A specific reverse complement sequence for distinguishing Brucella canis from other Brucella species
- 1Key Laboratory of Livestock Infectious Diseases, Ministry of Education, Shenyang Agricultural University, Shenyang, China
- 2Beijing Animal Disease Prevention and Control Center, Beijing, China
- 3Division of the Standards, China Institute of Veterinary Drug Control, Beijing, China
Canine brucellosis is primarily caused by Brucella canis, but other Brucella species can also cause the disease. Identifying sequences specific to B. canis and establishing PCR assays that can distinguish between B. canis and other Brucella species is essential to determine the etiology of canine brucellosis and the source of infection and to achieve effective control. We analyzed the gaps and SNPs of genomes I and II from B. canis strain RM6/66 and B. melitensis strain 16M using the Mauve genome alignment software, and the specificity of each of these differential regions was analyzed by BLAST. A 132 bp specific sequence was found between the DK60_915 (glycosyl hydrolase 108 family protein) and DK60_917 (aldose 1-epimerase) loci in B. canis chromosome 1. Further comparative analysis revealed that this is a reverse complement sequence between B. canis and other Brucella species. Then, three primers were designed based on the sequence that could detect B. canis with a 310 bp amplification product or other Brucella species with a 413 bp product. The PCR based on these primers had reasonable specificity and a sensitivity of 100 copies of Brucella DNA. The detection results for the blood samples of the aborted dogs showed a favorable accordance with the Bruce-ladder multiplex PCR assay. In conclusion, we found a specific reverse complement sequence between B. canis and other Brucella and developed a PCR method that allows a more comprehensive identification of the pathogen involved in canine brucellosis. These findings provide an effective means for preventing and controlling brucellosis.
Introduction
Brucellosis is a zoonotic disease caused by Brucella species. The number of species in this genus has been gradually expanded by the discovery of strains from wildlife animal species, such as amphibians and fish (1). Four major pathogenic Brucella species causing disease in humans are Brucella abortus (cattle, buffalo), Brucella melitensis (goats, sheep, camels), Brucella suis (pigs), and Brucella canis (dogs) (2). Currently, more than 500 000 new brucellosis cases are annually diagnosed worldwide (3).
Dogs come into frequent contact with people and can be infected with Brucella. The main pathogen in dogs is B. canis, but other Brucella species can also cause infection or disease (4–6). B. canis can be transmitted to humans by infected dogs or their secretions. Unlike other Brucella species, the infection symptoms of B. canis are absent or mild (7). However, endocarditis or meningitis may develop in some cases (8). Of the other Brucella species, B. abortus and B. melitensis are more pathogenic to humans. Thus, infection of humans by Brucella from dogs remains a concern, especially considering that the number of dogs used as pets is significantly increasing.
Isolation from culture is the most accurate form of brucellosis detection, and species identification is conducted by the amino sugar quinovosamine assay (9). However, these methods are time-consuming and need to be performed in a biosafety level III laboratory. Furthermore, the effectiveness is affected by the bacterial load levels in the blood of the infected animal (10). On the contrary, molecular biological detection technologies are safe, reliable, highly sensitive, and strongly specific and require a simple operation. Accordingly, they have been popularized and applied for the detection of Brucella (11, 12).
At present, there are a variety of PCR methods for detecting B. canis (13, 14), such as the Bruce-ladder (14) and multilocus variable-number tandem-repeat (VNTR) analysis (MLVA) procedures (15). However, most of them distinguish B. canis by multiple amplification products; hence, significant efforts are needed to recognize the amplified bands of each Brucella species. More importantly, they need high-quality genomic DNA in great concentrations, limiting their use for clinical specimens (16). In 2014, Kang established a PCR method for the specific detection of B. canis based on a 12 bp deletion in the BCAN_B0548 region (530056 site) of chromosome II in B. canis ATCC 23365 (16). However, it cannot detect other Brucella species and probably omits brucellosis caused by other Brucella species (17). Using this diagnostic method to test dogs and their owners for Brucella may overlook other Brucella species; therefore, it is essential to establish a test that can detect both B. canis and other Brucella species, that can be used to make inferences about the origin of Brucella infection in dogs and their owners, and that can be used for prevention and control treatment.
In this study, a specific 132 bp sequence of B. canis was found by comparing the genomes of B. canis and B. melitensis and conducting BLAST alignment of the single nucleotide polymorphisms (SNPs) and indels in GeneBank. A reverse complementary was found between the sequence in B. canis and that in other Brucella species. Based on this sequence, we developed a multiplex PCR with three primers that can specifically distinguish B. canis from other Brucella species. This method may be very helpful in preventing and controlling brucellosis and reducing human infection.
Materials and methods
Genome alignment of the B. canis strain RM6/66 and B. melitensis strain 16M
The genome sequences of B. canis strain RM6/66 and B. melitensis biovar 1. 16M were downloaded from the NCBI database and analyzed by the multiple genome alignment software Mauve 20150226 (The Darling lab at the University of Technology Sydney). The lists of gaps and SNPs from the alignment results of chromosomes 1 and 2 were exported.
Screening of the specific sequence of B. canis
The gaps of both B. canis and B. melitensis genomes were compared using BLAST for the specific differential sequences of B. canis. The candidate differential sequences were aligned by the DNAMAN 7 software (LynnonBiosoft, CA, USA).
Primer design
Based on the candidate differential sequences, primers were designed by the Primer-BLAST program on the NCBI website to distinguish B. canis from other Brucella species. Appropriate adjustments were made to obtain more specific primers. The designed primers were synthesized by Sangon Biotech Co., Ltd (Shanghai, China).
Strains and DNA extraction
All the strains used in this experiment are listed in Table 1. The DNA of B. abortus strain 2308, B. melitensis biovar 1 strain16M, and B. canis strain RM6/66 strains was donated by the China Institute of Veterinary Drug Control. The B. melitensis strain M5, B. abortus strain A19, and B. suis strain S2 were purchased from Tecon Biology Co. Ltd, Xinjiang. The canine Vanguard® Plus 5-CVL and feline Fel-O-Vax® PCT vaccines (Zoetis, NJ, USA) were purchased from a local pet hospital. Other common bacterial strains are preserved in this laboratory. According to the manufacturer's instructions, genomic DNA was extracted using a MiniBEST Bacteria Genomic DNA Extraction Kit (Takara, Dalian, China). Then, the quantity or quality of the extracted DNA was measured with an ultraviolet spectrophotometer, and the copies of the genome were counted online (http://scienceprimer.com/copy-number-calculator-for-realtime-pcr) based on the concentration of DNA and the base number of the Brucella genome. The extracted DNA was stored at −20°C for further use. A total of 86 canine blood samples were collected at the pet hospitals in Shenyang. DNA was extracted from the samples using a MiniBEST Whole Blood Genomic DNA Extraction Kit (Takara, Dalian, China) and stored at −20°C. Taq PCR MasterMix was purchased from Vazyme Biotech Co., Ltd, Nanjing, China.
Optimization of the PCR amplification conditions
For the PCR reaction, 10 μl 2× Taq PCR MasterMix, 1 μl of each primer (10 μM), and 6 μl water were mixed in a 200-μl PCR tube. Then, 1 μl of Brucella DNA template or 1 μl of distilled water was added as a template or negative control, respectively. The PCR amplification conditions were as follows: pre-denaturation at 94°C for 5 min, 35 cycles at 95°C for 15 s, 60°C for 15 s, and 72°C for 30 s, followed by a final extension at 72°C for 10 min. The PCR amplification products were visualized by electrophoresis on 1.5% agarose gels.
For better amplification conditions, the PCR reaction was optimized by annealing at different temperatures (60°C, 64°C, 68°C, and 72°C). The optimum annealing temperature was determined according to the stray band's existence and the amplified band's brightness.
Specificity and sensitivity of the assay
The genomic DNA template of B. canis strain RM6/66 and B. melitensis strain 16M was diluted from 103 to 1 copies/μl with sterilized distilled water. Then, 1 μl diluted DNA was used as the template in the PCR amplification. The PCR reaction contained 103-1 copies of DNA of the Brucella gene. Electrophoresis was performed to identify the PCR product. Then, 20 replicates of the DNA at the point of analytical sensitivity were detected in five separate experiments for confirmation.
To verify the specificity, DNAs from other Brucella strains and non-Brucella bacteria (Table 1) were used as templates for PCR amplification under the optimized PCR conditions. The amplification products were analyzed on a 1.5% electrophoresis gel and observed under ultraviolet light.
Repeatability test
PCR repeatability experiments were carried out with 104 to 102 copies/μl of the genomic DNA of B. canis strain RM6/66 as the template. In the intra-batch repeatability test, the above three samples were tested three times under the same reaction conditions, with three replicates for each sample. In the inter-batch repeatability test, the four samples were tested three times at 1-day intervals, and three replicates were set up for each sample. The amplification products were photographed using the OmegaLumG Gel imaging system (Aplegen Inc, CA, USA) after electrophoresis, and the integrated density values of the bands were calculated using ImageJ 1.52p software (NIH, USA). Then, the mean, standard deviation, and coefficient of variation were calculated to evaluate the repeatability of the PCR.
Detection of the clinical samples
To evaluate the clinical efficacy of the established PCR method, we collected whole blood samples of aborted dogs in Shenyang, China. DNA was extracted from blood samples of 300 aborted dogs using a MiniBEST Bacteria Genomic DNA Extraction Kit. These DNAs were examined using the established PCR, and the results were compared with those of the Bruce-Ladder multiplex PCR assay (14).
Results
Genome analysis
The genome sequences of B. canis strain RM6/66 (NZ_CP007758.1, NZ_CP007759.1) and B. melitensis biovar 1 strain 16M (NZ_CP007763.1, NZ_CP007762.1) were downloaded from the NCBI database. Their chromosome I (NZ_CP007758.1, NZ_CP007763.1) and II (NZ_CP007759.1, NZ_CP007762.1) sequences were analyzed by the multiple genome alignment software Mauve. Compared with those of B. melitensis strain 16M, chromosomes I and II of B. canis strain RM6/66 had gene rearrangement (Supplementary Figures 1A,B), and the frequency of chromosome II rearrangement was higher than that of chromosome I.
Aligned gaps and SNPs between B. canis and B. melitensis were exported from the mauve program (listed in Supplementary Table). There were 204 gaps, 231 inserts, and 5143 SNPs in chromosome I, and 140 gaps, 169 inserts, and 3113 SNPs in chromosome II (Table 2; Supplementary Figure 1C), illustrating that chromosome 1 is more diverse than chromosome 2.

Table 2. Insertions and deletions in the B.melitensis strain 16M genome compared to the B. canis strain 6/66 genome.
Screening of the specific sequence of B. canis
A BLAST alignment of the gaps and the adjacent sequence of B. canis strain RM6/66 showed that a 132 bp gap at the 943403 site on chromosome 1 has reasonable specificity, only having a high similarity and a high score with B. canis (Figure 1A). In Mauve, it corresponds to a gap of the same size at the 303367 site on B. melitensis chromosome 1 (Figure 1B). Then DNAMAN was used to compare the gaps between the two strains. When the gap with the flanking sequences was aligned, the gap sequence identity between both genomes was low (84.49%; Figure 1C). However, both gap sequences were found to be reversely complementary (Figure 1D). These findings suggest that this sequence is characteristic of B. canis and that it can be potentially used for B. canis identification. Further analysis showed that it is located in the noncoding region between the DK60_915 (glycosyl hydrolase 108 family protein) and DK60_917 (aldose 1-epimerase) loci.
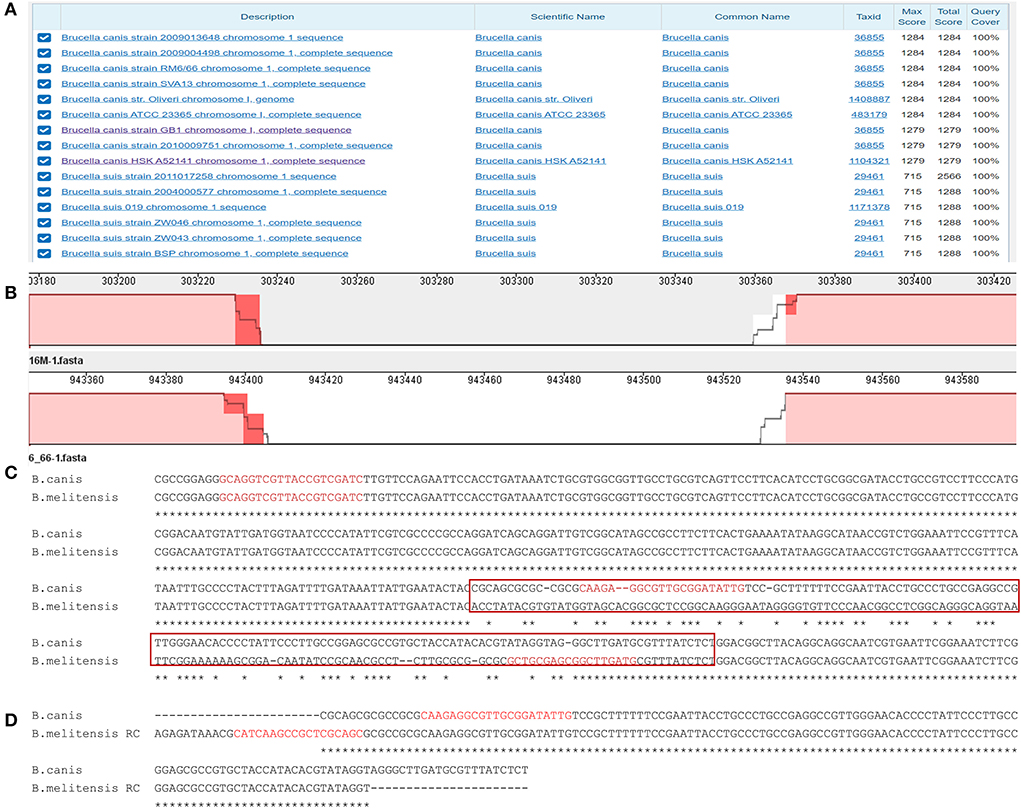
Figure 1. The analysis of the specific sequence in B. canis. (A) BLAST result of the specific sequence. (B) The region of the specific sequence in the genome alignment. (C) The alignment of the specific sequences in B. canis and the corresponding sequence in B. melitensis. The red box represents the sequences in (D). The red sequences represent the designed primers. (D) The alignment of the specific sequence in B. canis and the corresponding reverse complement sequence in B. melitensis.
Primer design
Three primers (Table 3) were designed based on the differential region. To avoid amplification between two downstream primers, a downstream primer (BSD) used for amplifying other Brucella species was selected at the junction of the specific and consensus sequences. The position of each primer is shown in Figure 1C. The sizes of the amplicons of these three primers were 310 bp and 413 bp for B. canis and other Brucella species, respectively.
Optimization of the PCR amplification conditions
To optimize amplification, different annealing temperatures were tested. PCR bands were visible at annealing temperatures ranging from 60°C to 68°C (Figure 2A). However, both amplification products using B. canis and B. melitensis as templates resulted in bright bands and no evident spurious bands when a 64°C temperature was used. Therefore, 64°C was used as the annealing temperature in the subsequent specificity and sensitivity tests.
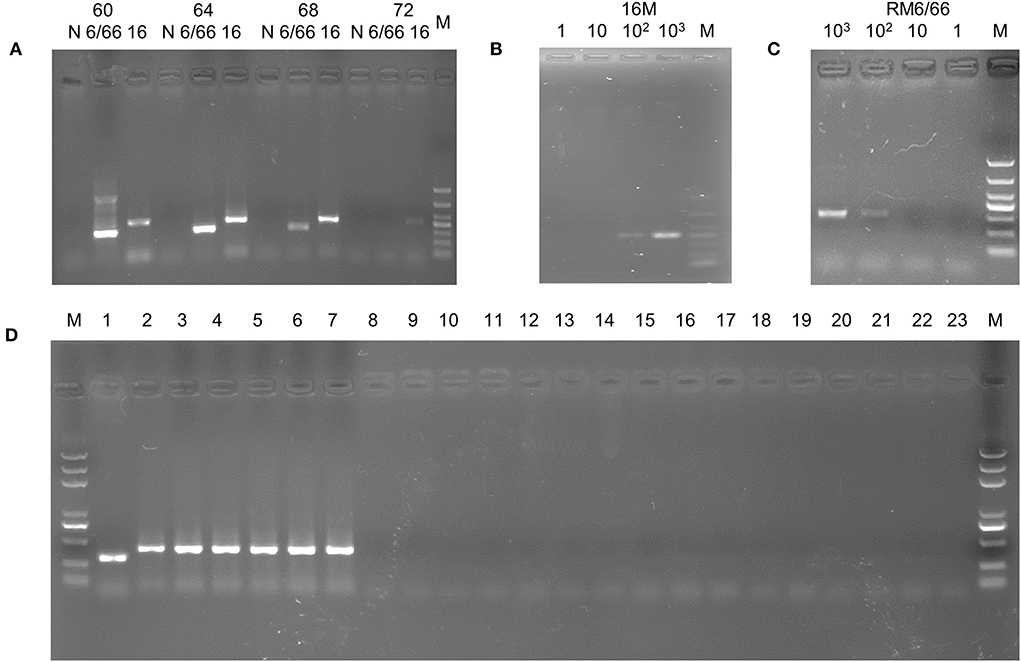
Figure 2. PCR assay based on the specific sequence. (A) Annealing temperature optimization. M, DL1000 DNA Marker; 6/66: B. canis strain RM6/66; 16: B.melitensis strain 16M. (B) Sensitivity assay of the B.melitensis strain 16M template. M, DL1000 DNA Marker. (C) Sensitivity assay of the B. canis strain 6/66 template. M, DL1000 DNA Marker. (D) Specificity assay. M, DL2000 plus DNA Marker; 1, B. canis strian RM6/66; 2, B. abortus strain A19; 3, B. suis strain S2; 4, B. melitensis strain M5; 5, B. abortus strain 2308; 6, B. suis strain 1330; 7, B. melitensis strain 16M; 8, Vanguard® Plus 5-CVL vaccine; 9, Fel-O-Vax® PCT vaccine (Zoetis, USA); 10, E. coli; 11, Salmonella enteritidis; 12, Shigella dysenteriae; 13, Pasteurella multocida; 14, Streptococcus hemolyticus; 15, Clostridium perfringens type C;16, Staphylococcus aureus; 17, Proteus mirabillis; 18, Candida albicans; 19, Streptococcus pyogenes; 20, Streptococcus pneumoniae; 20, Campylobacter jejuni; 21, Listeria monocytogenes; 22, Pseudomonas aeruginosa; 23, negative control.
The sensitivity of the assay
To determine the sensitivity of the PCR method, we performed gradient dilution of the DNA from B. canis strain RM6/66 and B. melitensis strain 16M. The reaction solutions with 103-102 copies of DNA resulted in PCR amplification (Figures 2B,C), indicating that the established PCR assay could detect a minimum of 100 copies of Brucella DNA. In the subsequent repetitive detection of DNA samples at sensitive sites, all samples were amplified with visible bands of interest (100%).
The specificity of the assay
Using DNA of B. canis strain RM6/66, B. abortus strain A19, B. abortus wild type 2308, B. suis strain S2, B. suis strian 1330, B. melitensis strain M5, B. melitensis strain 16M, and other non-Brucella bacteria (Table 1) as a template, the PCR assay was conducted with the optimal annealing temperature (64°C). The amplicons of B. canis DNA and other Brucella DNA appeared as distinct 310 bp and 413 bp bands on agarose gels, respectively (Figure 2D). At the same time, no band was amplified when non-Brucella DNA was used as a template. These results suggest excellent specificity of the established PCR, demonstrating that the method can detect Brucella and distinguish B. canis from other Brucella species.
Repeatability test results
DNA from three different dilutions of B. canis strain RM6/66 was used to perform intra- and inter-batch reproducibility tests. The integrated gray values of the 400 bp band in the marker and amplification product band were measured using ImageJ software. The number of amplified bands was expressed by the grayscale ratio of amplified bands to 400 bp bands. The mean, standard deviation, and coefficient of variation of three repeated measurements were calculated (Table 4). The coefficients of variation of both intra- and inter-batch reproducibility tests were lower than 10%, indicating that the established PCR method was reproducible.
Detection of the clinical samples
To validate the efficacy of the PCR assay, the DNA of blood samples from the aborted dogs was tested by the developed PCR and the Bruce-ladder multiplex PCR assay (14). The developed PCR detected 22 positive samples with a prevalence of 7.33% (22/300). The prevalence rate for B. canis and other Brucella was 6.33% (19/300) and 1.00% (3/300), respectively (Table 5). The Bruce-ladder multiplex PCR assay detected 21 positive samples with a prevalence rate of 7.0% (21/300), in which the prevalence for B. canis, B. melitensis, and B. abortus was 6.0% (18/300), 0.67% (2/300), and 0.33% (1/300), respectively (Table 5). The accordance of the two methods for B. canis and other Brucella was 94.7% (18/19) and 100% (3/3), respectively. There was no statistical difference between the two methods. These results indicated that the developed assay accurately distinguishes B. canis from other Brucella species.
Discussion
The aim of this study was to find a unique sequence to distinguish between B. canis and other Brucella species for rapidly detecting canine brucellosis. The designed PCR based on the different sequences demonstrated good specificity, sensitivity, and repeatability. The assay can not only detect brucellosis but can also distinguish B. canis from other types of Brucella. As a result, it can be applied to the diagnosis of canine brucellosis.
With the development of sequencing, databases, and networking technologies, a vast amount of genomic and sequence data is now available at individual terminals. Making good use of these data has become essential to improving research efficiency. Desktop or web-based bioinformatics software addresses this issue. Multiple Genome Alignment Software Mauve can quickly analyze rearrangements, insertions, deletions, and changes between genome sequences and determine the differences between genes from a macro and micro perspective (18, 19). BLAST is a general alignment procedure that compares sequences similar to target sequences in Genebank databases to elucidate the specificity of target sequences (20). Primer-Blast is a procedure for primer design and comparison that can effectively analyze the specificity of primers (21). The combined use of this software can effectively improve the specificity and success rate of PCR assays, which are widely used (22). In this study, we went through that process and satisfactory results were obtained. First, we found a 132 bp reverse complement sequence between B. canis and other Brucella species.
Molecular biological detection techniques for distinguishing Brucella species such as AMOS-PCR (23, 24) and Bruce-ladder (14, 25) have been established. However, it takes a long time to recognize each Brucella species' amplicons. Furthermore, these techniques require high-quality genomic DNA in great concentrations, which limits their use for clinical specimens (16). A PCR method for the specific detection of B. canis was established in 2014 based on the 12 bp deletion of chromosome II in B. canis ATCC 23365, which had a detection limit of 3 × 103 colony-forming units (16). However, brucellosis in dogs can be caused by other Brucella species (4–6); therefore, infection by these species may pass unidentified by this PCR method. This study established a multiplex PCR using three primers for the specific sequence to simultaneously detect B. canis and other Brucella species without amplifying other non-Brucella bacteria. Its detection limit was 100 copies of the Brucella genome, which is higher than that of the abovementioned PCR method. In detecting the blood samples of the aborted dogs, the developed PCR assay demonstrated favorable accordance with the Bruce-ladder multiplex PCR assay.
B. canis is the main pathogen causing canine brucellosis. However, other common Brucella species, such as B. suis, B. abortus, and B. melitensis, can also cause it (17). Unlike B. canis, they are highly pathogenic to humans. Therefore, people that have had contact with infected dogs are at an increased risk. Consequently, it is necessary to strengthen the diagnosis of brucellosis. The method established in this study can detect the causative agent of canine brucellosis directly, quickly, and comprehensively. However, drawbacks still exist. This PCR cannot distinguish infection caused by a common vaccine strain, and additional tests must be performed to rule this out.
Conclusion
Here, we found a specific reverse complement sequence between B. canis and other Brucella species. A multiplex PCR detection method was developed to distinguish Brucella species, which can be used for the prevention and control of canine brucellosis and to reduce human brucellosis.
Data availability statement
The original contributions presented in the study are included in the article/Supplementary material, further inquiries can be directed to the corresponding authors.
Ethics statement
The animal study was reviewed and approved by Institutional Animal Care and Use Committee of Shenyang Agricultural University. Written informed consent was obtained from the owners for the participation of their animals in this study.
Author contributions
B-SL conceived the study. Z-LC directed the study. D-LL, L-HY, and L-HH analyzed the data. Y-BY, J-HY, ZZ, and S-YM performed the PCR assays. HZ and F-YD wrote the manuscript. All authors contributed to the article and approved the submitted version.
Funding
This study was supported by the State Key Program of National Natural Science of China [U1808202], NSFC International (regional) cooperation and exchange program [31961143024], and Major science and technology projects of Inner Mongolia of China.
Acknowledgments
We thank Editage (www.editage.cn) for English language editing.
Conflict of interest
The authors declare that the research was conducted in the absence of any commercial or financial relationships that could be construed as a potential conflict of interest.
Publisher's note
All claims expressed in this article are solely those of the authors and do not necessarily represent those of their affiliated organizations, or those of the publisher, the editors and the reviewers. Any product that may be evaluated in this article, or claim that may be made by its manufacturer, is not guaranteed or endorsed by the publisher.
Supplementary material
The Supplementary Material for this article can be found online at: https://www.frontiersin.org/articles/10.3389/fvets.2022.983482/full#supplementary-material
References
1. Cloeckaert A, Vergnaud G, Zygmunt MS. Omp2b porin alteration in the course of evolution of Brucella spp. Front Microbiol. (2020) 11:284. doi: 10.3389/fmicb.2020.00284
2. Kyebambe PS. Acute brucella meningomyeloencephalo-spondylosis in a teenage male. Afr Health Sci. (2005)5:69–72.
3. Pisarenko SV, Kovalev DA, Volynkina AS, Ponomarenko DG, Rusanova DV, Zharinova NV, et al. Global evolution and phylogeography of Brucella melitensis strains. BMC Genom. (2018) 19:353. doi: 10.1186/s12864-018-4762-2
4. Mor SM, Wiethoelter AK, Lee A, Moloney B, James DR, Malik R. Emergence of Brucella suis in dogs in New South Wales, Australia: clinical findings and implications for zoonotic transmission. BMC Vet Res. (2016) 12:199–199. doi: 10.1186/s12917-016-0835-0
5. Alamian S, Dadar M. Brucella melitensis infection in dog: a critical issue in the control of brucellosis in ruminant farms. Comp Immunol Microbiol Infect Dis. (2020) 73:101554. doi: 10.1016/j.cimid.2020.101554
6. Wareth G, Melzer F, El-Diasty M, Schmoock G, Elbauomy E, Abdel-Hamid N, et al. Isolation of Brucella abortus from a Dog and a Cat Confirms their Biological Role in Re-emergence and Dissemination of Bovine Brucellosis on Dairy Farms. Transbound Emerg Dis. (2017) 64:e27–27e30–30 doi: 10.1111/tbed.12535
7. Yüksekkaya S, Aras Z, Uçan US. Investigation of Brucella canis Seroprevalence in Brucellosis Suspected Cases. Mikrobiyol Bul. (2013) 47:152-7. doi: 10.5578/mb.4417
8. Sayan M, Erdenlig S, Etiler N. Investigation of Brucella canis seropositivity by in-house slide agglutination test antigen in healthy blood donors. Mikrobiyol Bul. (2011) 45:655–63.
9. Ohishi K, Abe E, Amano M, Miyazaki N, Boltunov A, Katsumata E, et al. Detection of serum antibodies to Brucella in Russian aquatic mammals. J Vet Med Sci. (2018) 80:1696–701. doi: 10.1292/jvms.18-0330
10. Camargo-Castañeda AM, Stranahan LW, Edwards JF, Garcia-Gonzalez DG, Roa L, Avila-Granados LM, et al. Characterization of epididymal and testicular histologic lesions and use of immunohistochemistry and PCR on formalin-fixed tissues to detect Brucella canis in male dogs. J Vet Diagn Invest. (2021) 33:352–6. doi: 10.1177/1040638720986883
11. Ren H, Yang M, Zhang G, Liu S, Wang X, Ke Y, et al. Development of a rapid recombinase polymerase amplification assay for detection of Brucella in blood samples. Mol Cell Probes. (2016) 30:122–4. doi: 10.1016/j.mcp.2016.02.007
12. Bhat IA, Mashooq M, Kumar D, Varshney R, Rathore R. Development of probe based real time loop mediated isothermal amplification for detection of Brucella. J Appl Microbiol. (2018) 126:1332–9. doi: 10.1111/jam.13938
13. Hinić V, Brodard I, Thomann A, Cvetnić Z, Makaya PV, Frey J, et al. Novel identification and differentiation of Brucella melitensis, B. abortus, B suis, B ovis, B canis, and B neotomae suitable for both conventional and real-time PCR systems. J Microbiol Methods. (2008) 75:375–8. doi: 10.1016/j.mimet.2008.07.002
14. López-Goñi I, García-Yoldi D, Marín CM, de Miguel MJ, Barquero-Calvo E, Guzmán-Verri C, et al. New Bruce-ladder multiplex PCR assay for the biovar typing of Brucella suis and the discrimination of Brucella suis and Brucella canis. Vet Microbiol. (2011) 154:152–5. doi: 10.1016/j.vetmic.2011.06.035
15. Daugaliyeva A, Sultanov A, Usserbayev B, Baramova S, Modesto P, Adambayeva A, et al. Genotyping of Brucella melitensis and Brucella abortus strains in Kazakhstan using MLVA-15. Infect Genet Evol. (2018) 58:135–44. doi: 10.1016/j.meegid.2017.12.022
16. Kang SI, Lee SE, Kim JY, Lee K, Kim JW, Lee HK, et al. A new Brucella canis species-specific PCR assay for the diagnosis of canine brucellosis. Comp Immunol Microbiol Infect Dis. (2014) 37:237–41. doi: 10.1016/j.cimid.2014.07.003
17. Philippon A, Roumy B, Renoux G. A case of canine brucellosis due to Brucella abortus. Bull Acad Vet Fr. (1969) 42:923–8. doi: 10.4267/2042/66917
18. Darling AC, Mau B, Blattner FR, Perna NT. Mauve: multiple alignment of conserved genomic sequence with rearrangements. Genome Res. (2004) 14:1394–403. doi: 10.1101/gr.2289704
19. Rissman AI, Mau B, Biehl BS, Darling AE, Glasner JD, Perna NT. Reordering contigs of draft genomes using the Mauve aligner. Bioinformatics. (2009) 25:2071–3. doi: 10.1093/bioinformatics/btp356
20. Camacho C, Coulouris G, Avagyan V, Ma N, Papadopoulos J, Bealer K, et al. BLAST+: architecture and applications. BMC Bioinformatics. (2009) 10:421. doi: 10.1186/1471-2105-10-421
21. Ye J, Coulouris G, Zaretskaya I, Cutcutache I, Rozen S, Madden TL. Primer-BLAST: a tool to design target-specific primers for polymerase chain reaction. BMC Bioinformatics. (2012) 13:134. doi: 10.1186/1471-2105-13-134
22. Baoshan L, Yinbo Y, Jingbo Z, Yi Z, Jianghua Y, Dawei C, et al. Combined nucleic acid assays for diagnosis of A19 vaccine-caused human brucellosis. Transbound Emerg Dis. (2021) 68:368–74. doi: 10.1111/tbed.13685
23. Matope G, Bhebhe E, Muma JB, Skjerve E, Djønne B. Characterization of some Brucella species from Zimbabwe by biochemical profiling and AMOS-PCR. BMC Res Notes. (2009) 2:261–261. doi: 10.1186/1756-0500-2-261
24. Bricker BJ, Halling SM. Enhancement of the Brucella AMOS PCR assay for differentiation of Brucella abortus vaccine strains S19 and RB51. J Clin Microbiol. (1995) 33:1640–2. doi: 10.1128/jcm.33.6.1640-1642.1995
Keywords: canine brucellosis, B. canis, reverse complementary sequence, canine, specific
Citation: Ye Y-B, Yang J-H, Li D-L, Hao L-H, Zhang Z, Mei S-Y, Zhang H, Du F-Y, Yv L-H, Liu B-S and Chen Z-L (2022) A specific reverse complement sequence for distinguishing Brucella canis from other Brucella species. Front. Vet. Sci. 9:983482. doi: 10.3389/fvets.2022.983482
Received: 01 July 2022; Accepted: 07 October 2022;
Published: 04 November 2022.
Edited by:
Dirk Werling, Royal Veterinary College (RVC), United KingdomReviewed by:
Kun Li, Nanjing Agricultural University, ChinaLaxmi Narayan Sarangi, National Dairy Development Board, India
Tariq Jamil, Friedrich Loeffler Institut, Germany
Copyright © 2022 Ye, Yang, Li, Hao, Zhang, Mei, Zhang, Du, Yv, Liu and Chen. This is an open-access article distributed under the terms of the Creative Commons Attribution License (CC BY). The use, distribution or reproduction in other forums is permitted, provided the original author(s) and the copyright owner(s) are credited and that the original publication in this journal is cited, in accordance with accepted academic practice. No use, distribution or reproduction is permitted which does not comply with these terms.
*Correspondence: Bao-Shan Liu, lbslgy@syau.edu.cn; Ze-Liang Chen, zeliangchen@yahoo.com
†These authors have contributed equally to this work