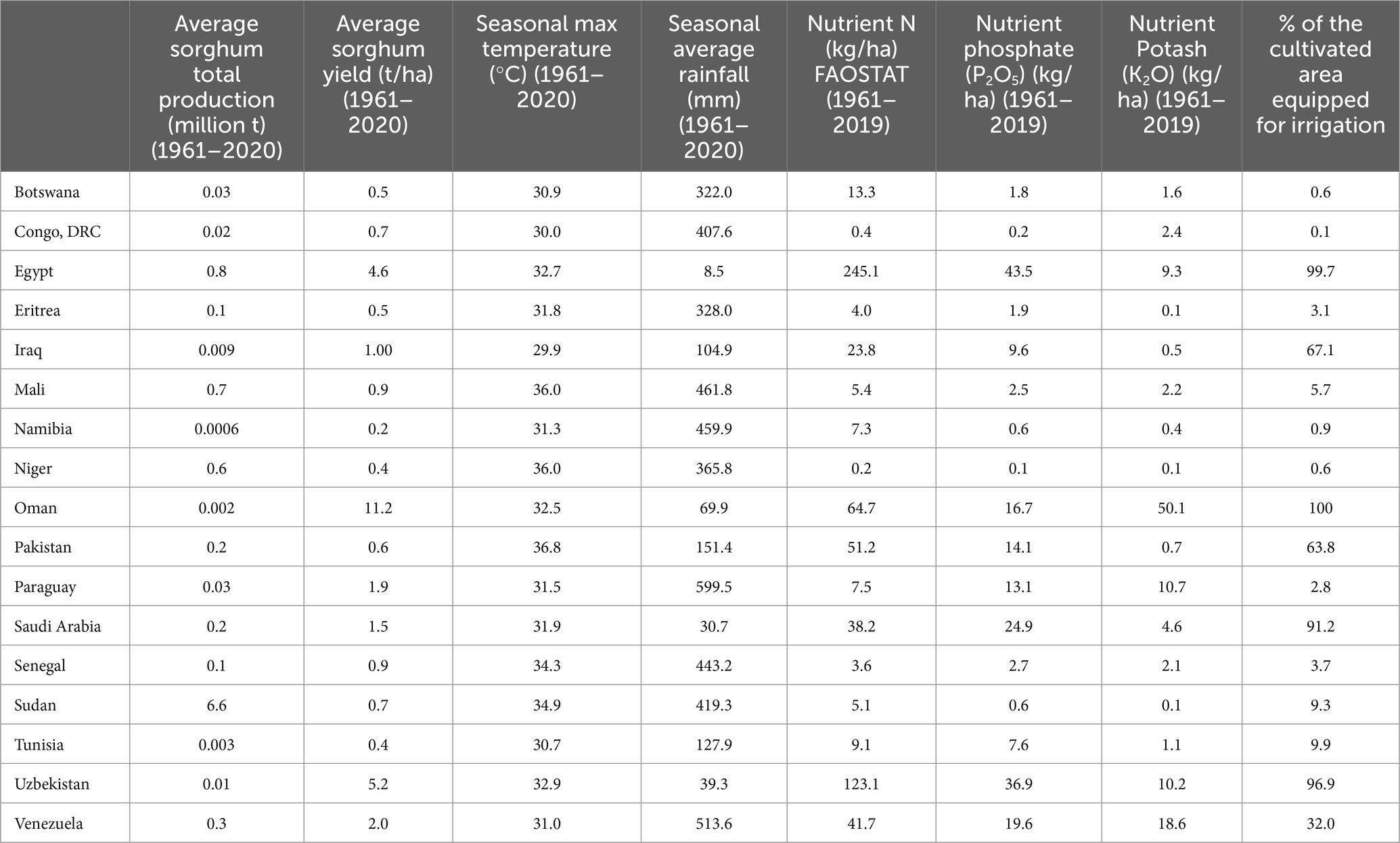
Table 1. Values of variables used for climate risk assessment in the current study for 17 countries identified as cultivating sorghum under unfavorable climate conditions (seasonal rainfall < 600 mm and average temperature > 30°C).
Our analysis of the rate use of fertilizers in these 17 countries shows that the use of fertilizer has increased dramatically in many of these countries (Supplementary Figure S2). The multi-year (1961–2019) average use per hectare of Nitrogen (N), Phosphate (P2O5), and Potash (K2O) are, respectively, 41.03 kg/ha, 12.4 kg/ha, and 7.5 kg/ha (Table 1). Egypt shows an outstanding levels of fertilizer use. Oman, Pakistan, Uzbekistan, and Venezuela also show levels of fertilizer use per hectare above the calculated average of the 17 countries, especially for nitrogen (N). Compared to these countries, sub-Saharan African countries use very little fertilizers. For instance, the long-term average use of N in Eritrea, Mali, Namibia, Niger, Senegal, and Sudan does not exceed 8 kg/ha (Table 1). See Supplementary Figure S3 for a visualized representation of fertilizer use, %irrigation, and yield in these 17 countries.
Ranking these countries according to their position in the three climate risk indicators, i.e., exposure, sensitivity, and adaptive capacity, indicates that Paraguay, Sudan, Venezuela, Niger, Pakistan, and Niger are the most highly exposed countries to climate hazards in sorghum cultivation (Figure 9). Sorghum in Oman, Uzbekistan, and Sudan is highly sensitive to climate hazard. Oman and Egypt have relatively the highest adaptive capacity among the 17 countries, reflecting their wide adoption of irrigation and high levels of fertilizers application. Based on these three risk elements, i.e., exposure, sensitivity, and adaptive capacity, sorghum cultivation is exposed to higher climate risk in Paraguay, Niger, Sudan, Mali, and Eritrea. This reflects their high exposure and sensitivity to climate hazard combined with low-medium levels of adaptive capacity.
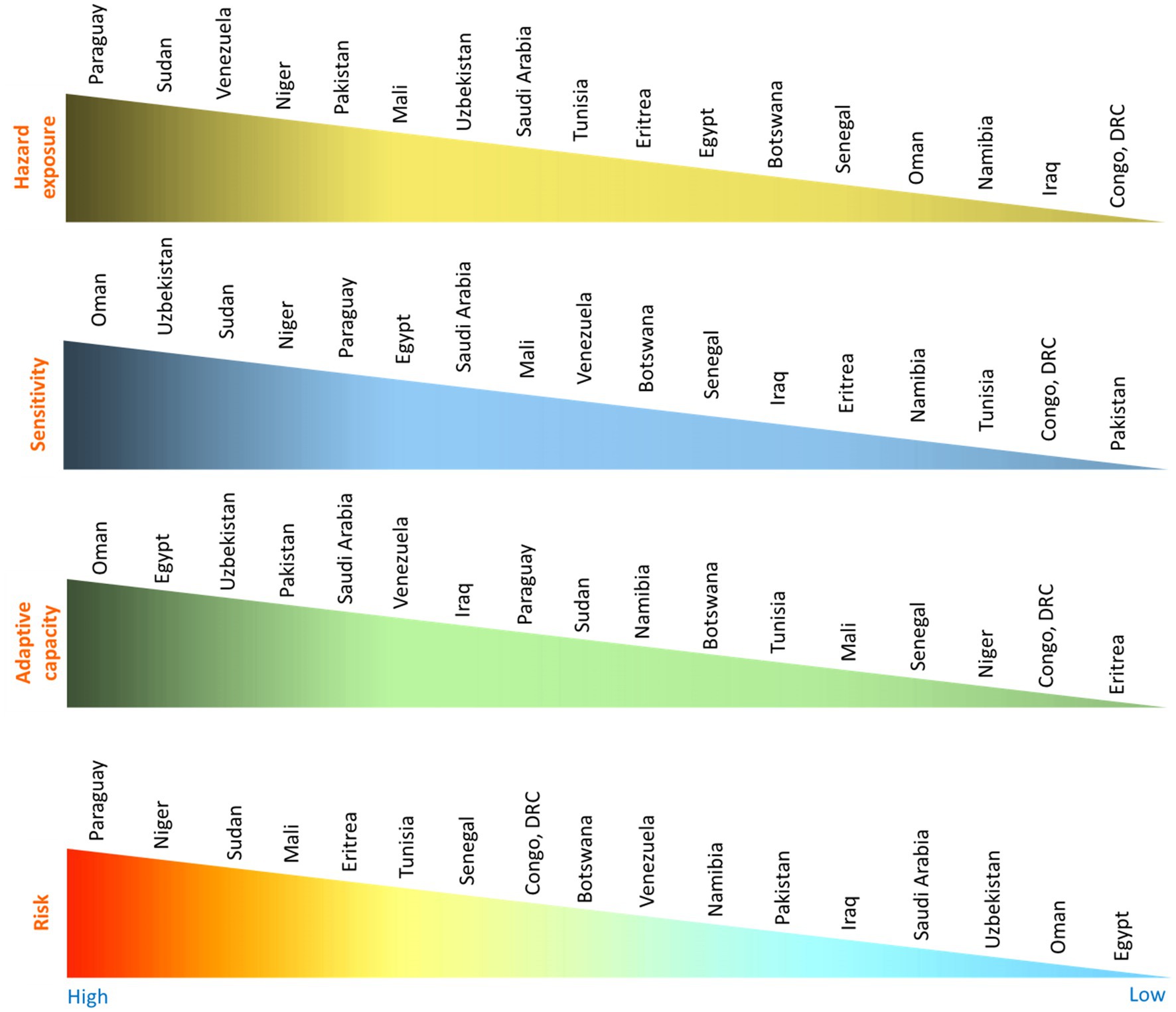
Figure 9. Ranking of the identified 17 countries where sorghum cultivation is under climate risk. Climate risk assessment includes three components: exposure, sensitivity, and adaptive capacity. Note: This ranking is only a relative comparison between the countries for visualization and has no absolute terms.
5. Discussion
5.1. Potentials for more sorghum production
The low yield in many countries, especially in sub-Saharan Africa (as shown in Section 4.2) represents a serious challenge not only for food security but also for natural resources (e.g., water and land). However, if addressed properly, it offers an opportunity to attain higher production from the same cultivated area (Khalifa et al., 2020). Our calculations indicate that bridging the yield gap to the average maximum yield achieved in the 31 countries in sub-Saharan Africa (1.3t/ha) has the potential to increase sorghum production in sub-Saharan Africa by 29.5% from the current production level, i.e., from 22.7 to 29.4 million t/ha (Table 2). More than 62% of this potential increase comes from Nigeria, Sudan, and Ethiopia alone. It should be noted that the yield gap estimated using the maximum achieved yield (2001–2020) in each country is a conservative estimate. Taking into consideration that sorghum potential yield in each country can be well higher than these figures, the current global average yield, and that of high-income countries, the potential production (by bridging yield gaps 2, and 3) must be much higher than the potential reported above (Table 2). However, using the approach of maximum achieved yield provides a modest and more realistic approximation of potential yield, assuming that this yield level can be achieved again (Ayyad and Khalifa, 2021).

Table 2. Comparison of the potential increase in sorghum production in sub-Saharan Africa by bridging the yield gaps calculated based on three proxies for potential yield: (i) the maximum achieved yield in each sub-Saharan Africa, (ii) the global yield average, and (iii) the average yield of the high-income countries.
5.2. Major abiotic stresses and tolerance mechanisms
As stated earlier, sorghum can withstand abiotic stresses of extreme climate conditions such as high temperature, moisture deficit, and waterlogging to a certain level. When these stresses exceed specific levels, they can impact the plants severely. For instance, an increase in temperature is likely to have positive impacts in cold regions and negative ones in regions where the temperature optimum window is crossed (Peñuelas et al., 2007). In this section, we discuss the impacts of three main abiotic stressors (Figure 10), namely, (i) heat stress, (ii) drought and moisture deficit, and (iii) excessive rainfall and waterlogging. on sorghum, and the crop tolerance to these stressors.
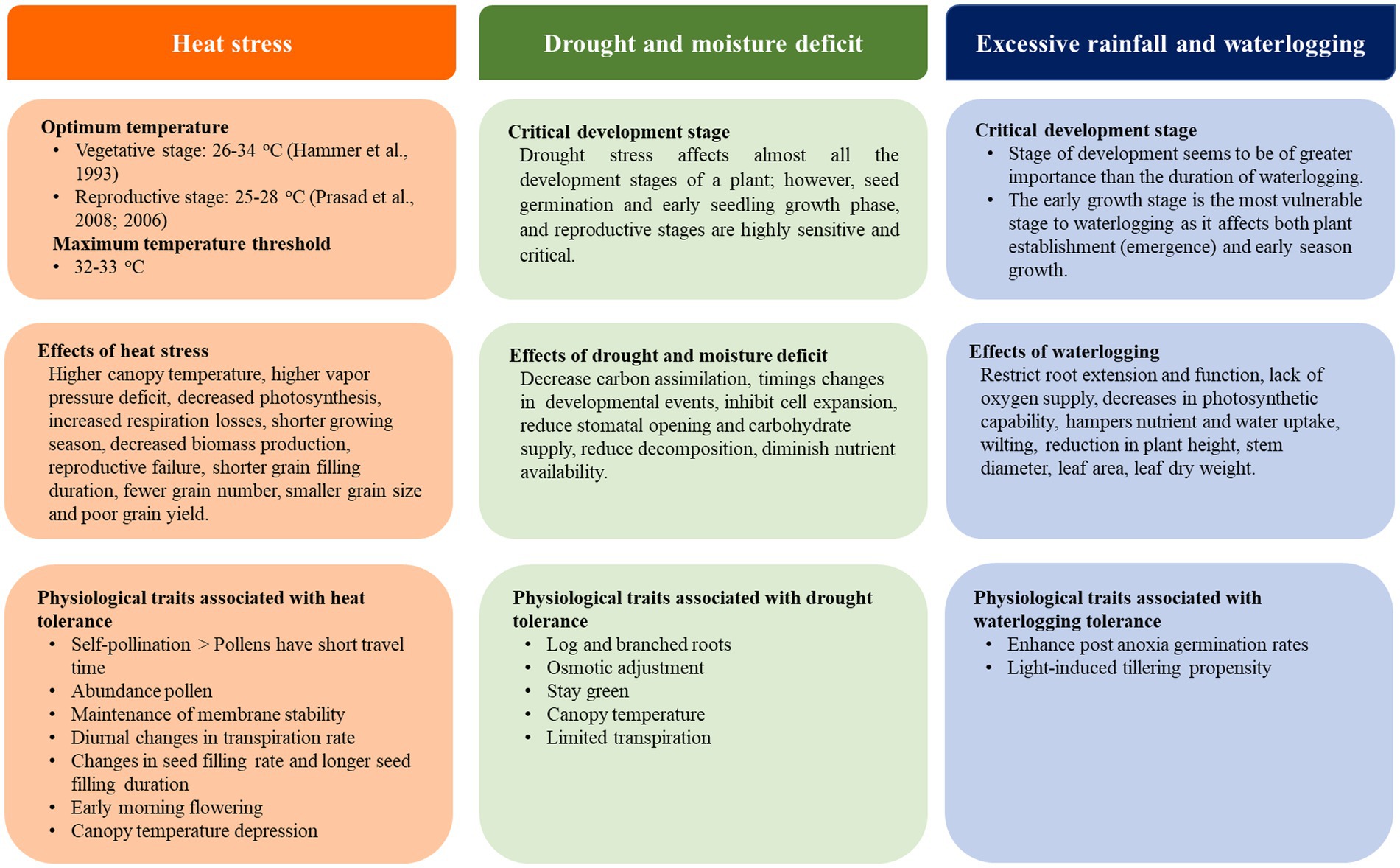
Figure 10. Summarized information regarding the three main abiotic stress that affect sorghum: (i) heat stress, (ii) drought and moisture deficit, and (iii) excessive rainfall and waterlogging.
5.2.1. Heat stress
Temperature is an important controlling factor influencing sorghum yield. For instance, it might explain the low yield of sorghum in some countries (e.g., with low temperatures) compared to their relatively high use of fertilizer. Sorghum can tolerate high temperatures better than other crops (Ciais et al., 2005). Several crop characteristics are contributing to this relative tolerance. This includes pollen and pollination process characteristics. According to Tack et al. (2017), the pollination process in sorghum is one of the critical processes that contribute to the higher tolerance of sorghum to heat stress. Self-pollination in sorghum decreases the travel distance of pollens, leading to less exposure to heat. With an abundance production of pollen from sorghum panicles, the pollen likelihood of survival is higher than in other crops. Early-morning release of pollens allows sorghum to avoid higher temperature levels during the day. Other characteristics that are associated with heat tolerance in this crop involve diurnal changes in transpiration rate, changes in seed filling rate and longer seed filling duration, and mechanisms for canopy temperature depression.
Although all these characteristics make sorghum stand out regarding tolerance to heat stress, high temperature that exceeds certain levels might be harmful to crop development (Tack et al., 2017). This heat stress might lead to a considerable reduction in the sorghum yield (Prasad et al., 2015). It has been experimentally demonstrated that a daily temperature between 32 and 33°C is the maximum threshold beyond which sorghum yield is severely affected and begins to drop (Tack et al., 2017; Prasad et al., 2019). The optimum temperature for sorghum development is found to vary for the different crop development stages. It seems that during the vegetative stages, the optimum temperature is between 26 and 34°C (Hammer et al., 1993). The reproductive stages are more sensitive to high temperatures than the vegetative stages (Prasad et al., 2015, 2021), and the optimum temperature for sorghum in these stages is between 25 and 28°C (Prasad et al., 2006, 2008). Therefore, heat stress due to future climate change might have negative impacts on sorghum yield in regions where the temperature is already at or beyond the optimum temperature, mostly in arid and sub-arid regions. In contrast, other regions with sub-optimal temperatures can be pushed towards the optimum temperature range due to climate change, benefiting crop development (De Boeck et al., 2010; Choi and Eltahir, 2023).
Many countries are already at or already crossed the critical temperature threshold (> 30°C), and sorghum cultivation in these countries might be at risk of heat stress as climate change increases the temperature in the future. The countries that are in a critical position in the temperature range include Pakistan and India (South Asia); Niger, Sudan, Mali, Senegal, and Burkina Faso (sub-Saharan Africa); Thailand (East Asia), and Uzbekistan (Central Asia). Climate change might push temperatures in some Latin American and European countries to more favorable conditions, benefiting sorghum yield.
We reviewed multiple articles on the impact of heat stress on sorghum. These studies used different types of climate models and forecasting scenarios (Table 3). In most of the studies, the long-term impact is higher than short- and medium-term climate change. Based on the region and climate model used, these studies predict reductions of up to 41% of the current sorghum yield in the future due to climate change (Table 4). These findings urge the development and adoption of effective adaptation measures to sustain sorghum production in a warming world.
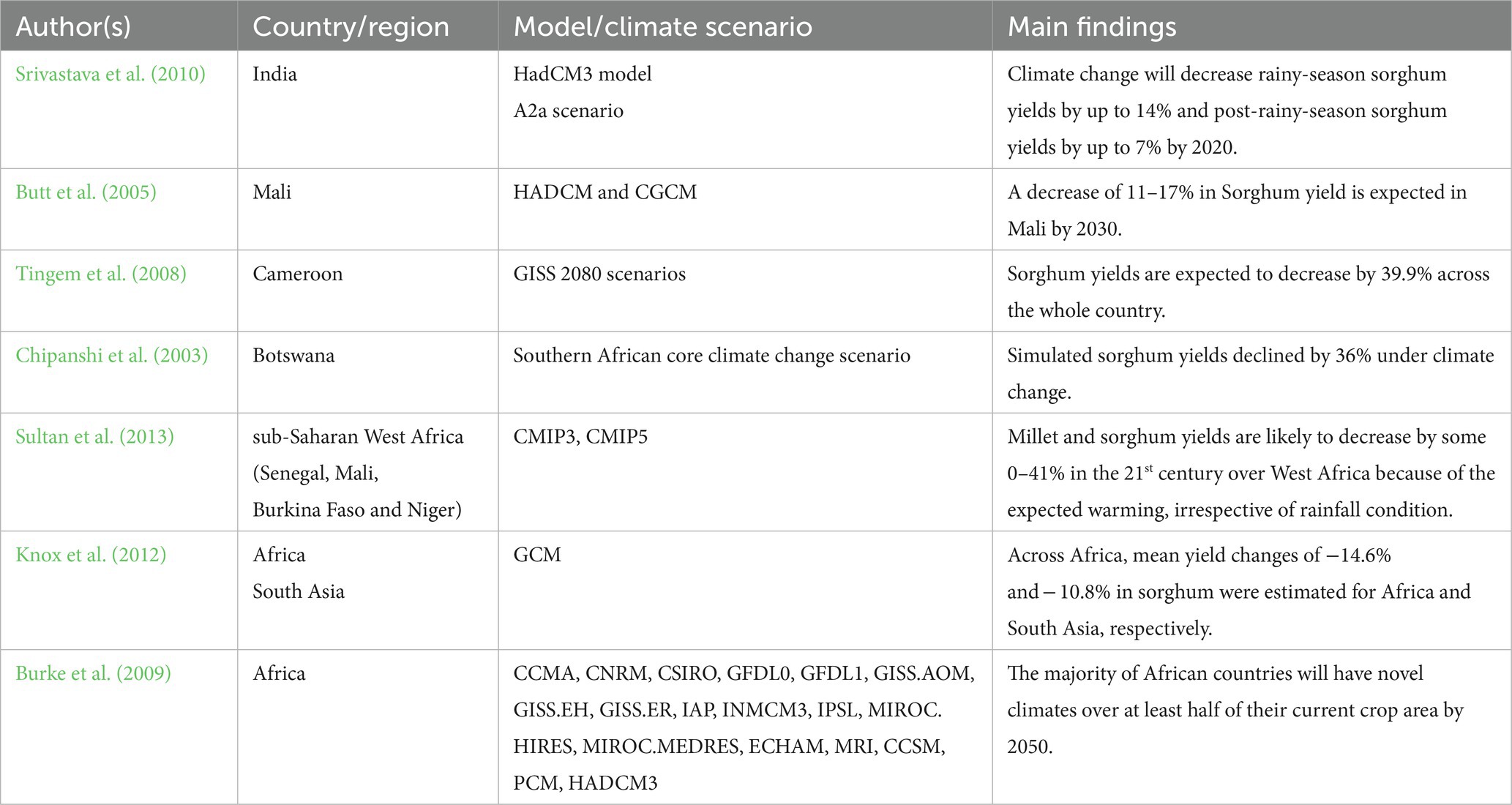
Table 3. Example studies investigated the impact of future climate change on sorghum yield using different climate models and scenarios and their main findings.
5.2.2. Drought and moisture deficit
Not only does sorghum withstand high temperatures, but it also can tolerate drought and moisture deficit better than other crops (Mastrorilli et al., 1999). It grows well under average seasonal rainfall of 500 mm (Elramlawi et al., 2020; Krishna, 2021). Sorghum developed two important strategies for adaptation to water deficit. The primary way is the tolerance of water availability decrease, while the second mechanism is the escape from water stress due to deep and extensive root formation (Tari et al., 2013). The main drought tolerance mechanisms in sorghum involve osmotic and photosynthesis adjustment (Hsiao, 1973; Boyer and Kramer, 1995; Goche et al., 2020). Morphological and physiological characteristics of sorghum that make it more drought-tolerant than other crops include the dense roots systems (Mayaki et al., 1976), the ability to maintain stomatal opening and photosynthesis at low water availability, plasticity in leaf area, and the associated reduction in canopy synthesis (Blum, 1996), and the ability for osmotic adjustment (Ludlow et al., 1990; Santamaria et al., 1990). High drought tolerance is associated with their high water extraction efficiency from the soil and the characteristics of the crop nodal roots (Salih et al., 1999). A high, constant hydraulic resistance to water flow also might decrease transpiration in sorghum (Zhang and Kirkham, 2000). These adjustments depend on the rate of development of the drought (Blum, 1996) and vary with the species (Turner, 2018) and severity of the stress (Turner and Jones, 1980). According to some studies, late-flowering varieties of sorghum can tolerate water deficit more effectively (Hsiao et al., 1976).
Although sorghum is considered a drought-tolerant crop and can be productive under low-input conditions, moisture stress affects the capability of sorghum’s soil-nutrient uptake and nutrient mobilization and transport (Yu et al., 2015; Sarshad et al., 2021). As stated by Obour et al. (2022), soil moisture deficit, besides low soil nitrogen, are the major factor that controls sorghum productivity and profitability under dryland conditions. This stress is regarded as the most frequent abiotic stress that sorghum faces in its major production areas (Assefa et al., 2010), and it can negatively affect sorghum productivity (Table 4). According to literature, 60% of the land in sub-Saharan Africa where sorghum is commonly grown is considered vulnerable to frequent droughts (Hadebe et al., 2017), and 80% of sorghum cultivated in the United States is grown under non-irrigated conditions, where water is a major limiting factor, which substantially reduces yield (Crasta et al., 1999).
Moisture deficit can occur at almost all the development stages of a crop (Prasad et al., 2021). The effect of temporary water stress on the yield and sensitivity of crops depend on the phenological stage in which it happens (Mastrorilli et al., 1999; Tari et al., 2013). It seems that the vegetative stage, especially seed germination, and early seedling growth phases are more sensitive to this type of stress (Mastrorilli et al., 1999; Prasad et al., 2021). In some field experiments, a moisture deficit in the vegetative stage was found to reduce biomass production by 30% compared to the control (Mastrorilli et al., 1999). Although the reproductive stage of development, especially after or at the ending phases of grain filling, seems to be generally more resistant to water limitation (Sarshad et al., 2021). This is mainly because of lower water requirements at the last crop development stages. However, water shortage at this stage can also reduce crop yield (Younis et al., 2000; Tingting et al., 2010).
Wilting of leaves, reduction in leaf area, bud/flower formation, sink numbers, and overall growth and yield are the main visible symptoms of drought and moisture deficit (Prasad et al., 2021). The most important visible symptoms of water stress in sorghum include slow leaf rolling and leaf yellowing (Orchard and Jessop, 1984).
5.2.3. Excessive rainfall and waterlogging
Waterlogging (also called flood, submergence, soil saturation, anoxia, and hypoxia) is one of the main abiotic stresses that threaten agriculture activities. Over the last decades, the number of waterlogging episodes on croplands has increased worldwide, mainly due to more intense and unpredictable rainfall events associated with climate change (Hirabayashi et al., 2013). In the United States, it represents a major problem for crop cultivation, with approximately 12% of cultivated soils affected by excess moisture (Boyer, 1982). It is estimated that losses in crop production due to flooding were second only to drought in many of the past years in the United States (Bailey-Serres et al., 2012; Li et al., 2019). This phenomenon is expected to increase as a consequence of global climate change, limiting crop production in various parts of the world (Ploschuk et al., 2018). Table 5 summarizes several examples of the impact of waterlogging on sorghum from different countries.
Because sorghum has a strong resistance to unfavorable conditions, it is usually grown in marginal areas such as low-lying regions and flooded areas with poor drainage (Huang, 2018). This makes it exposed to waterlogging stress. Sorghum withstands temporary waterlogging (Purseglove, 1986; Tari et al., 2013; Quinn et al., 2015; Matsuura et al., 2016), and is relatively more tolerant to waterlogging than other crops such as corn (Müller et al., 2020) and maize (von Haden et al., 2021). However, it shows major differences in cultivar sensitivity to waterlogging (Müller et al., 2020). It is well documented that considerable variation in waterlogging tolerance exists both between and within species (Orchard and Jessop, 1984). For example, sorghum varieties with high root growth may not be suitable for production in flooding-prone areas (Promkhambut et al., 2011). Waterlogging limits the sorghum root extension (Pardales et al., 1991) and severely affects the root function (Zhang et al., 2019). Moreover, it influences oxygen supply, hindering nutrient and water uptake (Ahmed et al., 2012). The change in leaf water could lead to a decline in photosynthetic capabilities (Zhang et al., 2019), which consequently affects crop yield. Visible symptoms of waterlogging in sorghum include reduction in root growth and rise in root death, increase in the number of nodal root axes, late tillering, reduction in leaf area and stem extension, rapid wilting of leaves, and yellowing of mature leaves (Jackson, 1956; Howell et al., 1976; Orchard and Jessop, 1984; Orchard et al., 1986; Pardales et al., 1991; Promkhambut et al., 2011; Zhang et al., 2016; Kadam et al., 2017).
The response of plants to waterlogging is usually considered to be dependent on genotype, environmental conditions, stage of development, and the duration of the waterlogging period (Russell, 1959; Cannell, 1977). The tolerance mechanism in sorghum to waterlogging includes higher seedling emergence rates (von Haden et al., 2021), higher tolerance of seed germination to anoxia, greater propensity for tillering (Al-Ani et al., 1985; Alam et al., 2017), and development of adventitious and nodal roots during waterlogging (Pardales et al., 1991; Matsuura et al., 2005). In addition, sorghum can adapt to waterlogging stress by reducing leaf chlorophyll and changing fluorescence parameters (Zhang et al., 2019). According to Orchard and Jessop (1984), the development stage when excessive moisture stress occurs is more important in determining the impact on sorghum yield than for how long the stress persists. However, the more the duration of the waterlogging event, the more severe the impact on sorghum will be. Although some previous reviews did not find a consistent pattern of plant damage from waterlogging at different stages of crop development (Orchard and Jessop, 1984), it seems that waterlogging stress at the early development stages is more impactful than in the late stages, because it affects plant establishment/emergence of the crop (Promkhambut et al., 2011; von Haden et al., 2021). Howell et al. (1976) found that waterlogging for 12 days reduces sorghum yield by 30, 26, and 0% at the early vegetative growth stage, early boot stage, and after grain heading stage, respectively. The impact of waterlogging on sorghum can be harmful (Zhang et al., 2016).
5.2.4. Synergetic effects between abiotic stresses
Although the effects of the three main abiotic stresses, i.e., heat stress, drought, and waterlogging, are usually studied independently, these stresses are strongly coupled. There are many synergetic effects between them, making their combined effect more detrimental than individual effects (Dreesen et al., 2012). The coupled impacts affect almost all morphological, physiological, and biochemical aspects of crop productivity (Ndlovu et al., 2021). Heat and drought are strongly coupled (Luo, 2007; Luo et al., 2008; Seneviratne et al., 2010) as heat stress often leads to moisture stress (Król, 2013), and drought can occur due to exceptional heat stress and low humidity (Prasad et al., 2021). The severity of the moisture deficit effect appears to be highly dependent on the temperature (Machado and Paulsen, 2001). In semi-arid regions, heat stress often overlaps with drought stress (Zandalinas et al., 2017). Recent research suggested that one of the main channels by which warming temperatures can affect sorghum yields is through its influence on water stress/drought conditions (Lobell et al., 2015). Based on crop modeling, the worst case of reduction in sorghum yield in the future due to climate change occurs when both heat and water stresses are combined (Sultan et al., 2013). Waterlogging is also associated with temperature (Zhang et al., 2019). Waterlogging impact on the crop can be amplified if associated with a rise in the temperature of floodwater (Fausey and McDonald, 1985).
5.3. Strategies to reduce climate risk on sorghum
5.3.1. Adequate use of fertilizers
As shown in our climate risk assessment (Section 4.4), sorghum yield in many counties such as Egypt, Uzbekistan, Paraguay, Saudi Arabia, and Oman exhibit higher magnitudes than in other countries despite being located in hot temperature conditions and receiving negligible amounts of rain. Sorghum is cultivated in these high-yield countries mainly under high rates of fertilizer use (Table 1), which enhance their adaptive capacity and contributed to the improved crop yield. This suggests that adequate use of fertilizer might offset the impact of unfavorable climate conditions.
It is well-documented that sorghum responds positively to fertilizers. Sánchez (2010) argued that without soil replenishment, even the best crop varieties and the most informed policies cannot prevent hunger in Africa. Especially where poor sorghum yield is prevailing, adding adequate quantities of chemical fertilizers and/or organic manures could enhance the crop yield noticeably. A review conducted by Pal et al. (1982) on the response of grain sorghum to inorganic fertilizers concluded that improved varieties and hybrids of sorghum respond to N and P rates up to 150 and 40 kg/ha, respectively. Examples in Africa of positive impacts of using fertilizers on sorghum yield include applications in Ethiopia (Bayu et al., 2006; Sebnie et al., 2020; Temeche et al., 2021), Senegal (Ganyo et al., 2019), and Ghana (Buah et al., 2012). The positive impact of the use of fertilizers extends also to sorghum’s grain quality (Kaufman et al., 2013; Babiker, 2015; Nokerbekova et al., 2018; Astuti et al., 2019), and mitigating some abiotic stresses such as salinity stress (Zamani et al., 2020).
The low use of fertilizers in agriculture can be considered a major obstacle to food security and poverty reduction efforts in sub-Saharan Africa (Koussoubé and Nauges, 2017). This low level of usage can be attributed to many factors, including, fertilizers prices (Cedrez et al., 2020), the financial status of farmers (Khalifa et al., 2020), and the lack of access to credits (Koussoubé and Nauges, 2017). In many regions in sub-Saharan Africa and South Asia, especially under rainfed conditions, farmers are applying no or low levels of inputs (e.g., fertilizers) as a risk-averse strategy in response to the fluctuating and unreliable rainfall (Ali, 2019; Begho et al., 2022). Promoting the use of fertilizers, especially among smallholder farmers, requires an economic optimization of the fertilizer type, quantity, and timing that increase yield with an acceptable profit margin (Kaizzi et al., 2012; Hegano et al., 2016). These conditions differ from case to case, implying different needs for fertilizer types and quantities. For instance, while 46 kg/ha of N in two split doses is recommended for farmers in lowland clay soil in Ethiopia (Temeche et al., 2021), N and P combination of 40:0 and 40:17 kg/ha is recommended for sorghum cultivated in sandy loam in Guinea savanna zone of Ghana (Buah et al., 2012). Mapping soil nutrient deficiency is critical as it enables determining the required fertilizer types and quantities needed for a specific location and a certain crop type. Hence, soil fertility mapping is an essential prerequisite for the effective and sustainable use of fertilizer that aim at improving crop productivity while minimizing the negative impacts of excessive fertilizer use.
5.3.2. Efficient irrigation
Although the effect of fertilizer application is obvious, the magnitude of the positive impact might differ between countries. Some countries with relatively high levels of fertilizer use, though, still exhibit relatively lower (or equal) sorghum yield compared to countries that use comparable amounts of fertilizers. This indicates that other controlling factors such as sorghum varieties, varieties’ tolerance to biotic and abiotic stress, and agronomic practices are also playing roles in determining crop yield. In particular, it seems that low adoption of irrigation and deficiencies in irrigation systems contribute substantially to preserving the low crop yield in these countries. For instance, although Pakistan shows a relatively high level of fertilizer use and high irrigation percentage of agricultural land, its average yield of sorghum is rather low. See Figure S3 in the supplementary information for a visualized representation of fertilizer use, %irrigation, and yield in these 17 countries. This suggests that factors such as water management, and deterioration of irrigation infrastructure are playing a role in suppressing crop yield in this country (Muzammil et al., 2021).
5.3.3. Improved varieties and better agronomic practices
A large body of literature found important differences between sorghum varieties in terms of yield, nutritional content, and tolerance to climate risks (Amare et al., 2015; Ogbaga et al., 2016; Assefa et al., 2020; Tasie and Gebreyes, 2020; Wendmu et al., 2022). Similarly, agronomic practices such as tillage, plant density, and sowing date, among others, are found to widely impact sorghum yield (Conley and Wiebold, 2003; Ajaj et al., 2021; Carcedo et al., 2021; Zander et al., 2021; Gao et al., 2022; Naoura et al., 2023), indicating that adopting best agronomic practices is an indispensable factor for any program that aims to improve crop productivity and build climate resilience.
To conclude, adequate use of fertilizers with reliable and adequate irrigation, supplemented with efficient management, improved varieties, and better agronomic practices, are likely to boost the sorghum yield in regions suffering from low crop yield and minimize the potential impacts of climate change in high-risk countries.
6. Summary and conclusion
Sorghum has a relatively higher tolerance to harsh environmental conditions compared to other crops. However, there are many sources of concern regarding the sustainability of sorghum cultivation under climate change. This includes, among others, the severe reduction in harvested area and yield experienced in some of the largest global sorghum producers and the serious imbalance in sorghum yield between regions and economies. Moreover, the relatively slow rate of improvement in sorghum yield based on the work of breeding programs, and the competition over water and land with biofuel production are other sources of concern regarding sorghum sustainability.
This study provides a comprehensive assessment of the current status of sorghum production, its vulnerability and resilience to climate risk. It consists of four assessments that involve (i) evaluation of sorghum production status and trends, (ii) estimates of yield gap in sub-Saharan Africa, (iii) assessment of deficit in soil nutrient replenishment, and (iv) evaluation of climate risk. The knowledge created in this research provides valuable insights into where and how to interfere in order to enhance the global production of sorghum, and highlights which strategies are most effective to strengthen sorghum resilience to climate change.
The wide yield gap in sub-Saharan Africa is a serious challenge for food security that jeopardize food security in this region. However, it highlights huge hidden opportunities available to boost global sorghum production. Bridging the yield gap to the country-specific maximum achieved yield in 31 sub-Saharan African countries would increase sorghum production from 22.7 to 29.4 million t. The potential production of 29.4 million t from these countries represents more than 50.7% of the current global sorghum production. Given the fact that the potential yield might be much larger than the ones that have been considered in the current investigation, this implies higher potential production to be expected from this region if the yield gap is bridged. Focusing on bridging the sorghum yield gap in countries such as Nigeria, Sudan, and Ethiopia would generate most of this potential increase in production, mainly due to their large harvested areas of sorghum.
The low yield in many sub-Saharan African countries is possibly a direct result of the low use of improved varieties, highly variable rainfall, and the low use of fertilizers (Dembele et al., 2021). Although this low yield is a challenge for food security in these countries, it represents an opportunity to attain higher sorghum production from the same cultivated areas by using a combination of fertilizers, new cultivars, and improved agronomic practices (Sanders et al., 2019).
Despite the relative resilience of sorghum to stresses, this crop can be affected by multiple biotic and abiotic stresses. Among abiotic stresses, heat stress, moisture deficit, and waterlogging might be the most pressing challenges for sorghum cultivation in the future under climate change. Our current investigation identified several countries where sorghum is cultivated under unfavorable climate conditions. Characteristics of these countries helped us to derive some insights on how to increase the resilience of sorghum under climate change. It seems that adequate fertilization and irrigation can play a critical role in improving sorghum tolerance to unfavorable climate conditions. However, better soil fertilization and better management of soil moisture alone may not solve sorghum production challenges. Rather, a combination of strategies that include soil fertility management, efficient water management, improved crop varieties, and better agronomic practices might be more effective. The wide gap between the low-income and the higher income countries in terms of advanced technologies, financial resources, and agricultural practices suggests major efforts by developing countries on technology adoption, policy reform and enforcement need to be carried out to achieve higher crop yield.
For future research, knowledge regarding traits associated with heat, moisture deficit, and waterlogging tolerance needs to be improved (Promkhambut et al., 2011; Zhang et al., 2016; Kadam et al., 2017; Prasad et al., 2021). In addition, investigating the synergies between the three stresses is still limited (Machado and Paulsen, 2001; Dreesen et al., 2012) and needs more investigation.
Data availability statement
Publicly available datasets were analyzed in this study. This data can be found at: FAOSTAT: https://www.fao.org/faostat/en/#home; AQUASTAT: https://www.fao.org/aquastat/en/databases/; Crop calenders: https://www.fao.org/giews/en/ Crop distribution: https://mapspam.info/; and Rainfall and temperature (CRU TS v. 4.05): https://crudata.uea.ac.uk/cru/data/hrg/cru_ts_4.05/.
Author contributions
MK and EE contributed to the conception and design of the study. MK processed and analyzed the data and wrote the first draft of the manuscript. All authors contributed to the manuscript revision and approved the submitted version.
Funding
This research has been conducted under the framework of the project “Impact of Near-term Climate Change on Water Availability and Food Productivity in Africa,” funded by the Abdul Latif Jameel Water and Food Systems Lab (J-WAFS) at the Massachusetts Institute of Technology (MIT).
Acknowledgments
The authors extend their thanks to the providers of FAOSTAT, AQUASTAT, GIEWS, IPAD-USDA, CRU TS, GADM, and SPAM datasets. We thank Professor William Rooney (Texas A&M University, USA) for the insightful disucssion and helpful literature. Finally, we thank the two reviewers for their careful reading of our manuscript and their many insightful comments and suggestions on the earlier version of the manuscript.
Conflict of interest
The authors declare that the research was conducted in the absence of any commercial or financial relationships that could be construed as a potential conflict of interest.
Publisher’s note
All claims expressed in this article are solely those of the authors and do not necessarily represent those of their affiliated organizations, or those of the publisher, the editors and the reviewers. Any product that may be evaluated in this article, or claim that may be made by its manufacturer, is not guaranteed or endorsed by the publisher.
Supplementary material
The Supplementary material for this article can be found online at: https://www.frontiersin.org/articles/10.3389/fsufs.2023.1184373/full#supplementary-material
References
Abreha, K. B., Enyew, M., Carlsson, A. S., Vetukuri, R. R., Feyissa, T., Motlhaodi, T., et al. (2021). Sorghum in dryland: morphological, physiological, and molecular responses of sorghum under drought stress. Planta 255:20. doi: 10.1007/s00425-021-03799-7
Ahmed, F., Rafii, M. Y., Ismail, M. R., Juraimi, A. S., Rahim, H. A., Asfaliza, R., et al. (2012). Waterlogging tolerance of crops: breeding, mechanism of tolerance, molecular approaches, and future prospects. Biomed. Res. Int. 2013:e963525, 1–10. doi: 10.1155/2013/963525
Ajaj, H. A., Mohammed, Y. A., Alrubaya, A. A., and Addaheri, A. M. (2021). Effect of planting dates on the growth, yield and quality of three cultivars of Sorghum (Sorghum bicolor L. Moench). IOP Conf. Ser. Earth Environ. Sci. 904:012019. doi: 10.1088/1755-1315/904/1/012019
Alam, M. M., van Oosterom, E. J., Cruickshank, A. W., Jordan, D. R., and Hammer, G. L. (2017). Predicting Tillering of diverse Sorghum germplasm across environments. Crop Sci. 57, 78–87. doi: 10.2135/cropsci2016.04.0262
Al-Ani, A., Bruzau, F., Raymond, P., Saint-Ges, V., Leblanc, J. M., and Pradet, A. (1985). Germination, respiration, and adenylate energy charge of seeds at various oxygen partial pressures. Plant Physiol. 79, 885–890. doi: 10.1104/pp.79.3.885
Ali, E. (2019). Farmers’ attitudes towards climate risks and effects of farmers’ risk aversion behavior on inputs use in northern Togo. SJA 35. doi: 10.17582/journal.sja/2019/35.3.663.674
Amare, K., Zeleke, H., and Bultosa, G. (2015). Variability for yield, yield related traits and association among traits of Sorghum (Sorghum Bicolor (L.) Moench) varieties in Wollo, Ethiopia. Journal of Plant Breeding and Crop Science. 7, 125–133. doi: 10.5897/JPBCS2014.0469
Ananda, G. K. S., Myrans, H., Norton, S. L., Gleadow, R., Furtado, A., and Henry, R. J. (2020). Wild Sorghum as a promising resource for crop improvement. Front. Plant Sci. 11:1108. doi: 10.3389/fpls.2020.01108
Arora, G., Feng, H., Anderson, C. J., and Hennessy, D. A. (2020). Evidence of climate change impacts on crop comparative advantage and land use. Agric. Econ. 51, 221–236. doi: 10.1111/agec.12551
Assefa, A., Bezabih, A., Girmay, G., Alemayehu, T., and Lakew, A. (2020). Evaluation of sorghum (Sorghum bicolor (L.) Moench) variety performance in the lowlands area of wag lasta, north eastern Ethiopia. Cogent Food Agric. 6:1778603. doi: 10.1080/23311932.2020.1778603
Assefa, Y., Staggenborg, S. A., and Prasad, V. P. V. (2010). Grain Sorghum water requirement and responses to drought stress: a review. Crop Manag 9, 1–11. doi: 10.1094/CM-2010-1109-01-RV
Astuti, D., Suhartanto, B., Umami, N., and Irawan, A. (2019). Productivity, nutrient composition, and hydrocyanic acid concentration of Super-2 forage Sorghum at different NPK levels and planting spaces. Tropical Anim. Sci. J. 42, 189–195. doi: 10.5398/tasj.2019.42.3.189
Ayyad, S., and Khalifa, M. (2021). Will the eastern Nile countries be able to sustain their crop production by 2050? An outlook from water and land perspectives. Sci. Total Environ. 775:145769. doi: 10.1016/j.scitotenv.2021.145769
Babiker, E. (2015). Effect of different levels of micronutrients fertilizer on protein and basic and acidic amino acids contents of grains of Sorghum cultivars. J. Agric. Sci. 21, 159–166. doi: 10.15832/tbd.26636
Bailey-Serres, J., Lee, S. C., and Brinton, E. (2012). Waterproofing crops: effective flooding survival strategies. Plant Physiol. 160, 1698–1709. doi: 10.1104/pp.112.208173
Bayu, W., Rethman, N. F. G., Hammes, P. S., and Alemu, G. (2006). Effects of farmyard manure and inorganic fertilizers on Sorghum growth, yield, and nitrogen use in a semi-arid area of Ethiopia. J. Plant Nutr. 29, 391–407. doi: 10.1080/01904160500320962
Begho, T., Eory, V., and Glenk, K. (2022). Demystifying risk attitudes and fertilizer use: a review focusing on the behavioral factors associated with agricultural nitrogen emissions in South Asia. Front. Sustain. Food Syst. 6. doi: 10.3389/fsufs.2022.991185
Blum, A. (1996). Crop responses to drought and the interpretation of adaptation. Plant Growth Regul. 20, 135–148. doi: 10.1007/BF00024010
Boyer, J. S. (1982). Plant productivity and environment. Science 218, 443–448. doi: 10.1126/science.218.4571.443
Boyer, J. S., and Kramer, P. J. (1995). Water Relations of Plants and Soils. 1st edition. MA, USA: Academic Press, Inc, 512.
Buah, S. S. J., Kombiok, J. M., and Abatania, L. N. (2012). Grain Sorghum response to NPK fertilizer in the Guinea savanna of Ghana. J. Crop Improv. 26, 101–115. doi: 10.1080/15427528.2011.616625
Burke, M. B., Lobell, D. B., and Guarino, L. (2009). Shifts in African crop climates by 2050, and the implications for crop improvement and genetic resources conservation. Glob. Environ. Chang. 19, 317–325. doi: 10.1016/j.gloenvcha.2009.04.003
Butt, T. A., McCarl, B. A., Angerer, J., Dyke, P. T., and Stuth, J. W. (2005). The economic and food security implications of climate change in Mali. Clim. Chang. 68, 355–378. doi: 10.1007/s10584-005-6014-0
Cannell, R. Q. (1977). Soil aeration and compaction in relation to root growth and soil management. Appl. Biol. 2, 1–86.
Carcedo, A. J. P., Cejas, E., and Gambin, B. L. (2021). Adapting sorghum sowing date and genotype maturity to seasonal rainfall variation in a temperate region. In silico Plants 3:diab007. doi: 10.1093/insilicoplants/diab007
Cedrez, C. B., Chamberlin, J., Guo, Z., and Hijmans, R. J. (2020). Spatial variation in fertilizer prices in sub-Saharan Africa. PLoS One 15:e0227764. doi: 10.1371/journal.pone.0227764
Chipanshi, A. C., Chanda, R., and Totolo, O. (2003). Vulnerability assessment of the maize and Sorghum crops to climate change in Botswana. Clim. Chang. 61, 339–360. doi: 10.1023/B:CLIM.0000004551.55871.eb
Choi, Y.-W., and Eltahir, E.A.B. (2023). Near-term climate change impacts on food crops productivity in East Africa. Theor Appl Climatol, 152, 843–860. doi: 10.1007/s00704-023-04408-1
Ciais, P., Reichstein, M., Viovy, N., Granier, A., Ogée, J., Allard, V., et al. (2005). Europe-wide reduction in primary productivity caused by the heat and drought in 2003. Nature 437, 529–533. doi: 10.1038/nature03972
Conley, S. P., and Wiebold, W. J. (2003). Grain Sorghum response to planting date. Crop Manag 2, 1–5. doi: 10.1094/CM-2003-0204-01-RS
Crasta, O. R., Xu, W. W., Rosenow, D. T., Mullet, J., and Nguyen, H. T. (1999). Mapping of post-flowering drought resistance traits in grain sorghum: association between QTLs influencing premature senescence and maturity. Mol. Gen. Genet. 262, 579–588. doi: 10.1007/s004380051120
Damerau, K., Patt, A. G., and van Vliet, O. P. R. (2016). Water saving potentials and possible trade-offs for future food and energy supply. Glob. Environ. Chang. 39, 15–25. doi: 10.1016/j.gloenvcha.2016.03.014
de BOECK, H. A. N. S. J., DREESEN, F. E., JANSSENS, I. A., and NIJS, I. (2010). Climatic characteristics of heat waves and their simulation in plant experiments. Glob. Chang. Biol. 16, 1992–2000. doi: 10.1111/j.1365-2486.2009.02049.x
de Wet, J. M. J. (1978). Systematics and evolution of Sorghum sect. Sorghum (Gramineae). Am. J. Bot. 65, 477–484. doi: 10.2307/2442706
Dembele, J. S. B., Gano, B., Kouressy, M., Dembele, L. L., Doumbia, M., Ganyo, K. K., et al. (2021). Plant density and nitrogen fertilization optimization on sorghum grain yield in Mali. Agron. J. 113, 4705–4720. doi: 10.1002/agj2.20850
Dreesen, F. E., De Boeck, H. J., Janssens, I. A., and Nijs, I. (2012). Summer heat and drought extremes trigger unexpected changes in productivity of a temperate annual/biannual plant community. Environ. Exp. Bot. 79, 21–30. doi: 10.1016/j.envexpbot.2012.01.005
Elramlawi, H. R., Mohammed, H. I., Elamin, A. W., Abdallah, O. A., and Taha, A. A. A. M. (2020). “Adaptation of Sorghum (Sorghum bicolor L. Moench) crop yield to climate change in eastern dryland of Sudan” in Handbook of Climate Change Resilience. ed. W. Leal Filho (Cham: Springer International Publishing), 2549–2573.
ESRI (2014). ArcGIS desktop: Release 10.3.1. Redlands, CA: Environmental Systems Research Institute.
Fakrudin, B., Lakshmidevamma, T. N., Ugalat, J., Gunnaiah, R., Khan, J., Gautham Suresh, S. P., et al. (2021). “Genomic designing for biotic stress resistance in Sorghum” in Genomic Designing for Biotic Stress Resistant Cereal Crops. ed. C. Kole (Cham: Springer International Publishing), 213–255.
FAO (2022a). Crops and Livestock Products. United Nation Food and Agriculture Organization. Rome: FAO.
Fausey, N. R., and McDonald, M. B. Jr. (1985). Emergence of inbred and hybrid corn following Flooding1. Agron. J. 77, 51–56. doi: 10.2134/agronj1985.00021962007700010013x
Fernandes, M., Vieira da Silva, C., and Frazão Santos, C. (2022). Climate-related vulnerability and risk assessment of Main Ocean uses: An overview. Front. Mar. Sci. 9. doi: 10.3389/fmars.2022.787882
Ganyo, K. K., Muller, B., Ndiaye, M., Gaglo, E. K., Guissé, A., and Adam, M. (2019). Defining fertilization strategies for Sorghum (Sorghum bicolor (L.) Moench) production under Sudano-Sahelian conditions: options for late basal fertilizer application. Agronomy 9:697. doi: 10.3390/agronomy9110697
Gao, F.-C., Yan, H.-D., Gao, Y., Huang, Y., Li, M., Song, G.-L., et al. (2022). Interpretation of genotype-environment-sowing date/plant density interaction in sorghum [Sorghum bicolor (L.) Moench] in early mature regions of China. Front. Plant Sci, 13. doi: 10.3389/fpls.2022.1008198/full
Gebrehiwot, K. A. (2018). A review on waterlogging, salinization and drainage in Ethiopian irrigated agriculture. Sustain. Water Resour. Manag. 4, 55–62. doi: 10.1007/s40899-017-0121-8
Goche, T., Shargie, N. G., Cummins, I., Brown, A. P., Chivasa, S., and Ngara, R. (2020). Comparative physiological and root proteome analyses of two sorghum varieties responding to water limitation. Sci. Rep. 10:11835. doi: 10.1038/s41598-020-68735-3
Graham-Rowe, D. (2011). Agriculture: beyond food versus fuel. Nature 474, S6–S8. doi: 10.1038/474S06a
Hadebe, S. T., Modi, A. T., and Mabhaudhi, T. (2017). Drought tolerance and water use of cereal crops: a focus on Sorghum as a food security crop in sub-Saharan Africa. J. Agron. Crop Sci. 203, 177–191. doi: 10.1111/jac.12191
Hammer, G. L., Carberry, P. S., and Muchow, R. C. (1993). Modelling genotypic and environmental control of leaf area dynamics in grain sorghum. I Whole plant level. Field Crops Res 33, 293–310. doi: 10.1016/0378-4290(93)90087-4
Hao, H., Li, Z., Leng, C., Lu, C., Luo, H., Liu, Y., et al. (2021). Sorghum breeding in the genomic era: opportunities and challenges. Theor. Appl. Genet. 134, 1899–1924. doi: 10.1007/s00122-021-03789-z
Hegano, A., Adicha, A., and Tesema, S. (2016). Economic analysis of the effect of nitrogen and phosphorous fertilizer application for Sorghum production at Alduba, south Omo, South Western Ethiopia. Int. J. Agric. Econ. 1:26. doi: 10.11648/j.ijae.20160102.11
Hirabayashi, Y., Mahendran, R., Koirala, S., Konoshima, L., Yamazaki, D., Watanabe, S., et al. (2013). Global flood risk under climate change. Nat. Clim. Change 3, 816–821. doi: 10.1038/nclimate1911
Hochman, G., Kaplan, S., Rajagopal, D., and Zilberman, D. (2012). Biofuel and food-commodity prices. Agriculture 2, 272–281. doi: 10.3390/agriculture2030272
Howell, T. A., Hiler, E. A., Zolezzi, O., and Ravelo, C. (1976). Grain Sorghum response to inundation at three growth stages. Transactions of the ASAE 19, 876–880. doi: 10.13031/2013.36138
Hsiao, T. C. (1973). Plant responses to water stress. Annu. Rev. Plant Physiol. 24, 519–570. doi: 10.1146/annurev.pp.24.060173.002511
Hsiao, T. C., Fereres, E., Acevedo, E., and Henderson, D. W. (1976). “Water stress and dynamics of growth and yield of crop plants” in Water and Plant Life: Problems and Modern Approaches, Ecological Studies. eds. O. L. Lange, L. Kappen, and E.-D. Schulze (Berlin, Heidelberg: Springer), 281–305.
Huang, R. (2018). Research progress on plant tolerance to soil salinity and alkalinity in sorghum. J. Integr. Agric. 17, 739–746. doi: 10.1016/S2095-3119(17)61728-3
IFPRI (2019). Global spatially-disaggregated crop production statistics data for 2010 version 2.0. Int. Food Policy Res. Institute. doi: 10.7910/DVN/PRFF8V
Igartua, E., Gracia, M. P., and Lasa, J. M. (1994). Characterization and genetic control of germination-emergence responses of grain sorghum to salinity. Euphytica 76, 185–193. doi: 10.1007/BF00022163
Iizumi, T., and Ramankutty, N. (2015). How do weather and climate influence cropping area and intensity? Glob. Food Sec. 4, 46–50. doi: 10.1016/j.gfs.2014.11.003
Iqbal, A., Sadia, B., Khan, A. I., Awan, F. S., Kainth, R. A., and Sadaqat, H. A. (2010). Biodiversity in the sorghum (Sorghum bicolor L. Moench) germplasm of Pakistan. Genet. Mol. Res. 9, 756–764. doi: 10.4238/vol9-2gmr741
Jackson, W. T. (1956). The relative importance of factors causing injury to shoots of flooded tomato plants. Am. J. Bot. 43, 637–639. doi: 10.2307/2438827
Jurgilevich, A., Räsänen, A., Groundstroem, F., and Juhola, S. (2017). A systematic review of dynamics in climate risk and vulnerability assessments. Environ. Res. Lett. 12:013002. doi: 10.1088/1748-9326/aa5508
Kadam, S., Abril, A., Dhanapal, A. P., Koester, R. P., Vermerris, W., Jose, S., et al. (2017). Characterization and regulation of aquaporin genes of Sorghum [Sorghum bicolor (L.) Moench] in response to waterlogging stress. Front. Plant Sci. 8:862. doi: 10.3389/fpls.2017.00862
Kaizzi, K. C., Byalebeka, J., Semalulu, O., Alou, I., Zimwanguyizza, W., Nansamba, A., et al. (2012). Sorghum response to fertilizer and nitrogen use efficiency in Uganda. Agron. J. 104, 83–90. doi: 10.2134/agronj2011.0182
Kaufman, R. C., Wilson, J. D., Bean, S. R., Presley, D. R., Blanco-Canqui, H., and Mikha, M. (2013). Effect of nitrogen fertilization and cover cropping systems on Sorghum grain characteristics. J. Agric. Food Chem. 61, 5715–5719. doi: 10.1021/jf401179n
Khalifa, M., Elagib, N. A., Ahmed, B. M., Ribbe, L., and Schneider, K. (2020). Exploring socio-hydrological determinants of crop yield in under-performing irrigation schemes: pathways for sustainable intensification. Hydrol. Sci. J. 65, 153–168. doi: 10.1080/02626667.2019.1688333
Khan, N. A., Shah, A. A., Chowdhury, A., Tariq, M. A. U. R., and Khanal, U. (2022). Rice farmers’ perceptions about temperature and rainfall variations, respective adaptation measures, and determinants: implications for sustainable farming systems. Front. Environ. Sci. 10. doi: 10.3389/fenvs.2022.997673
Knox, J., Hess, T., Daccache, A., and Wheeler, T. (2012). Climate change impacts on crop productivity in Africa and South Asia. Environ. Res. Lett. 7:034032. doi: 10.1088/1748-9326/7/3/034032
Koussoubé, E., and Nauges, C. (2017). Returns to fertiliser use: does it pay enough? Some new evidence from sub-Saharan Africa. Eur. Rev. Agric. Econ. 44, 183–210. doi: 10.1093/erae/jbw018
Krishna, K. R. (2021). Agroecosystems: Soils, Climate, Crops, Nutrient Dynamics and Productivity. 1st Edn, New York, USA: Routledge—Taylor & Francis Group.
Król, A. (2013). The Growth and Water Uptake by Yellow Seed and Black Seed Rape Depending on the State Of Soil Compaction. Bohdan Dobrzañski Institute of Agrophysics PAS, Lublin.
Legwaila, G. M., Balole, T. V., and Karikari, S. K. (2003). Review of sweet sorghum: a potential cash and forage crop in Botswana. UNISWA J. Agric. 12, 5–14. doi: 10.4314/uniswa.v12i1.4631
Li, Y., Guan, K., Schnitkey, G. D., DeLucia, E., and Peng, B. (2019). Excessive rainfall leads to maize yield loss of a comparable magnitude to extreme drought in the United States. Glob. Chang. Biol. 25, 2325–2337. doi: 10.1111/gcb.14628
Liu, W., Ye, T., Jägermeyr, J., Müller, C., Chen, S., Liu, X., et al. (2021). Future climate change significantly alters interannual wheat yield variability over half of harvested areas. Environ. Res. Lett. 16:094045. doi: 10.1088/1748-9326/ac1fbb
Lobell, D. B., and Burke, M. B. (2008). Why are agricultural impacts of climate change so uncertain? The importance of temperature relative to precipitation. Environ. Res. Lett. 3:034007. doi: 10.1088/1748-9326/3/3/034007
Lobell, D. B., Hammer, G. L., Chenu, K., Zheng, B., McLean, G., and Chapman, S. C. (2015). The shifting influence of drought and heat stress for crops in Northeast Australia. Glob. Chang. Biol. 21, 4115–4127. doi: 10.1111/gcb.13022
Ludlow, M. M., Santamaria, J. M., and Fukai, S. (1990). Contribution of osmotic adjustment to grain yield in Sorghum bicolor (L.) Moench under water-limited conditions. II. Water stress after anthesis. Aust. J. Agric. Res. 41, 67–78. doi: 10.1071/ar9900067
Luo, Y. (2007). Terrestrial carbon–cycle feedback to climate warming. Annu. Rev. Ecol. Evol. Syst. 38, 683–712. doi: 10.1146/annurev.ecolsys.38.091206.095808
Luo, Y., Gerten, D., Le Maire, G., Parton, W. J., Weng, E., Zhou, X., et al. (2008). Modeled interactive effects of precipitation, temperature, and [CO2] on ecosystem carbon and water dynamics in different climatic zones. Glob. Chang. Biol. 14, 1986–1999. doi: 10.1111/j.1365-2486.2008.01629.x
Machado, S., and Paulsen, G. M. (2001). Combined effects of drought and high temperature on water relations of wheat and sorghum. Plant Soil 233, 179–187. doi: 10.1023/A:1010346601643
Manjarrez-Sandoval, P., González-Hernández, V. A., Mendoza-Onofre, L. E., and Engleman, E. M. (1989). Drought Stress Effects On The Grain Yield And Panicle Development Of Sorghum. Can. J. Plant Sci. 69, 631–641. doi: 10.4141/cjps89-079
Maranville, J. W., delRosario, D. A., Dalmacio, S. A., and Clark, R. B. (1986). Variability in growth and nutrient accumulation in sorghum grown in waterlogged soils. Commun. Soil Sci. Plant Anal. 17, 1089–1108. doi: 10.1080/00103628609367776
Mastrorilli, M., Katerji, N., and Rana, G. (1999). Productivity and water use efficiency of sweet sorghum as affected by soil water deficit occurring at different vegetative growth stages. Eur. J. Agron. 11, 207–215. doi: 10.1016/S1161-0301(99)00032-5
Matsuura, A., An, P., Murata, K., and Inanaga, S. (2016). Effect of pre- and post-heading waterlogging on growth and grain yield of four millets. Plant Prod. Sci. 19, 348–359. doi: 10.1080/1343943X.2016.1146907
Matsuura, H., Inanaga, S., Tetsuka, T., and Murata, K. (2005). Differences in vegetative growth response to soil flooding between common and Tartary buckwheat. Plant Prod. Sci. 8, 525–532. doi: 10.1626/pps.8.525
Mayaki, W. C., Stone, L. R., and Teare, I. D. (1976). Irrigated and nonirrigated soybean, corn, and grain Sorghum root systems 1. Agron. J. 68, 532–534. doi: 10.2134/agronj1976.00021962006800030028x
Monk, R., Franks, C., and Dahlberg, J. (2014). “Sorghum” in Yield Gains in Major U.S. Field Crops (USA: John Wiley & Sons, Ltd), 293–310.
Moore, F. C., Baldos, U., Hertel, T., and Diaz, D. (2017). New science of climate change impacts on agriculture implies higher social cost of carbon. Nat. Commun. 8:1607. doi: 10.1038/s41467-017-01792-x
Müller, M., Dembélé, S., Zougmoré, R. B., Gaiser, T., and Partey, S. T. (2020). Performance of three Sorghum cultivars under excessive rainfall and waterlogged conditions in the Sudano-Sahelian zone of West Africa: a case study at the climate-Smart Village of Cinzana in Mali. Water 12:2655. doi: 10.3390/w12102655
Muzammil, M., Zahid, A., and Breuer, L. (2021). Economic and environmental impact assessment of sustainable future irrigation practices in the Indus Basin of Pakistan. Sci. Rep. 11:23466. doi: 10.1038/s41598-021-02913-9
Nagaraj, N., Basavaraj, G., Rao, P. P., Bantilan, C., and Haldar, S. (2013). Sorghum and pearl millet economy of India: future outlook and options. Econ. Polit. Wkly. 48, 74–81.
Nainggolan, D., Abay, A. T., Christensen, J. H., and Termansen, M. (2023). The impact of climate change on crop mix shift in the Nordic region. Sci. Rep. 13:2962. doi: 10.1038/s41598-023-29249-w
Naoura, G., Emendack, Y., Sawadogo, N., Djirabaye, N., Tabo, R., Laza, H., et al. (2023). Assessment of photoperiod sensitivity and the effect of sowing date on dry-season Sorghum cultivars in southern Chad. Agronomy 13:932. doi: 10.3390/agronomy13030932
Ndlovu, E., van Staden, J., and Maphosa, M. (2021). Morpho-physiological effects of moisture, heat and combined stresses on Sorghum bicolor [Moench (L.)] and its acclimation mechanisms. Plant Stress 2:100018. doi: 10.1016/j.stress.2021.100018
Nokerbekova, N. K., Suleimenov, Y. T., and Zhapayev, R. K. (2018). Influence of fertilizing with nitrogen fertilizer on the content of amino acids in sweet Sorghum grain. Agricult. Food Sci. Res. 5, 64–67. doi: 10.20448/journal.512.2018.52.64.67
Obour, A. K., Holman, J. D., and Assefa, Y. (2022). Grain sorghum productivity as affected by nitrogen rates and available soil water. Crop Sci. 62, 1360–1372. doi: 10.1002/csc2.20731
Ogbaga, C. C., Stepien, P., Dyson, B. C., Rattray, N. J. W., Ellis, D. I., Goodacre, R., et al. (2016). Biochemical analyses of Sorghum varieties reveal differential responses to drought. PLoS One 11:e0154423. doi: 10.1371/journal.pone.0154423
Orchard, P. W., and Jessop, R. S. (1984). The response of sorghum and sunflower to short-term waterlogging. Plant Soil 81, 119–132. doi: 10.1007/BF02206901
Orchard, P. W., Jessop, R. S., and So, H. B. (1986). The response of sorghum and sunflower to short-term waterlogging. Plant Soil 91, 87–100. doi: 10.1007/BF02181821
Pal, U., Upadhyay, U., Singh, S., and Umrani, N. (1982). Mineral nutrition and fertilizer response of grain sorghum in India — a review over the last 25 years. Fertilizer Res. 3, 141–159. doi: 10.1007/BF01082974
Pardales, J. R., Kono, Y., and Yamauchi, A. (1991). Response of the different root system components of sorghum to incidence of waterlogging. Environ. Exp. Bot. 31, 107–115. doi: 10.1016/0098-8472(91)90013-E
Peñuelas, J., Prieto, P., Beier, C., Cesaraccio, C., and De ANGELIS, P. (2007). Response of plant species richness and primary productivity in shrublands along a north–south gradient in Europe to seven years of experimental warming and drought: reductions in primary productivity in the heat and drought year of 2003. Glob. Chang. Biol. 13, 2563–2581. doi: 10.1111/j.1365-2486.2007.01464.x
Perumal, R., Hays, A. R. C., Magill, U. C. W., Center, E., and Prom, U. L. K. (2018). Sorghum breeding for biotic stress tolerance Texas a&M University, USA; Gary C. Peterson, Texas a&M AgriLife research and university, USA, in: Achieving sustainable cultivation of Sorghum volume 1, Pa, USA: Burleigh Dodds Science Publishing.
Pfeiffer, B. K., Pietsch, D., Schnell, R. W., and Rooney, W. L. (2019). Long-term selection in hybrid Sorghum breeding programs. Crop Sci. 59, 150–164. doi: 10.2135/cropsci2018.05.0345
Ploschuk, R. A., Miralles, D. J., Colmer, T. D., Ploschuk, E. L., and Striker, G. G. (2018). Waterlogging of winter crops at early and late stages: impacts on leaf physiology, growth and yield. Front. Plant Sci. 9:1863. doi: 10.3389/fpls.2018.01863
Prasad, P. V. V., Boote, K. J., and Allen, L. H. (2006). Adverse high temperature effects on pollen viability, seed-set, seed yield and harvest index of grain-sorghum [Sorghum bicolor (L.) Moench] are more severe at elevated carbon dioxide due to higher tissue temperatures. Agric. For. Meteorol. 139, 237–251. doi: 10.1016/j.agrformet.2006.07.003
Prasad, P. V. V., Djanaguiraman, M., Jagadish, S. V. K., and Ciampitti, I. A. (2019). “Drought and high temperature stress and traits associated with tolerance” in Sorghum: A State of the Art and Future Perspectives (Madison, WI: ASA, CSSA, SSSA), 245–265.
Prasad, P. V. V., Djanaguiraman, M., Perumal, R., and Ciampitti, I. A. (2015). Impact of high temperature stress on floret fertility and individual grain weight of grain sorghum: sensitive stages and thresholds for temperature and duration. Front. Plant Sci. 6:820. doi: 10.3389/fpls.2015.00820
Prasad, V. B. R., Govindaraj, M., Djanaguiraman, M., Djalovic, I., Shailani, A., Rawat, N., et al. (2021). Drought and high temperature stress in Sorghum: physiological, genetic, and molecular insights and breeding approaches. Int. J. Mol. Sci. 22:9826. doi: 10.3390/ijms22189826
Prasad, P. V. V., Pisipati, S. R., Mutava, R. N., and Tuinstra, M. R. (2008). Sensitivity of grain Sorghum to high temperature stress during reproductive development. Crop Sci. 48, 1911–1917. doi: 10.2135/cropsci2008.01.0036
Promkhambut, A., Polthanee, A., Akkasaeng, C., and Younger, A. (2011). Growth, yield and aerenchyma formation of sweet and multipurpose sorghum (Sorghum bicolor L. Moench) as affected by flooding at different growth stages. Aust. J. Crop. Sci. 5, 954–965. doi: 10.1111/j.1439-0523.2010.01842.x
Purseglove, J. W. (1986). Tropical Crops-monocotyledons. Wiley, Harlow, Essex, England: New York, NY.
Quinn, L. D., Straker, K. C., Guo, J., Kim, S., Thapa, S., Kling, G., et al. (2015). Stress-tolerant feedstocks for sustainable bioenergy production on marginal land. Bioenergy Res. 8, 1081–1100. doi: 10.1007/s12155-014-9557-y
Rao, P., Basavaraj, G., Ahmad, W., and Bhagavatula, S. (2010). An analysis of availability and utilization of sorghum grain in India. SAT eJ 8.
Raza, A., Razzaq, A., Mehmood, S. S., Zou, X., Zhang, X., Lv, Y., et al. (2019). Impact of climate change on crops adaptation and strategies to tackle its outcome: a review. Plants (Basel) 8:34. doi: 10.3390/plants8020034
Rosegrant, M. W., and Msangi, S. (2014). Consensus and contention in the food-versus-fuel debate. Annu. Rev. Environ. Resour. 39, 271–294. doi: 10.1146/annurev-environ-031813-132233
Russell, M. B. (1959). “Water and its relation to soils and crops” in Advances in Agronomy. ed. A. G. Norman (USA: Academic Press), 1–131.
Salih, A. A., Ali, I. A., Lux, A., Luxová, M., Cohen, Y., Sugimoto, Y., et al. (1999). Rooting, water uptake, and xylem structure adaptation to drought of two Sorghum cultivars. Crop Sci. 39, 168–173. doi: 10.2135/cropsci1999.0011183X003900010027x
Sánchez, P. A. (2010). Tripling crop yields in tropical Africa. Nat. Geosci. 3, 299–300. doi: 10.1038/ngeo853
Sanders, J. H., Ouendeba, B., Ndoye, A., Témé, N., and Traore, S. (2019). Economics of increasing Sorghum productivity in sub-Saharan Africa: the Mali case. Methods Mol. Biol. 1931, 223–243. doi: 10.1007/978-1-4939-9039-9_16
Santamaria, J. M., Ludlow, M. M., and Fukai, S. (1990). Contribution of osmotic adjustment to grain yield in Sorghum bicolor (L.) Moench under water-limited conditions. I. Water stress before anthesis. Aust. J. Agric. Res. 41, 51–65. doi: 10.1071/ar9900051
Sarshad, A., Talei, D., Torabi, M., Rafiei, F., and Nejatkhah, P. (2021). Morphological and biochemical responses of Sorghum bicolor (L.) Moench under drought stress. SN. Appl. Sci. 3, 1–12. doi: 10.1007/s42452-020-03977-4
Sebnie, W., Mengesha, M., Girmay, G., Feyisa, T., Asgedom, B., Beza, G., et al. (2020). Evaluation of micro-dosing fertilizer application on sorghum (Sorghum bicholor L) production at wag-Lasta areas of Amhara region. Ethiopia. Sci Rep 10:6889. doi: 10.1038/s41598-020-63851-6
Seneviratne, S. I., Corti, T., Davin, E. L., Hirschi, M., Jaeger, E. B., Lehner, I., et al. (2010). Investigating soil moisture–climate interactions in a changing climate: a review. Earth Sci. Rev. 99, 125–161. doi: 10.1016/j.earscirev.2010.02.004
Sharma, J., and Ravindranath, N. H. (2019). Applying IPCC 2014 framework for hazard-specific vulnerability assessment under climate change. Environ. Res. Commun. 1:051004. doi: 10.1088/2515-7620/ab24ed
Shrestha, D. S., Staab, B. D., and Duffield, J. A. (2019). Biofuel impact on food prices index and land use change. Biomass Bioenergy 124, 43–53. doi: 10.1016/j.biombioe.2019.03.003
Srivastava, A., Naresh Kumar, S., and Aggarwal, P. K. (2010). Assessment on vulnerability of sorghum to climate change in India. Agric. Ecosyst. Environ. 138, 160–169. doi: 10.1016/j.agee.2010.04.012
Stein, K. (2007). Food vs biofuel. J. Am. Diet. Assoc. 107, 1870–1878. doi: 10.1016/j.jada.2007.09.014
Sultan, B., Roudier, P., Quirion, P., Alhassane, A., Muller, B., Dingkuhn, M., et al. (2013). Assessing climate change impacts on sorghum and millet yields in the Sudanian and Sahelian savannas of West Africa. Environ. Res. Lett. 8:014040. doi: 10.1088/1748-9326/8/1/014040
Tack, J., Lingenfelser, J., and Jagadish, S. V. K. (2017). Disaggregating sorghum yield reductions under warming scenarios exposes narrow genetic diversity in US breeding programs. PNAS 114, 9296–9301. doi: 10.1073/pnas.1706383114
Tari, I., Laskay, G., Takács, Z., and Poór, P. (2013). Response of Sorghum to abiotic stresses: a review. J. Agron. Crop Sci. 199, 264–274. doi: 10.1111/jac.12017
Tasie, M. M., and Gebreyes, B. G. (2020). Characterization of nutritional, Antinutritional, and mineral contents of thirty-five Sorghum varieties grown in Ethiopia. Int. J. Food Sci. 2020:e8243617, 1–11. doi: 10.1155/2020/8243617
Temeche, D., Getachew, E., and Hailu, G. (2021). Effects of nitrogen fertilizer quantity and time of application on Sorghum (Sorghum bicolor (L.) Moench) production in lowland areas of north Shewa, Ethiopia. Int. J. Agronomy 2021:e3019528. doi: 10.1155/2021/3019528
Tingem, M., Rivington, M., Bellocchi, G., Azam-Ali, S., and Colls, J. (2008). Effects of climate change on crop production in Cameroon. Clim. Res. 36, 65–77. doi: 10.3354/cr00733
Tingting, X., Peixi, S., and Lishan, S. (2010). Photosynthetic characteristics and water use efficiency of sweet sorghum under different watering regimes. Pak. J. Bot. :42, 3981–3994.
Turner, N. C. (2018). Turgor maintenance by osmotic adjustment: 40 years of progress. J. Exp. Bot. 69, 3223–3233. doi: 10.1093/jxb/ery181
Turner, N., and Jones, M. M. (1980). “Turgor maintenance by osmotic adjustment: a review and evaluation” in Book Chapter in Adaptation of Plants to Water and High Temperature Stress. USA: John Wiley & Sons, 58–86.
Upadhyaya, H. D., Vetriventhan, M., Asiri, A. M., and Azevedo, C. R. (2019). Multi-trait diverse germplasm sources from Mini Core collection for Sorghum improvement. Agriculture 9:121. doi: 10.3390/agriculture9060121
von Haden, A. C., Burnham, M. B., Yang, W. H., and DeLucia, E. H. (2021). Comparative establishment and yield of bioenergy sorghum and maize following pre-emergence waterlogging. Agron J. 113, 5602–5611. doi: 10.1002/agj2.20832
Wendmu, T. A., Cuni-Sanchez, A., Abebe, H. T., de Boer, H. J., Abera, F. A., and Westengen, O. T. (2022). Cultural effects on Sorghum varieties grown, traits preferred, and seed management practices in northern Ethiopia. Econ. Bot. 76, 233–249. doi: 10.1007/s12231-022-09555-6
Winchell, F., Stevens, C. J., Murphy, C., Champion, L., and Fuller, D. Q. (2017). Evidence for Sorghum domestication in fourth millennium BC eastern Sudan: spikelet morphology from ceramic impressions of the Butana group. Curr. Anthropol. 58, 673–683. doi: 10.1086/693898
Younis, M. E., El-Shahaby, O. A., Abo-Hamed, S. A., and Ibrahim, A. H. (2000). Effects of water stress on growth, pigments and 14CO2 assimilation in three Sorghum cultivars. J. Agron. Crop Sci. 185, 73–82. doi: 10.1046/j.1439-037x.2000.00400.x
Yu, S.-M., Lo, S.-F., and Ho, T.-H. D. (2015). Source–sink communication: regulated by hormone, nutrient, and stress cross-signaling. Trends Plant Sci. 20, 844–857. doi: 10.1016/j.tplants.2015.10.009
Zamani, A., Emam, Y., Pessarakli, M., and Shakeri, E. (2020). Growth and biochemical responses of sorghum genotypes to nitrogen fertilizer under salinity stress conditions. J. Plant Nutr. 44, 569–579. doi: 10.1080/01904167.2020.1845379
Zandalinas, S. I., Sales, C., Beltrán, J., Gómez-Cadenas, A., and Arbona, V. (2017). Activation of secondary metabolism in Citrus plants is associated to sensitivity to combined drought and high temperatures. Front. Plant Sci. 7:1954. doi: 10.3389/fpls.2016.01954
Zander, A., Lofton, J., Harris, C., and Kezar, S. (2021). Grain sorghum production: influence of planting date, hybrid selection, and insecticide application. Agrosyst. Geosci. Environ. 4:e20162. doi: 10.1002/agg2.20162
Zhang, J., and Kirkham, M. B. (2000). Hydraulic resistance of Sorghum (C4) and sunflower (C3). J. Crop. Prod. 2, 287–298. doi: 10.1300/J144v02n02_12
Zhang, F., Wang, Y., Yu, H., Zhu, K., Zhang, Z., and Zou, F. L. J. (2016). Effect of excessive soil moisture stress on sweet Sorghum: physiological changes and productivity. Pak. J. Bot. 10, 1–10. doi: 10.1371/journal.pone.0140620
Zhang, R., Zhou, Y., Yue, Z., Chen, X., Cao, X., Ai, X., et al. (2019). The leaf-air temperature difference reflects the variation in water status and photosynthesis of sorghum under waterlogged conditions. PLoS One 14:e0219209. doi: 10.1371/journal.pone.0219209
Keywords: sorghum, tolerance, heat stress, drought, waterlogging, food security, climate change
Citation: Khalifa M and Eltahir EAB (2023) Assessment of global sorghum production, tolerance, and climate risk. Front. Sustain. Food Syst. 7:1184373. doi: 10.3389/fsufs.2023.1184373
Edited by:
Dengpan Xiao, Hebei Normal University, ChinaReviewed by:
Cristina Patanè, National Research Council (CNR), ItalyDandan Ren, Hebei Normal University, China
Copyright © 2023 Khalifa and Eltahir. This is an open-access article distributed under the terms of the Creative Commons Attribution License (CC BY). The use, distribution or reproduction in other forums is permitted, provided the original author(s) and the copyright owner(s) are credited and that the original publication in this journal is cited, in accordance with accepted academic practice. No use, distribution or reproduction is permitted which does not comply with these terms.
*Correspondence: Muhammad Khalifa, khalifa@mit.edu; msakhalifa@hotmail.com