Introduction
Materials and Methods
Plant Material and Growth Condition
Treatments
Measurements
Fresh Weight and Dry Weight Measurements
Tipburn Index
Ca2+ Content in Leaves and Roots and its Absorption Rate
Ca2+ Content in Different Leaf Layers
Root Ca2+ Energy Spectrum Analysis
Chlorophyll Content
Fv/Fm Measurement
Results
Plant Growth and Tipburn Index
Ca2+ Content in Leaves and Roots and the Ca2+ Absorption Rate
Ca2+ Energy Spectrum Distribution in the Root Meristematic Zone and the Root Hair Zone
Ca2+ Content in Different Leaf Layers
Chlorophyll Content and Fv/Fm
Discussion
Introduction
Lettuce (Lactuca sativa L.) is an annual or biennial herbaceous plant widely grown around the world (Chu et al., 2002). It is one of the main horticultural crops because of its short growth cycle and easy planting. However, a physiological disorder, called tipburn, commonly occurs in lettuce production, particularly in greenhouse cultivation and in hot seasons. Early symptoms of tipburn appear as browning on the edges of leaves, especially on young leaves. Under high humidity and high temperatures, the symptomatic leaves gradually rot, reducing yield, appearance, and quality.
Tipburn is mainly caused by insufficient levels of available calcium (Ca) in the growing medium or poor uptake of Ca through plant roots (Collier and Tibbits, 1982; Olle and Bender, 2009). Common methods to prevent tipburn are Ca supplementation and promoting absorption and utilization of Ca
within the plant. Cresswell (1991) reported that spraying 100 mg·L-1 of calcium nitrate on head lettuce leaves could prevent or reduce the incidence of tipburn without influencing fresh weight. Applications of other exogenous substances have also been shown to prevent tipburn in vegetables by promoting Ca absorption and utilization. He et al. (2017) reported that a foliar application of calcium ascorbate promoted Ca absorption and reduced the incidence of tipburn in hydroponic lettuce. Wen et al. (1991) found that a foliar application of 0.7% calcium chloride dihydrate and 50 ppm naphthalene acetic acid increased the absorption of Ca and promoted the transportation of Ca2+ to young leaves in Chinese cabbage (Brassica rapa subsp. pekinensis), resulting in a reduced occurrence of tipburn. However, in these studies, foliar applications of Ca did not improve Ca uptake by plant roots. Some other methods that have been suggested to reduce tipburn include: improvement of the ion balance in the nutrient solution (Ashkar and Ries, 1971), blowing air onto the meristem (Frantz, et al., 2004) or onto the inner leaves (Goto and Takakura, 1992), shortening the day/night cycle (Goto and Takakura, 2003), or adjusting the light intensity for indoor cultivated lettuce (Sago, 2016). Nevertheless, tipburn is induced by a number of factors (e.g., growth rate, ion balance in the nutrient solution, stress, etc.) and the above methods are not always practical in greenhouse lettuce cultivation. Tipburn can also occur despite a sufficient supply of Ca2+ in the root zone when Ca2+ absorption by roots or its transportation into inner leaves cannot keep up with the high growth rate (Maruo and Johkan, 2016). Therefore, improving the absorption and transport of Ca2+ may reduce the occurrence of tipburn.
Polyamines (PAs), which mainly include putrescine (Put), cadaverine (Cad), spermidine (Spd), and spermine (Spm), are a class of physiologically active substances that play a wide range of regulatory roles in plants. Their metabolic activities are closely related to the growth and development (Zhao et al., 1999), stress tolerance (Hu et al., 2006; Sun et al., 2016), and disease resistance (Hussain et al., 2011) of higher plants. PAs may affect Ca2+ channels in plants and thus may influence Ca2+ uptake and transportation (Velarde-Buendia, 2012) as well as promote the intracellular concentration of free Ca (Yamaguchi et al., 2007; An et al., 2008), suggesting that PAs play a role in regulating Ca absorption and transportation in plants. Therefore, we hypothesized that PAs may have a positive effect on the prevention of tipburn in vegetables. In this research, we investigated the effect of an exogenous application of Spd on the incidence of tipburn in hydroponically cultivated lettuce. In addition, we explored the possible mechanism of how Spd prevents tipburn in lettuce by investigating the effects of Spd on Ca2+ absorption and transportation. The results obtained in this research provide experimental evidence for the application of exogenous Spd for preventing tipburn.
aterials and Methods
Plant Material and Growth Condition
Seeds of head lettuce (Lactuca sativa L. var. capitata L.) were sown in 72-plug seeding trays filled with sponge blocks and germinated in a nursery greenhouse in Nanjing Agricultural University, China (32°N, 118°50’E). During the cultivation period (2016-2017), the temperature in the greenhouse was maintained at 20 ± 5°C, the relative humidity was 50-70%, and the natural day length was 8-10 h. The seedlings were irrigated twice a day with 1/4-strength Hoagland’s nutrient solution (CaNO3·4H2O, 945 mg·L-1; KNO3, 607 mg·L-1; NH4H2PO4, 115 mg·L-1; MgSO4·7H2O, 493 mg·L-1; H3BO3, 2.86 mg·L-1; MnSO4·4H2O, 2.13 mg·L-1; ZnSO4·7H2O, 0.22 mg·L-1; CuSO4·5H2O, 0.08 mg·L-1; Na2MoO4, 0.02 mg·L-1; NaFeEDTA, 30 mg·L-1). Three weeks after sowing, uniform seedlings with 6-8 true leaves were transplanted onto the cultivation panels floating on the hydroponic containers (dimension: 55 × 40 × 10 cm, 20 L) in a deep flow technique system. Eight plants were planted onto each container filled with 1/2-strength Hoagland’s nutrient solution (EC of 1.8 dS·m-1 and pH of 6.5), and all plants were cultivated for 21 days before the treatments were applied.
Treatments
Twenty-one days after transplanting, two types of Hoagland’s nutrient solution were applied as the main treatments, one that contained 4 mM of Ca2+ (full Ca, sufficient calcium) and one that contained 2 mMof Ca2+ (half Ca, calcium deficient). For the sub treatments, plants were sprayed with 1.0 mM Spd (purity ≥ 98%, Sigma, USA) or distilled water as a control, resulting in four treatments: (1) Full Ca, as the control; (2) Full Ca + Spd; (3) Half Ca; (4) Half Ca + Spd. The Spd (or distilled water) was sprayed onto the lettuce leaves (2 mL/plant) once a day at 6 pm for 3 days. After 3 days of the Spd treatment, all plants were cultivated in their respective nutrient solution and grown for 17 days. To make the content of N in the Ca-deficient nutrient solution consistent with that in the full-Ca nutrient solution, and to avoid experimental error caused by lack of N, 4 mM of potassium nitrate (KNO3) was added to the Ca-deficient nutrient solution. Since potassium ions (K+) have an antagonistic effect on Ca2+ uptake (Flowers and Yeo, 1986), the KNO3 added to the Ca-deficient solution can exacerbate the symptoms of Ca deficiency in plants. The containers were arranged in a 2-way factorial complete block design with three blocks, comprising a total of 12 containers with 96 seedlings in the four treatments (24 seedlings per treatment).
Measurements
The plants were harvested on the 17th day from the start of the treatments (38 days after transplanting). The fresh weight, tipburn index, chlorophyll content, and the maximum quantum yield of photosystem II (PSII) primary photochemistry (Fv/Fm) of lettuce leaves were measured, and the root Ca2+ energy spectrum was determined. The Ca2+ content in leaves and roots were determined at 0, 1, 2, 4, 8, and 16 days after the start of the treatments.
Fresh Weight and Dry Weight Measurements
The fresh weight of the lettuce plants was measured immediately after harvest using an electronic balance (ML204, Mettler-Toledo, Switzerland). To record the dry weight, the plants were put into an oven (DTS-5, Taishun, China) at 105°C for 15 min, then dried at 75°C until constant weight.
Tipburn Index
The tipburn index is used to indicate the severity of tipburn. The occurrence of tipburn is defined as when there are more than 3 brown spots on a leaf edge. The grading standard followed the method described by Shen et al. (2012): Grade 0: no tipburned leaf in the whole plant; Grade 1: less than 1/5 of the plant has tipburned leaves; Grade 2: 1/5 to 2/5 of the plant has tipburned leaves; Grade 3: 2/5 to 3/5 of the plant has tipburned leaves; Grade 4: 3/5 to 4/5 of the plant has tipburned leaves; Grade 5: more than 4/5 of the plant has tipburned leaves.
Tipburn index = Σ (the number of tipburned plants × the grade of tipburn) / (the total number of plants × the highest grade of tipburn) × 100.
Ca2+ Content in Leaves and Roots and its Absorption Rate
Measurement of the Ca2+ content in leaves and roots followed the method described in Sun et al. (2010) with a few modifications. Lettuce leaves (after the main veins were removed) and roots (after being washed lightly with distilled water) were dried in an oven (DTS-5, Taishun, China) at 105°C for 15 mins, then dried at 75°C until constant weight. The dried sample was then ground to a powder and 500 mg of the sample was placed into a digestion tube containing 5 mL of sulfuric acid and hydrogen peroxide and heated to 300°C. The appropriate amount (5 mL) of hydrogen peroxide was repeatedly added to the digestion tube and the tube was heated until the liquid become clear, then the liquid was diluted with water to 50 mL. The Ca2+ concentration was measured with an atomic absorption spectrophotometer (Hp3500G, Hewlett-Packard, Palo Alto, California) at a wavelength of 317.93 nm. Then the Ca2+ content was calculated by the following equation: Ca content (mg·kg-1) = C × n × V/m, where C is the measured concentration (mg·L-1), n is the dilution factor, V is the volume of the solution (mL), and m is the sample dry mass (g).
The absorption rate of Ca2+ by the root from the nutrient solution (Root-Solution) and Ca2+ in the leaves absorbed from the root (Leaf-Root) were analyzed on the 8th day after the start of the treatments using the following equation (Sun et al. 2010):
(R-S) Ca = Ca (R)/Ca (S);
(L-R) Ca = Ca (L)/Ca (R)
where the (R-S) Ca and (L-R) Ca are the absorption rate of Ca2+ by the root from the nutrient solution and Ca2+ in the leaves absorbed from the root, respectively. Ca (R) is the Ca2+ content in the root, Ca (S) is the Ca2+ content in the nutrient solution, and Ca (L) is the Ca2+ content in the leaves.
Ca2+ Content in Different Leaf Layers
Leaves of each plant were divided into six layers from the outer to inner leaves, and each layer included 4 leaves (Zhang et al., 2015). Counting from the oldest leaf, the 1st to 2nd layers of leaves were named as the outer leaves, the 3rd to 4th layers of leaves were the middle leaves, and the 5th to 6th layers of leaves were the inner leaves. Samples were dried in the same way as described above for measuring the Ca2+ content in the leaves of each layer.
Root Ca2+ Energy Spectrum Analysis
The method of Peng et al. (2004) was used for Ca2+ energy spectrum analysis with slight modification. The root of each sample was rinsed 3 times with deionized water, and the harvested root segments, including the tip and 1 cm or more of the root, were dipped in 5% agar, inserted to a depth of 1.0 cm in a copper holder, and immediately sliced free-hand with a razor blade to obtain transverse sections of the elongation zone and root hair zone, and then frozen in liquid nitrogen. The samples were freeze-dried, carbon coated in a high vacuum sputter coater, and stored in a desiccator. Samples were analyzed in a scanning electron microscope (S-3000N, Hitachi, Japan) in conjunction with an energy dispersive spectrometer (Horiba Emax, Horiba, Japan). Counts per second of [Ca2+] were measured in roots from the four different treatments. More than three transverse sections of each treatment were observed and 12 location spots of the same tissue of each section were analyzed. Many of these spectra were transformed to data by the professional software in the computer of S-3000N. Ca2+ content was represented as a percentage in all countable elements in each root transverse section.
Chlorophyll Content
The six leaf layers from the outer to inner leaves of the plant were divided as stated above. For each layer of leaves, 0.1 g fresh leaves were mixed with 5 mL of absolute ethanol:acetone:water (4.5:4.5:1) and placed in the dark for 24 hours. The mixed solution without leaves was used as a control. The optical density (OD) values at 645 nm and 663 nm were measured using a spectrophotometer (UV2800, Unico, China). The chlorophyll content was calculated according to the following formula (Zhou et al., 2016): chlorophyll a content = 12.71 OD663-2.59 OD645, chlorophyll b content = 22.88 OD645-4.67 OD663, total chlorophyll content = chlorophyll a content + chlorophyll b content.
Fv/Fm Measurement
One to two fully expended leaves from each layer were selected for determining a chlorophyll fluorescence parameter, Fv/Fm, using a PAM 2100 modulation fluorometer (PAM 2000, Heinz Walz GmbH, Effeltrich, Germany). Minimum fluorescence (Fo) was determined by illuminating the leaf with a dim red light (< 0.1 µmol·m-2·s-1) modulated at 0.6 kHz after keeping the leaf in darkness for 30 min. Maximum fluorescence of the dark-adapted leaf (Fm) was obtained during a subsequent saturating light pulse (8,000 µmol·m-2·s-1 for 0.8 s). Fv/Fm was calculated as (Fm–Fo)/Fm.
Statistical Analysis
All experiments were conducted using three biological replicates. Three plants from each replicate were selected for determination of plant growth, tipburn index, chlorophyll content, Ca2+ content, chlorophyll fluorescence, and the Ca2+ energy spectrum of roots. All data were statistically analyzed in SAS (SAS institute, Cary, NC, USA) using Duncan’s multiple range test at the p < 0.05 level of significance.
Results
Plant Growth and Tipburn Index
The plants grown under the Ca-deficient condition had lower fresh weight and dry weight and a higher tipburn index than those of the control (Table 1). The Spd treatment significantly increased the fresh weight and dry weight, and decreased the tipburn index in the Ca-deficient plants. There was no significant difference in growth parameters and tipburn index between the plants with and without Spd treatment under the sufficient-Ca condition (Table 1). The results indicate that Ca deficiency significantly inhibits plant growth, leading to severe tipburn incidence in leaves, while spraying the plants with Spd relieved the inhibition in growth and the level of tipburn caused by Ca deficiency.
Ca2+ Content in Leaves and Roots and the Ca2+ Absorption Rate
Under the Ca-deficient condition, the contents of Ca2+ in leaves and roots at 0, 1, 2, 4, 8, and 16 days after the start of the treatments were significantly lower than those of the control, while the Spd treatment significantly increased the content of Ca2+ in the leaves (Fig. 1A) and roots (Fig. 1B).
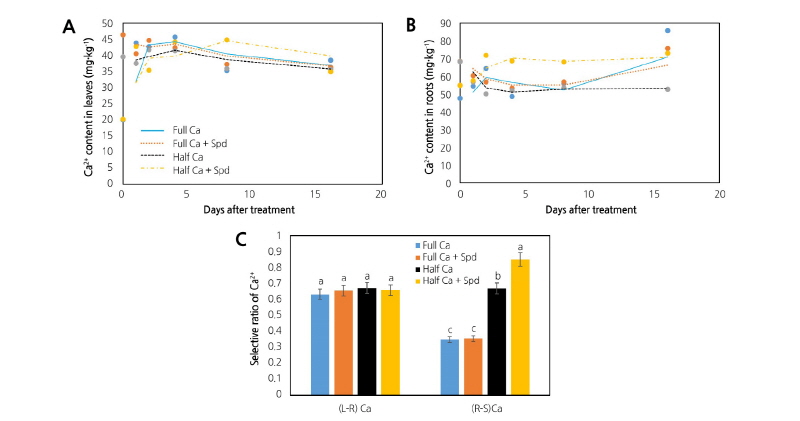
Fig. 1. Effects of an exogenous application of spermidine (Spd) on the Ca2+ content in leaves (A) and roots (B), and the selective ratio of Ca2+ at 8 days after the start of the treatments (C) of lettuce grown in Ca-sufficient and Ca-deficient conditions. Ca2+ content data were obtained at 0, 1, 2, 4, 8, and 16 days after the start of the treatments, and data of the selective ratio of Ca2+ were obtained at 8 days after the start of the treatments. Data are shown as the mean ± SE (n = 3). Different letters indicate significant differences at p < 0.05 according to Duncan’s multiple range test. Full Ca: control, plants cultivated in a Ca2+ concentration of 4 mM Hoagland’s solution; Full Ca + Spd: plants cultivated in the control solution with a foliar application of 0.1 mM Spd for 3 days; Half Ca: plants cultivated in a Ca2+ concentration of 2 mM Hoagland’s solution; Half Ca + Spd: plants cultivated in a Ca2+ concentration of 2 mM Hoagland’s solution with a foliar application of 0.1 mM Spd for 3 days. The (R-S) Ca and (L-R) Ca are the absorption rate of Ca2+ by the roots from the nutrient solution and Ca2+ in the leaves absorbed from the root, respectively.
The (R-S) Ca and (L-R) Ca of the plants at 8 days after starting the treatments are shown in Fig. 1C. Foliar spraying of Spd had no significant effect on (L-R) Ca under the control or Ca-deficient conditions, but significantly increased (R-S) Ca under the Ca-deficient condition, indicating that exogenous Spd promoted Ca2+ absorption by the root from the nutrient solution, thereby increasing the Ca2+ content in the plant.
Ca2+ Energy Spectrum Distribution in the Root Meristematic Zone and the Root Hair Zone
Supplemental Fig. 1 shows a typical image of the energy spectrum analysis. We quantitatively analyzed Ca2+ distribution in the root elongation zone and root hair zone of lettuce and the results are shown in Fig. 2. Ca deficiency decreased the Ca2+ content in the root elongation and root hair zones compared to that of the control, while the Spd treatment increased the Ca2+ content in the root elongation and root hair zones of the plants grown in the Ca-deficient condition. In the Ca-sufficient condition, the Spd treatment did not significantly affect the distribution of Ca2 + in the roots of lettuce. These results further suggested that exogenous Spd promoted the absorption of Ca2+ by the roots from the nutrient solution under the Ca-deficient condition.
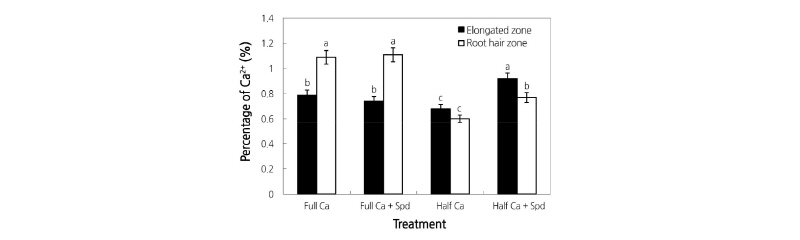
Fig. 2. Effects of an exogenous application of spermidine (Spd) on the percentage of Ca2+ in the root elongation zone and the root hair zone of lettuce grown in Ca-sufficient and Ca-deficient conditions. Data were obtained at 17 days after the start of the treatments and represent the mean ± SE (n = 3) and were transformed from the spectra of Supplemental Fig. 1 by the professional software in the computer of S-3000N. Ca2+ content is represented as a percentage in all countable elements in each root transverse section. Different letters in the same color column indicate significant differences at p < 0.05 according to Duncan’s multiple range test. Full Ca: control, plants cultivated in a Ca2+ concentration of 4 mmol·L-1 Hoagland’s solution; Full Ca + Spd: plants cultivated in the control solution with a foliar application of 0.1 mM Spd for 3 days; Half Ca: plants cultivated in a Ca2+ concentration of 2 mM Hoagland’s solution; Half Ca + Spd: plants cultivated in a Ca2+ concentration of 2 mM Hoagland’s solution with a foliar application of 0.1 mM Spd for 3 days.
Ca2+ Content in Different Leaf Layers
Under the control condition, the Ca2+ content in the different leaf layers showed a decreasing trend from the outside to inside layers (Fig. 3). The Spd treatment significantly increased the Ca2+ content in each layer of lettuce leaves. Under the Ca-deficient condition, the Ca2+ content of the 3rd to 6th leaves (middle part and inner part) was significantly lower than that of the control, whereas the Spd treatment significantly increased the Ca2+ content in the middle and inner leaves.
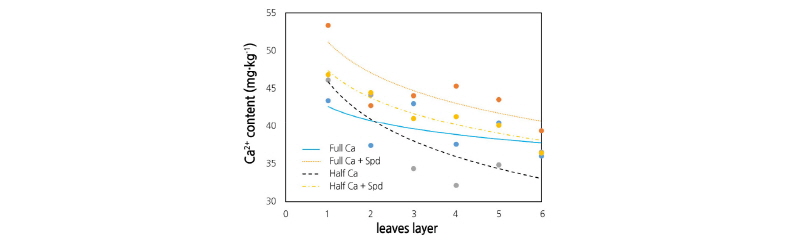
Fig. 3. Effects of an exogenous application of spermidine (Spd) on the Ca2+ content in different layers of leaves of lettuce grown in Ca-sufficient and Ca-deficient conditions. Plant leaves were divided into six layers, each layer included 4 leaves. Data were obtained at 17 days after the start of the treatments and represent the mean ± SE (n = 3). Different letters indicate significant differences at p < 0.05 according to Duncan’s multiple range test. Full Ca: control, plants cultivated in a Ca2+ concentration of 4 mM Hoagland’s solution; Full Ca + Spd: plants cultivated in the control solution with a foliar application of 0.1 mM Spd for 3 days; Half Ca: plants cultivated in a Ca2+ concentration of 2 mM Hoagland’s solution; Half Ca + Spd: plants cultivated in a Ca2+ concentration of 2 mM Hoagland’s solution with a foliar application of 0.1 mM Spd for 3 days.
Chlorophyll Content and Fv/Fm
As shown in Fig. 4, the chlorophyll content and Fv/Fm of lettuce leaves increased gradually from the 1st- to 4th layers, and then decreased from the 4th- to 6th layers of leaves in the control plants. Under the Ca-sufficient condition, the Spd treatment increased the chlorophyll contend and Fv/Fm of the leaves in the 2nd to 6th layers. The Ca-deficiency treatment significantly decreased the chlorophyll content of the leaves in the 1st to 4th layers and the Fv/Fm of leaves in the 2nd to 6th layers, but the Spd treatment increased the chlorophyll content and Fv/Fm of the middle and inner leaves in these plants.
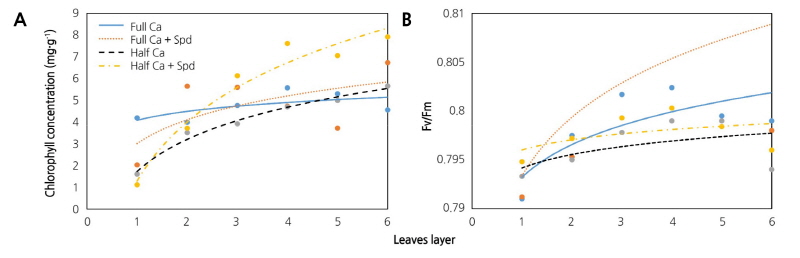
Fig. 4. Effects of an exogenous application of spermidine (Spd) on the chlorophyll content (A) and Fv/Fm (B) of different layers of leaves of lettuce grown in Ca-sufficient and Ca-deficient conditions. Plant leaves were divided into six layers, each layer included 4 leaves. Data were obtained at 17 days after the start of the treatments and represent the mean ± SE (n = 3). Full Ca: control, plants cultivated in a Ca2+ concentration of 4 mM Hoagland’s solution; Full Ca + Spd: plants cultivated in the control solution with a foliar application of 0.1 mM Spd for 3 days; Half Ca: plants cultivated in a Ca2+ concentration of 2 mM Hoagland’s solution; Half Ca + Spd: plants cultivated in a Ca2+ concentration of 2 mM Hoagland’s solution with a foliar application of 0.1 mM Spd for 3 days.
Discussion
There are many physiological diseases associated with Ca deficiency in vegetable production, such as blossom end rot of tomatoes (Solanum lycopersicum), tipburn of leafy vegetables, and fruit cracking (Marty, 1999). Natti and Atkin (1962) explained that tipburn in cabbage (Brassica oleracea L.) and baby cabbage (Brassica rapa pekinensis) is a kind of physiological disease caused by Ca deficiency and Ca metabolic disorders in plants. Barta and Tibbitts (2000) reported that Ca deficiency was the main cause of tipburn in head lettuce. In the present study, our results showed that the Ca deficiency treatment indeed lead to severe tipburn (a high tipburn index) and significantly inhibited lettuce growth. An exogenous application of Spd significantly relieved the effects caused by Ca deficiency (Table 1).
Walker and Edgington (1957) found that only a small amount of Ca2+ was detected in the inner leaves of cabbage even though a large amount of Ca2+ was absorbed by the roots, indicating that the tipburn observed on inner leaves is due to a certain environment that limits Ca2+ movement to the inner leaves from the roots. As Ca is a relatively immobile element in plant leaves, Ca2+ cannot be translocated from the older leaves to the younger leaves when Ca deficiency occurs, resulting in Ca deficiency of the young leaves and tipburn (Aloni, 1986). The Ca2+ contents in lettuce leaves and roots grown under the Ca-deficient condition were lower than those of the control; however, after exogenous Spd was applied, more Ca was transported into the plants (Fig. 1A and B). Furthermore, exogenous Spd significantly increased the Ca2+ uptake rate of the roots from the nutrient solution under the Ca-deficient condition (Fig. 1C), increased the Ca2+ content in the root elongation zone and the root hair zone (Fig. 2 and Supplemental Fig. 1), and increased the Ca2+ content in the middle and inner leaves (which are relatively young leaves) (Fig. 3), resulting in a reduced incidence of tipburn. Ca supplementation seems to diminish the action of Spd. This result indicates that Spd may be involved in the initial ionic attachment mechanism only under Ca-deficient conditions.
A decrease in chlorophyll content and Fv/Fm is typically associated with leaf senescence (Thimann and Satler, 1979; Wingler et al., 2004). Under Ca deficiency, the chlorophyll content and Fv/Fm of lettuce leaves decreased in the present study (Fig. 4), indicating that Ca deficiency accelerated leaf senescence of lettuce. The typical symptom of tipburn in cabbage is that the edges of the leaves gradually begin to turn yellow, become dry, and finally turn to brown or black (Ji et al., 2005). Senescence is also characterized as a gradual loss of the green color caused by the disappearance of chlorophyll (Zhou et al., 2016), indicating that there may be a close relationship between tipburn and leaf senescence. Ca deficiency accelerated lettuce leaf senescence (a decrease in chlorophyll content, Fig. 4) and led to tipburn (Table 1), suggesting that Ca deficiency may first cause leaf senescence and then induce tipburn. Spd treatments delayed leaf senescence in barley (Hordeum vulgare) (Legocka and Zajchert, 1999) and cucumber (Hordeum vulgare) (Zhou et al., 2016). In our research, the Spd treatment significantly increased the Ca2+ content in leaves (Fig. 3) and enhanced the chlorophyll content (Fig. 4A) and Fv/Fm (Fig. 4B) of lettuce plants grown under the Ca-deficient condition, indicating that Spd delayed leaf senescence and decreased the incidence of tipburn by promoting Ca2+ absorption. This result also indicates that Spd may work as an anti-senescence agent, though the Ca-sufficient condition diminished the function of Spd.
Physiological diseases related to Ca deficiency are widespread and cause great losses in vegetable production. This study found that an exogenous application of Spd could prevent tipburn caused by Ca deficiency in lettuce plants and explored its mechanism of action. This finding provides a new approach for the prevention of such diseases. The next step is to further explore the molecular mechanism of Spd in preventing tipburn.
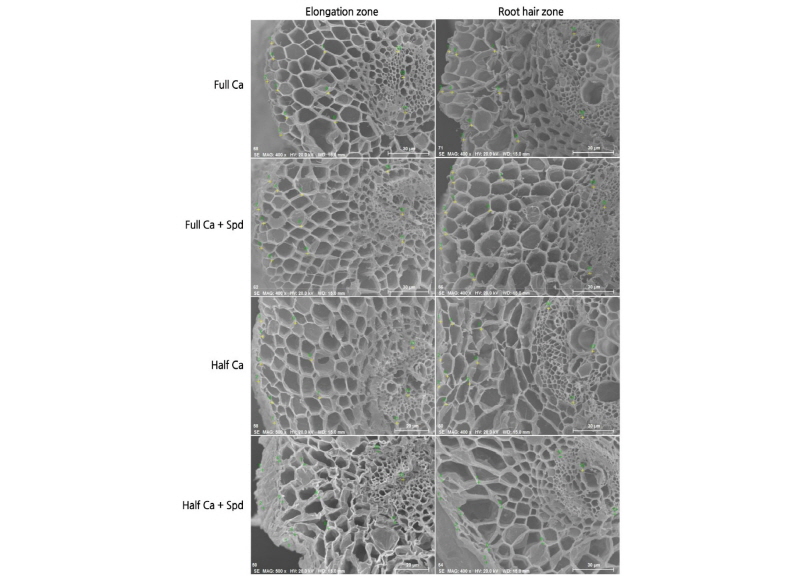
Supplemental Fig. 1. Images of the Ca2+ distribution in the root elongation zone and the root hair zone of lettuce grown in Ca-sufficient and Ca-deficient conditions from map-scanning of an X-ray. Images are the scanned photo of the root elongation zone and root hair zone by a scanning electron microscope and an energy spectrometer at 17 days after the start of the treatments. Full Ca: control, plants cultivated in a Ca2+ concentration of 4 mM Hoagland’s solution; Full Ca + Spd: plants cultivated in the control solution with a foliar application of 0.1 mM Spd for 3 days; Half Ca: plants cultivated in a Ca2+ concentration of 2 mM Hoagland’s solution; Half Ca + Spd: plants cultivated in a Ca2+ concentration of 2 mM Hoagland’s solution with a foliar application of 0.1 mM Spd for 3 days.